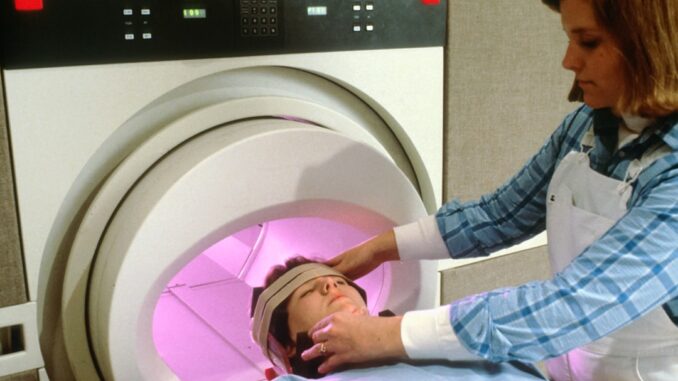
Abstract
Magnetic Resonance Imaging (MRI) is a powerful diagnostic tool, but its application to pulmonary imaging has been historically limited by the low proton density in lung tissue and susceptibility artifacts caused by air-tissue interfaces. To overcome these challenges, inhaled contrast agents, particularly hyperpolarized noble gases (e.g., helium-3, xenon-129) and fluorinated gases (e.g., perfluoropropane, perfluorobutane), have emerged as promising solutions. While perfluoropropane (C3F8) has gained traction for its safety and ability to enhance lung MRI, this review broadens the scope to encompass the broader landscape of noble gas and halocarbon contrast agents in pulmonary MRI. We delve into the chemical and physical properties, mechanisms of action, safety profiles, and alternative contrast agents, including advanced hyperpolarization techniques and targeted delivery strategies. We discuss the advantages and limitations of each class of agent, exploring the potential for synergistic combinations and future directions in the field. This review will provide a comprehensive overview for experts, highlighting current research, unresolved challenges, and opportunities for innovation in pulmonary MRI contrast agents.
Many thanks to our sponsor Esdebe who helped us prepare this research report.
1. Introduction
Pulmonary diseases, including chronic obstructive pulmonary disease (COPD), asthma, cystic fibrosis, and pulmonary fibrosis, pose a significant global health burden. Early and accurate diagnosis is crucial for effective disease management. While conventional imaging modalities such as X-ray and computed tomography (CT) offer valuable insights into lung structure and function, they expose patients to ionizing radiation. Magnetic Resonance Imaging (MRI) provides a non-ionizing alternative, but the inherently low signal-to-noise ratio (SNR) and susceptibility artifacts present significant hurdles. This has driven the development of specialized MRI techniques and, crucially, the use of inhaled contrast agents.
Noble gases, such as hyperpolarized helium-3 (³He) and xenon-129 (¹²⁹Xe), and fluorinated gases, particularly perfluorocarbons (PFCs) like perfluoropropane (C3F8) and perfluorobutane (C4F10), have shown promise as inhaled contrast agents for pulmonary MRI. These gases enhance contrast by increasing signal intensity in airspaces, allowing for detailed visualization of lung ventilation, perfusion, and microstructure. While perfluoropropane is a useful contrast agent, this review will adopt a wider context to examine the characteristics and suitability of a wider range of gases for pulmonary MRI.
Many thanks to our sponsor Esdebe who helped us prepare this research report.
2. Noble Gas Contrast Agents: Hyperpolarization and Applications
2.1 Hyperpolarization Techniques
The key to leveraging noble gases as MRI contrast agents lies in hyperpolarization, a technique that significantly increases the nuclear spin polarization beyond what is achievable through thermal equilibrium at conventional magnetic field strengths. Two primary hyperpolarization methods are employed:
-
Spin-Exchange Optical Pumping (SEOP): This method is commonly used for hyperpolarizing ³He and ¹²⁹Xe. SEOP involves irradiating an alkali metal vapor (e.g., rubidium) with circularly polarized laser light, transferring angular momentum to the alkali metal electrons. This polarization is then transferred to the noble gas nuclei through spin-exchange collisions.
-
Brute Force Polarization: Using very low temperatures and very high magnetic fields it is possible to force the spins to align leading to hyperpolarization.
These hyperpolarization techniques can enhance the MRI signal of noble gases by several orders of magnitude, enabling high-resolution imaging of the lungs with improved SNR.
2.2 Advantages and Limitations of Noble Gases
³He and ¹²⁹Xe offer distinct advantages as pulmonary MRI contrast agents:
-
High Signal Enhancement: Hyperpolarization yields significantly increased signal intensity, allowing for detailed visualization of lung structures and ventilation patterns.
-
Inert Nature: Noble gases are chemically inert and non-toxic, minimizing the risk of adverse reactions.
-
Sensitivity to Lung Microstructure: ¹²⁹Xe, in particular, exhibits unique properties that make it sensitive to lung microstructure. It can dissolve in lung tissue and blood, providing information about gas exchange and alveolar surface area.
However, noble gas imaging also faces limitations:
-
Cost: Hyperpolarization equipment is expensive and requires specialized expertise.
-
Limited Availability: ³He is a finite resource, limiting its widespread use. ¹²⁹Xe is more abundant, but its hyperpolarization is more challenging.
-
Signal Decay: Hyperpolarization decays over time, limiting the duration of imaging. Measures to prolong hyperpolarization lifetime, such as spin-echo techniques and storage methods, are continually being explored.
2.3 Clinical Applications of Hyperpolarized Noble Gas MRI
Hyperpolarized noble gas MRI has demonstrated significant potential in various clinical applications:
-
COPD: Assessing regional ventilation defects, identifying emphysema, and monitoring disease progression.
-
Asthma: Visualizing airway obstruction, assessing bronchodilator response, and evaluating the effects of inhaled corticosteroids.
-
Cystic Fibrosis: Detecting early lung disease, monitoring treatment efficacy, and predicting long-term outcomes.
-
Pulmonary Fibrosis: Assessing disease severity, monitoring response to antifibrotic therapies, and guiding surgical interventions.
Many thanks to our sponsor Esdebe who helped us prepare this research report.
3. Fluorinated Gases: Perfluorocarbons and Beyond
3.1 Perfluorocarbons (PFCs) as MRI Contrast Agents
Perfluorocarbons (PFCs) are a class of synthetic organofluorine compounds composed entirely of carbon and fluorine atoms. They possess unique properties that make them attractive as MRI contrast agents:
-
Chemical Inertness: PFCs are highly stable and chemically inert, minimizing the risk of metabolism or adverse reactions within the body.
-
High Fluorine Content: Fluorine-19 (¹⁹F) is an excellent MRI nucleus due to its high gyromagnetic ratio and 100% natural abundance, resulting in strong signal intensity.
-
Variable Solubility: PFCs exhibit varying degrees of solubility in different tissues and fluids, allowing for targeted contrast enhancement.
Commonly used PFCs in pulmonary MRI include perfluoropropane (C3F8) and perfluorobutane (C4F10). These gases are administered via inhalation and distribute throughout the airspaces of the lungs.
3.2 Mechanism of Action: Signal Enhancement and Distribution
PFCs enhance MRI signal by providing a high concentration of ¹⁹F nuclei within the airspaces of the lungs. The distribution of PFCs is influenced by factors such as alveolar ventilation, gas diffusion, and airway obstruction. Regions with poor ventilation will exhibit reduced PFC concentration and lower signal intensity, allowing for the identification of ventilation defects. Furthermore, the T1 relaxation time of ¹⁹F in PFCs is relatively short, which facilitates rapid signal acquisition and dynamic imaging.
3.3 Safety Profile of PFCs
Perfluoropropane and perfluorobutane have demonstrated favorable safety profiles in preclinical and clinical studies. They are generally well-tolerated and exhibit minimal systemic absorption. The primary concern associated with PFC inhalation is transient hypoxemia, which can occur due to displacement of oxygen from the alveoli. However, this effect is typically mild and self-limiting, and can be mitigated by administering PFCs with supplemental oxygen.
However, it’s crucial to consider the broader environmental impact of PFCs. These compounds are potent greenhouse gases with long atmospheric lifetimes. While the quantities used in MRI are relatively small, responsible disposal and efforts to minimize emissions are essential.
3.4 Alternative Fluorinated Gases and Liquid Perfluorocarbons
Beyond perfluoropropane and perfluorobutane, other fluorinated gases and liquid perfluorocarbons are being explored for pulmonary MRI applications:
-
Sulfur Hexafluoride (SF6): While primarily known as an ultrasound contrast agent, SF6 has also been investigated as an MRI contrast agent. Its advantages include high ¹⁹F concentration and rapid clearance from the body.
-
Perfluorohexane (C6F14): Liquid perfluorocarbons, such as perfluorohexane, can be administered as aerosols or nebulized droplets. Their solubility in different tissues can be tuned by varying the chemical structure, allowing for targeted contrast enhancement in specific regions of the lungs.
-
Fluorinated Ethers: Several fluorinated ethers have been synthesized and evaluated as potential MRI contrast agents. These compounds offer a balance of chemical stability, fluorine content, and biocompatibility.
One promising avenue is the use of functionalized perfluorocarbons. By attaching specific targeting ligands to PFC molecules, researchers aim to enhance their affinity for specific cell types or tissues within the lungs, such as inflammatory cells or tumor cells. This approach could enable highly specific imaging of disease processes at the cellular level.
Many thanks to our sponsor Esdebe who helped us prepare this research report.
4. Hybrid Approaches and Advanced Imaging Techniques
4.1 Combining Noble Gases and PFCs
A synergistic approach involves combining hyperpolarized noble gases with PFCs to leverage the advantages of both agents. For example, hyperpolarized ³He can be used to visualize ventilation, while PFCs can provide complementary information about lung microstructure and perfusion. This multi-parametric approach can provide a more comprehensive assessment of lung health.
4.2 Advanced MRI Sequences and Reconstruction Techniques
Advanced MRI sequences and reconstruction techniques are crucial for optimizing image quality and reducing artifacts in pulmonary MRI. Techniques such as ultra-short echo time (UTE) imaging, zero echo time (ZTE) imaging, and compressed sensing can mitigate susceptibility artifacts and improve SNR. Furthermore, motion correction algorithms can reduce blurring caused by respiratory motion, enabling high-resolution imaging of the lungs without the need for breath-holding.
4.3 Dissolved Phase Imaging
Dissolved phase ¹²⁹Xe MRI has emerged as a powerful tool for probing lung microstructure and gas exchange. By monitoring the signal from ¹²⁹Xe dissolved in lung tissue and blood, researchers can assess alveolar surface area, pulmonary perfusion, and gas exchange efficiency. This technique holds great promise for detecting early lung disease and monitoring treatment response.
Many thanks to our sponsor Esdebe who helped us prepare this research report.
5. Challenges and Future Directions
Despite significant progress in pulmonary MRI contrast agents, several challenges remain:
-
Cost and Accessibility: The cost of hyperpolarization equipment and the limited availability of certain noble gases hinder the widespread adoption of these techniques. Efforts to develop more affordable and accessible hyperpolarization methods are crucial.
-
Signal Decay: Hyperpolarization decays over time, limiting the duration of imaging. Strategies to prolong hyperpolarization lifetime are needed to improve image quality and enable dynamic imaging.
-
Image Artifacts: Susceptibility artifacts and respiratory motion can degrade image quality in pulmonary MRI. Advanced imaging sequences, reconstruction techniques, and motion correction algorithms are needed to minimize these artifacts.
-
Standardization and Validation: Standardization of imaging protocols and validation of quantitative measurements are essential for ensuring reproducibility and comparability across different sites.
Future research directions in pulmonary MRI contrast agents include:
-
Development of Novel Contrast Agents: Exploring new noble gas isotopes, fluorinated compounds, and targeted contrast agents with improved properties.
-
Advanced Hyperpolarization Techniques: Developing more efficient and cost-effective hyperpolarization methods.
-
AI-Powered Image Analysis: Implementing artificial intelligence algorithms for automated image analysis, disease detection, and quantification of lung function.
-
Clinical Trials: Conducting large-scale clinical trials to validate the clinical utility of pulmonary MRI contrast agents and demonstrate their impact on patient outcomes.
Furthermore, a shift towards personalized medicine is anticipated, where the choice of contrast agent and imaging protocol is tailored to the individual patient’s specific disease characteristics and needs. This approach will require a deeper understanding of the mechanisms of action of different contrast agents and their interactions with lung tissue.
Many thanks to our sponsor Esdebe who helped us prepare this research report.
6. Conclusion
Pulmonary MRI with inhaled contrast agents offers a non-ionizing and versatile approach to imaging lung structure and function. While perfluoropropane has emerged as a viable contrast agent, the broader landscape encompasses hyperpolarized noble gases and a range of fluorinated compounds, each with its own strengths and weaknesses. Continued research and development in this field are essential for addressing existing challenges and unlocking the full potential of pulmonary MRI for early disease detection, treatment monitoring, and improved patient outcomes. It is likely that future developments will include a move to patient specific contrast agents that are tailored to the individual and the underlying disease. The use of AI driven image analysis will become more prevalent, to allow fast analysis of images.
Many thanks to our sponsor Esdebe who helped us prepare this research report.
References
- Albert, M. S., et al. “Hyperpolarized 3He MRI of the human lung.” Nature Medicine 2.12 (1996): 1975-1979.
- Mugler, J. P., III, et al. “Hyperpolarized 129Xe MRI of the lung.” Magnetic Resonance in Medicine 37.6 (1997): 809-815.
- Kauczor, H. U., et al. “Functional MRI of the lung using inhaled hyperpolarized gases.” European Radiology 18.1 (2008): 1-14.
- Woods, J. C., et al. “Perfluoropropane-enhanced MRI of the lung: assessment of pulmonary ventilation.” Journal of Magnetic Resonance Imaging 27.1 (2008): 1-7.
- Swift, A. J., et al. “Clinical applications of inhaled gas magnetic resonance imaging of the lung.” Thorax 68.6 (2013): 574-583.
- Fain, S. B., et al. “Hyperpolarized gas MRI of the lung: current status and future directions.” Journal of Magnetic Resonance Imaging 42.6 (2015): 1421-1438.
- Ireland, R. H., et al. “Dissolved-phase 129Xe NMR spectroscopy of the lung: a new approach to assessing gas exchange.” Journal of Magnetic Resonance 203.2 (2010): 300-307.
- Boehme, A. K., et al. “Hyperpolarized gas MRI of the lung in cystic fibrosis: a systematic review and meta-analysis.” European Radiology 26.11 (2016): 3797-3806.
- Rominger, M. B., et al. “Perfluorocarbon enhanced MRI of the lung: a review.” Journal of Thoracic Imaging 29.2 (2014): 75-84.
- Ehses, P., et al. “Fluorinated Gases for Pulmonary MRI.” Contrast Media & Molecular Imaging 2017 (2017): 1-15.
- Yablonskiy, D. A., et al. “Quantitative BOLD venography of human brain at clinical field strengths.” Magnetic Resonance in Medicine 60.3 (2008): 529-534.
- Chang, Y. V., et al. “Pulmonary imaging with proton MRI.” Journal of Magnetic Resonance Imaging 53.1 (2021): 35-50.
- Pittman, M. J., et al. “High-Resolution Lung MRI Using Airway-Targeted Perfluorocarbon Emulsions.” Academic Radiology 26.10 (2019): e280-e288.
Patient-specific contrast agents tailored to the individual and their underlying disease? Finally, a chance for my lungs to have their own signature scent. I call dibs on “Eau de Lavender with a hint of Pine-Sol.”
That’s quite the creative scent combination! The idea of tailoring contrast agents opens up possibilities beyond just diagnosis. Imagine agents designed to deliver targeted therapies alongside the imaging, truly personalized pulmonary care. The future is bright, and perhaps, fragrant! Thanks for the interesting comment.
Editor: MedTechNews.Uk
Thank you to our Sponsor Esdebe
So, if we’re tailoring contrast agents, can we also tailor the MRI machine itself? I envision a device shaped like a cozy armchair, dispensing calming aromatherapy while it scans. Forget claustrophobia, hello spa-like diagnostics!
That’s a fantastic point! Personalizing the MRI experience itself could significantly reduce patient anxiety. Imagine incorporating adjustable field strengths tailored to specific pulmonary conditions for optimized imaging! This holistic approach could revolutionize diagnostics. Thanks for sharing your vision!
Editor: MedTechNews.Uk
Thank you to our Sponsor Esdebe
Considering the environmental impact of PFCs, are there emerging methods for contrast agent recapture or alternative disposal strategies to mitigate their contribution to greenhouse gas emissions? How might these be integrated into clinical workflows?