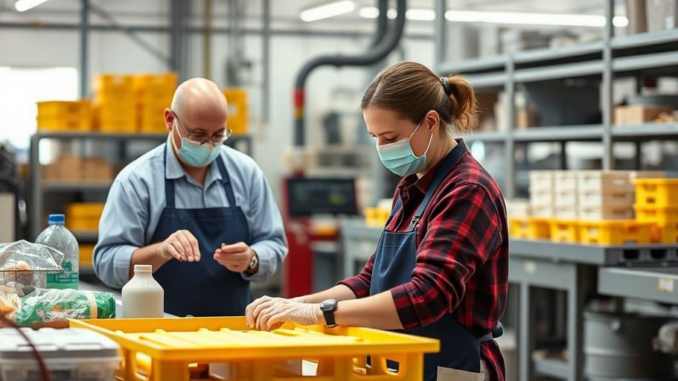
Abstract
Cell and gene therapies (CGTs) represent a paradigm shift in modern medicine, offering the potential to treat and even cure previously intractable diseases. This report provides a comprehensive overview of the current state of CGTs, encompassing technological advancements, clinical applications across diverse therapeutic areas, manufacturing challenges, regulatory pathways, and the ethical considerations that accompany these innovative approaches. The report explores the underlying scientific principles, diverse delivery methods, and the evolution from early successes to more sophisticated and targeted therapies. Furthermore, it examines the challenges related to scalability, cost, and accessibility, highlighting the need for novel manufacturing strategies and equitable access models. The ethical implications of CGTs, including germline editing, off-target effects, and the potential for enhancement, are also critically discussed. Finally, the report outlines future directions and potential breakthroughs, emphasizing the need for continued research, collaboration, and responsible innovation to realize the full potential of CGTs.
Many thanks to our sponsor Esdebe who helped us prepare this research report.
1. Introduction
Cell and gene therapies (CGTs) have emerged as transformative therapeutic modalities, offering the prospect of addressing the root cause of diseases rather than merely managing symptoms. These therapies hold immense promise for a wide range of conditions, including inherited genetic disorders, cancers, infectious diseases, and autoimmune disorders. The field has witnessed remarkable progress in recent years, driven by advancements in gene editing technologies, viral vector engineering, cell culture techniques, and our understanding of disease mechanisms. However, the development and implementation of CGTs are not without significant challenges. This report aims to provide a comprehensive overview of the current state of CGTs, encompassing technological innovations, clinical applications, manufacturing hurdles, regulatory landscape, and the ethical considerations that shape the future of this rapidly evolving field.
The foundational principle of CGTs involves manipulating a patient’s cells or genes to achieve a therapeutic outcome. Cell therapies involve the administration of living cells, either autologous (derived from the patient) or allogeneic (derived from a donor), to replace or repair damaged tissues or to enhance immune function. Gene therapies, on the other hand, introduce genetic material into cells to correct defective genes, silence overexpressed genes, or deliver therapeutic genes. Both approaches leverage the body’s own biological machinery to achieve a lasting therapeutic effect.
Many thanks to our sponsor Esdebe who helped us prepare this research report.
2. Technological Advancements in Cell and Gene Therapy
The rapid progress in CGTs is underpinned by a series of groundbreaking technological advancements that have enhanced the precision, efficiency, and safety of these therapies.
2.1 Gene Editing Technologies
Gene editing technologies, such as CRISPR-Cas9, TALENs, and zinc finger nucleases (ZFNs), have revolutionized the field by enabling precise and targeted modification of the genome. These technologies allow researchers to correct disease-causing mutations, insert therapeutic genes at specific genomic locations, and disrupt the expression of unwanted genes. CRISPR-Cas9, in particular, has gained widespread adoption due to its simplicity, versatility, and efficiency. However, challenges remain in minimizing off-target effects and ensuring efficient delivery of the editing machinery to the target cells.
The evolution of gene editing extends beyond simply cutting and disrupting genes. Base editing and prime editing are newer approaches that allow for more precise DNA modifications without requiring double-strand breaks. Base editing allows for the direct conversion of one base pair to another (e.g., C to T or A to G) with high precision, while prime editing can insert, delete, or replace DNA sequences at targeted locations. These advanced editing tools hold promise for treating a wider range of genetic diseases with fewer off-target effects.
2.2 Viral Vector Engineering
Viral vectors are commonly used to deliver therapeutic genes into cells. Adeno-associated viruses (AAVs), lentiviruses, and adenoviruses are the most widely used viral vectors in gene therapy. Researchers have made significant strides in engineering these vectors to improve their tropism (targeting specificity), transduction efficiency (ability to deliver genes into cells), and safety profile. For instance, AAV serotypes have been engineered to target specific tissues and cell types, reducing the risk of off-target effects. Furthermore, self-inactivating (SIN) lentiviral vectors have been developed to minimize the risk of insertional mutagenesis (disruption of endogenous genes).
2.3 Cell Culture and Manufacturing Technologies
The successful development of CGTs relies heavily on efficient and scalable cell culture and manufacturing processes. Advances in bioreactor technology, cell separation techniques, and cryopreservation methods have enabled the large-scale production of therapeutic cells and viral vectors. Closed-system manufacturing platforms are increasingly being adopted to minimize the risk of contamination and ensure product quality. Automation and process optimization are also crucial for reducing manufacturing costs and increasing throughput.
2.4 Delivery Methods
The efficacy of CGTs depends on the efficient delivery of therapeutic genes or cells to the target tissue or organ. Delivery methods can be broadly classified into two categories: in vivo and ex vivo. In vivo delivery involves direct administration of the therapeutic agent into the patient’s body, while ex vivo delivery involves modifying cells outside the body and then transplanting them back into the patient. Each approach has its own advantages and disadvantages. In vivo delivery is less complex and less costly but may be less efficient and more prone to off-target effects. Ex vivo delivery allows for greater control over the modification process but is more complex and costly. Emerging delivery technologies, such as lipid nanoparticles (LNPs) and exosomes, are showing promise for targeted delivery of genetic payloads.
Many thanks to our sponsor Esdebe who helped us prepare this research report.
3. Clinical Applications of Cell and Gene Therapy
CGTs have demonstrated remarkable clinical success in a variety of therapeutic areas. Several CGTs have been approved by regulatory agencies for the treatment of genetic diseases, cancers, and other conditions.
3.1 Genetic Diseases
CGTs have shown particular promise in treating inherited genetic disorders caused by single-gene mutations. Examples of successful gene therapies for genetic diseases include:
- Spinal Muscular Atrophy (SMA): Zolgensma, an AAV-based gene therapy, has been approved for the treatment of SMA, a neuromuscular disorder caused by mutations in the SMN1 gene. Zolgensma delivers a functional copy of the SMN1 gene to motor neurons, improving muscle function and survival.
- Beta-Thalassemia: Beti-cel, a lentiviral-based gene therapy, has been approved for the treatment of beta-thalassemia, a blood disorder caused by mutations in the HBB gene. Beti-cel involves transplanting autologous hematopoietic stem cells (HSCs) that have been genetically modified to express a functional beta-globin gene.
- Severe Combined Immunodeficiency (SCID): Gene therapies have been developed for various forms of SCID, including adenosine deaminase (ADA)-SCID and X-linked SCID. These therapies involve transplanting autologous HSCs that have been genetically modified to express the deficient enzyme or protein.
3.2 Cancer
CGTs have revolutionized the treatment of certain cancers, particularly hematological malignancies. Chimeric antigen receptor (CAR) T-cell therapy has emerged as a highly effective treatment for relapsed or refractory B-cell lymphomas and acute lymphoblastic leukemia (ALL). CAR T-cell therapy involves engineering a patient’s T cells to express a CAR that recognizes and binds to a specific antigen on cancer cells, leading to their destruction. Several CAR T-cell therapies have been approved by regulatory agencies, including:
- Tisagenlecleucel (Kymriah): Approved for the treatment of relapsed or refractory B-cell ALL in pediatric and young adult patients.
- Axicabtagene ciloleucel (Yescarta): Approved for the treatment of relapsed or refractory large B-cell lymphoma.
- Lisocabtagene maraleucel (Breyanzi): Approved for the treatment of relapsed or refractory large B-cell lymphoma.
3.3 Other Therapeutic Areas
CGTs are being explored for the treatment of a wide range of other conditions, including:
- Infectious Diseases: Gene therapies are being developed to treat HIV infection by delivering genes that interfere with viral replication or enhance immune function. Cell therapies are also being investigated for the treatment of infectious diseases by enhancing the immune response against pathogens.
- Autoimmune Disorders: CGTs are being explored for the treatment of autoimmune disorders by modulating the immune system. For example, CAR T-cell therapy targeting B cells is being investigated for the treatment of systemic lupus erythematosus (SLE) and rheumatoid arthritis.
- Cardiovascular Diseases: Gene therapies are being developed to treat cardiovascular diseases by delivering genes that promote angiogenesis (formation of new blood vessels), reduce inflammation, or improve cardiac function.
Many thanks to our sponsor Esdebe who helped us prepare this research report.
4. Manufacturing Challenges and Scalability
The widespread adoption of CGTs is hindered by significant manufacturing challenges and scalability issues. The complex and labor-intensive manufacturing processes, coupled with the need for specialized facilities and equipment, contribute to the high cost of these therapies.
4.1 Cost of Goods
The cost of goods (COGs) for CGTs is substantially higher than that of conventional pharmaceuticals. The COGs include the cost of raw materials, manufacturing equipment, personnel, quality control testing, and regulatory compliance. Reducing the COGs is crucial for making CGTs more accessible to patients.
4.2 Process Optimization and Automation
Process optimization and automation are essential for improving the efficiency and scalability of CGT manufacturing. Automation can reduce manual labor, minimize the risk of contamination, and improve product consistency. Process optimization involves identifying and eliminating bottlenecks in the manufacturing process to increase throughput and reduce COGs.
4.3 Supply Chain Management
The CGT supply chain is complex and involves the coordination of multiple stakeholders, including cell and gene therapy manufacturers, hospitals, apheresis centers, and logistics providers. Efficient supply chain management is crucial for ensuring the timely delivery of therapeutic products to patients. Challenges in supply chain management include maintaining product integrity during transport, managing cryogenic storage, and coordinating patient scheduling.
4.4 Allogeneic vs. Autologous Therapies
Allogeneic cell therapies, derived from healthy donors, offer the potential for off-the-shelf availability and reduced manufacturing costs compared to autologous therapies, which are patient-specific. However, allogeneic therapies carry the risk of graft-versus-host disease (GVHD), where the donor cells attack the recipient’s tissues. Strategies to mitigate GVHD include using immunosuppressive drugs or genetically modifying the donor cells to reduce their immunogenicity. The development of universal donor cells, such as induced pluripotent stem cells (iPSCs), holds promise for creating scalable and cost-effective allogeneic cell therapies.
Many thanks to our sponsor Esdebe who helped us prepare this research report.
5. Regulatory Landscape and Reimbursement
The regulatory landscape for CGTs is evolving rapidly as regulatory agencies grapple with the unique challenges posed by these innovative therapies. The US Food and Drug Administration (FDA) and the European Medicines Agency (EMA) have established specific regulatory pathways for CGTs to ensure their safety and efficacy. These pathways include accelerated approval mechanisms for therapies that address unmet medical needs.
5.1 Regulatory Pathways
The FDA has established the Regenerative Medicine Advanced Therapy (RMAT) designation to expedite the development and review of CGTs that have the potential to address unmet medical needs. The RMAT designation provides sponsors with increased opportunities for interaction with the FDA and allows for the use of surrogate endpoints to support accelerated approval.
5.2 Reimbursement Challenges
The high cost of CGTs poses significant reimbursement challenges for healthcare payers. Payers are grappling with how to value and reimburse these therapies, which often provide long-term or even curative benefits. Innovative reimbursement models, such as outcomes-based pricing and installment payments, are being explored to address these challenges.
Many thanks to our sponsor Esdebe who helped us prepare this research report.
6. Ethical Considerations
CGTs raise a number of important ethical considerations that must be carefully addressed to ensure their responsible development and use. These considerations include:
6.1 Germline Editing
Germline editing, which involves modifying the DNA of sperm, eggs, or embryos, raises profound ethical concerns because the changes would be passed on to future generations. There is a broad consensus against germline editing for reproductive purposes due to concerns about unintended consequences and the potential for exacerbating social inequalities. However, some argue that germline editing may be ethically permissible in certain limited circumstances, such as to prevent the transmission of serious genetic diseases when no other options are available.
6.2 Off-Target Effects
Gene editing technologies can sometimes cause off-target effects, where the editing machinery modifies DNA at unintended locations. These off-target effects can potentially lead to adverse health consequences, such as cancer. Minimizing off-target effects is a major focus of research in gene editing.
6.3 Access and Equity
The high cost of CGTs raises concerns about access and equity. These therapies may be unaffordable for many patients, particularly those in low- and middle-income countries. Ensuring equitable access to CGTs is a major challenge that requires innovative financing models and global collaboration.
6.4 Enhancement vs. Therapy
CGTs have the potential to be used for enhancement purposes, such as to improve physical or cognitive abilities. The use of CGTs for enhancement raises ethical concerns about fairness, social justice, and the potential for creating a genetically enhanced elite. There is a need for careful consideration of the ethical boundaries between therapy and enhancement.
Many thanks to our sponsor Esdebe who helped us prepare this research report.
7. Future Directions and Conclusion
The field of CGTs is rapidly evolving, with ongoing research and development efforts focused on improving the safety, efficacy, and accessibility of these therapies. Future directions include:
- Development of more precise and efficient gene editing technologies: Research is focused on minimizing off-target effects and improving the delivery of gene editing machinery to target cells.
- Engineering of novel viral vectors with improved tropism and safety profiles: Researchers are developing viral vectors that can target specific tissues and cell types with greater precision and reduce the risk of immunogenicity.
- Development of scalable and cost-effective manufacturing processes: Efforts are focused on automating and optimizing manufacturing processes to reduce COGs and increase throughput.
- Expansion of clinical applications to a wider range of diseases: CGTs are being explored for the treatment of a growing number of conditions, including neurodegenerative diseases, autoimmune disorders, and cardiovascular diseases.
- Development of personalized CGTs tailored to individual patients: Advances in genomics and precision medicine are enabling the development of CGTs that are tailored to the specific genetic and immunological characteristics of individual patients.
In conclusion, CGTs represent a paradigm shift in modern medicine, offering the potential to treat and even cure previously intractable diseases. The field has witnessed remarkable progress in recent years, driven by technological innovations and clinical successes. However, significant challenges remain in terms of manufacturing, cost, and ethical considerations. Continued research, collaboration, and responsible innovation are essential to realize the full potential of CGTs and ensure their equitable access to all patients.
Many thanks to our sponsor Esdebe who helped us prepare this research report.
References
- Naldini, L. (2015). Gene therapy returns to centre stage. Nature, 526(7573), 351-360.
- Bulpin, S., & Cosgrove, D. (2022). Cell and gene therapies: Challenges and opportunities in manufacturing. Drug Discovery Today, 27(6), 1692-1703.
- Ledford, H. (2020). CRISPR gene-editing tested in human patients for the first time. Nature, 578(7794), 205-206.
- O’Reilly, M., Gurusamy, D., Ryan, M., & Barry, F. (2019). Cell and gene therapies: The future of drug discovery. Drug Discovery Today, 24(12), 2294-2308.
- Zhang, F., Wen, Y., & Guo, X. (2017). CRISPR-Cas9 for genome editing: progress, implications and challenges. Human molecular genetics, 26(R1), R1-R11.
- Hayden, E. C. (2018). CRISPR: Gene editing is just the beginning. Nature, 557(7705), 299-301.
- US Food and Drug Administration. (n.d.). Cellular & Gene Therapy Products. Retrieved from https://www.fda.gov/vaccines-blood-biologics/cellular-gene-therapy-products/cellular-gene-therapy-products
- European Medicines Agency. (n.d.). Advanced therapy medicinal products: Overview. Retrieved from https://www.ema.europa.eu/en/human-regulatory/overview/advanced-therapy-medicinal-products
- National Academies of Sciences, Engineering, and Medicine. (2017). Human Genome Editing: Science, Ethics, and Governance. Washington, DC: The National Academies Press. https://doi.org/10.17226/24623
- Ginn, R. S., Veldman, G. M., Reitsma, B. A., Bleijenberg, N., van den Oetelaar, B. M. J., & de Vries, R. R. P. (2018). Gene therapy clinical trials worldwide to 2017—an update. The journal of gene medicine, 20(5), e3015.
- June, C. H., Sadelain, M., & Levine, B. L. (2015). CAR T-cell immunotherapy in cancer. New England Journal of Medicine, 373(5), 495-506.
- Adair, J. E., & Lawson, S. (2022). Allogeneic cell therapy: Current challenges and future directions. Expert Opinion on Biological Therapy, 22(3), 291-303.
- Introna, M., Barbanti, I., Cesana, L., Algarotti, A., Ubezio, P., & Golay, J. (2021). Manufacturing of allogeneic CAR-T cells: Current challenges and possible solutions. Blood Reviews, 48, 100791.
- Parekh, S., Donnelley, M., & Hogarth, R. (2021). Ethical implications of genome editing for human enhancement. BMC Medical Ethics, 22(1), 1-12.
The discussion of manufacturing challenges is crucial. Could innovative solutions like modular manufacturing units or decentralized production models help to overcome scalability issues and reduce costs, thus improving patient access to these potentially life-changing therapies?