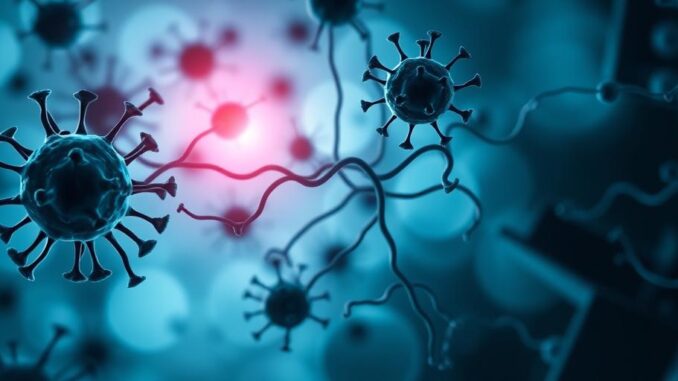
Abstract
Apoptosis, or programmed cell death, is a fundamental biological process critical for maintaining tissue homeostasis, development, and immunity. This highly regulated process eliminates unwanted or damaged cells without eliciting a significant inflammatory response. Dysregulation of apoptosis is implicated in a wide range of diseases, including cancer, autoimmune disorders, and neurodegenerative conditions. This research report provides an in-depth overview of apoptosis, encompassing its intricate molecular mechanisms, diverse physiological roles, and the therapeutic potential of manipulating apoptotic pathways. We delve into the major apoptotic pathways, including the intrinsic (mitochondrial) and extrinsic (death receptor) pathways, as well as the less-characterized perforin/granzyme pathway. Furthermore, we examine the crucial role of apoptosis in shaping the immune response, particularly in T cell development and the elimination of autoreactive lymphocytes. The report also explores the complex interplay between apoptosis and other cell death mechanisms, such as necrosis and autophagy, and discusses the implications of this interplay in disease pathogenesis. Finally, we critically evaluate current strategies for therapeutic modulation of apoptosis, focusing on both pro-apoptotic and anti-apoptotic approaches, and highlight the challenges and opportunities in developing targeted therapies that selectively manipulate apoptotic pathways in specific cell types, minimizing potential off-target effects. Specifically, we consider the therapeutic potential of shifting the balance of cell death in Tuberculosis infected cells from necrosis to apoptosis.
Many thanks to our sponsor Esdebe who helped us prepare this research report.
1. Introduction
Apoptosis, derived from the Greek word meaning “falling off” (as leaves from a tree), is a genetically encoded process of programmed cell death characterized by a distinct set of morphological and biochemical changes. It is a critical mechanism for maintaining tissue homeostasis, eliminating damaged or infected cells, and sculpting tissues during embryonic development. Unlike necrosis, which is a form of cell death induced by external factors (e.g., trauma, infection, or toxins) and results in cellular swelling, rupture, and inflammation, apoptosis is a tightly regulated process that proceeds without triggering a significant inflammatory response. The apoptotic cell undergoes characteristic morphological changes, including cell shrinkage, chromatin condensation, DNA fragmentation, and the formation of apoptotic bodies, which are subsequently engulfed by phagocytes. Dysregulation of apoptosis is implicated in a wide spectrum of human diseases, including cancer, autoimmune disorders, neurodegenerative diseases, and infectious diseases. Understanding the intricate molecular mechanisms that govern apoptosis is crucial for developing effective therapeutic strategies to target these diseases.
Many thanks to our sponsor Esdebe who helped us prepare this research report.
2. Molecular Mechanisms of Apoptosis
Apoptosis is executed through a complex cascade of molecular events involving a variety of signaling pathways and effector proteins. The process can be broadly divided into two major pathways: the intrinsic (mitochondrial) pathway and the extrinsic (death receptor) pathway. While these pathways can operate independently, they often converge to activate a common set of executioner caspases, ultimately leading to cell death.
2.1 The Intrinsic (Mitochondrial) Pathway
The intrinsic pathway, also known as the mitochondrial pathway, is initiated by intracellular stress signals, such as DNA damage, oxidative stress, growth factor deprivation, and endoplasmic reticulum (ER) stress. These signals trigger the activation of pro-apoptotic members of the Bcl-2 family of proteins, including Bax and Bak. Bax and Bak undergo conformational changes and oligomerize in the outer mitochondrial membrane, leading to mitochondrial outer membrane permeabilization (MOMP). MOMP results in the release of several pro-apoptotic factors from the mitochondrial intermembrane space into the cytoplasm, including cytochrome c, Smac/DIABLO, and Omi/HtrA2. Cytochrome c binds to Apaf-1 (apoptotic protease activating factor-1) in the cytoplasm, forming the apoptosome complex. The apoptosome then recruits and activates pro-caspase-9, initiating the caspase cascade. Smac/DIABLO inhibits the activity of IAPs (inhibitor of apoptosis proteins), which are endogenous inhibitors of caspases, thereby promoting caspase activation. The balance between pro-apoptotic (e.g., Bax, Bak, Bid, Bim, Puma) and anti-apoptotic (e.g., Bcl-2, Bcl-xL, Mcl-1) Bcl-2 family members determines the cell’s susceptibility to apoptosis.
2.2 The Extrinsic (Death Receptor) Pathway
The extrinsic pathway is triggered by the binding of death ligands to their cognate death receptors on the cell surface. Death receptors belong to the tumor necrosis factor receptor (TNFR) superfamily and include Fas (CD95), TNF-R1, TRAIL-R1 (DR4), and TRAIL-R2 (DR5). Upon ligand binding, death receptors recruit adaptor proteins, such as FADD (Fas-associated death domain protein), to form the death-inducing signaling complex (DISC). The DISC recruits and activates pro-caspase-8 (or pro-caspase-10 in some cell types), initiating the caspase cascade. In type I cells, caspase-8 activation at the DISC is sufficient to directly activate executioner caspases. In type II cells, however, caspase-8 activation is limited, and the intrinsic pathway is required for efficient apoptosis. In these cells, caspase-8 cleaves Bid, a pro-apoptotic Bcl-2 family member, into truncated Bid (tBid), which translocates to the mitochondria and promotes MOMP, thereby linking the extrinsic and intrinsic pathways.
2.3 Execution Phase of Apoptosis
Both the intrinsic and extrinsic pathways converge on the activation of executioner caspases, primarily caspase-3, caspase-6, and caspase-7. These caspases cleave a variety of intracellular substrates, leading to the characteristic morphological and biochemical changes associated with apoptosis. Key substrates of executioner caspases include ICAD (inhibitor of caspase-activated DNase), which, when cleaved, releases CAD (caspase-activated DNase), leading to DNA fragmentation; and structural proteins such as actin and lamin, which contribute to cell shrinkage and cytoskeletal disassembly. The coordinated action of these caspases ultimately leads to cell disassembly and the formation of apoptotic bodies.
2.4 Perforin/Granzyme Pathway
Another important pathway involves cytotoxic T lymphocytes (CTLs) and natural killer (NK) cells, which induce apoptosis in target cells through the release of perforin and granzymes. Perforin creates pores in the target cell membrane, allowing granzymes to enter the cell. Granzyme B, a serine protease, directly activates caspases, leading to apoptosis. This pathway is crucial for eliminating virus-infected cells and tumor cells.
Many thanks to our sponsor Esdebe who helped us prepare this research report.
3. Regulation of Apoptosis
The apoptotic process is tightly regulated at multiple levels to ensure that cell death occurs only when necessary and to prevent inappropriate or premature apoptosis. This regulation involves a complex interplay of pro-apoptotic and anti-apoptotic factors, as well as post-translational modifications, such as phosphorylation and ubiquitination.
3.1 Bcl-2 Family Proteins
The Bcl-2 family of proteins plays a central role in regulating the intrinsic pathway of apoptosis. This family comprises both pro-apoptotic (e.g., Bax, Bak, Bid, Bim, Puma, Bad) and anti-apoptotic (e.g., Bcl-2, Bcl-xL, Mcl-1) members. The balance between these opposing forces determines the cell’s susceptibility to apoptosis. Anti-apoptotic Bcl-2 family members inhibit apoptosis by binding to and neutralizing pro-apoptotic members, preventing their oligomerization and MOMP. Pro-apoptotic BH3-only proteins, such as Bid, Bim, and Puma, can initiate apoptosis by either directly activating Bax and Bak or by displacing anti-apoptotic proteins from Bax and Bak.
3.2 Inhibitor of Apoptosis Proteins (IAPs)
IAPs are a family of proteins that inhibit apoptosis by directly binding to and inhibiting caspases. They also promote the ubiquitination and degradation of caspases. Several IAPs have been identified, including XIAP (X-linked inhibitor of apoptosis protein), cIAP1, cIAP2, and survivin. Smac/DIABLO, released from the mitochondria during apoptosis, antagonizes IAPs, thereby promoting caspase activation.
3.3 Post-translational Modifications
Post-translational modifications, such as phosphorylation and ubiquitination, play a crucial role in regulating the activity and stability of apoptotic proteins. For example, phosphorylation of Bad can prevent its binding to Bcl-2, thereby promoting cell survival. Ubiquitination can target apoptotic proteins for degradation by the proteasome, or it can alter their activity or localization.
Many thanks to our sponsor Esdebe who helped us prepare this research report.
4. Physiological Roles of Apoptosis
Apoptosis plays a vital role in a wide range of physiological processes, including:
4.1 Development
Apoptosis is essential for normal embryonic development, shaping tissues and organs by eliminating unwanted cells. For example, apoptosis is responsible for sculpting digits during limb development, removing interdigital webbing, and eliminating excess neurons during brain development. Failure of apoptosis during development can lead to birth defects and developmental abnormalities.
4.2 Immune System
Apoptosis is critical for the proper development and function of the immune system. It is involved in the selection of T cells in the thymus, eliminating autoreactive T cells that could attack the body’s own tissues. Apoptosis also plays a role in the elimination of activated lymphocytes after an immune response has resolved, preventing chronic inflammation. Furthermore, apoptosis is used by cytotoxic T lymphocytes and natural killer cells to kill infected or cancerous cells.
4.3 Tissue Homeostasis
Apoptosis maintains tissue homeostasis by balancing cell proliferation and cell death. It eliminates damaged or aged cells, preventing the accumulation of potentially harmful cells. Dysregulation of apoptosis can disrupt tissue homeostasis and contribute to the development of diseases such as cancer and autoimmune disorders.
Many thanks to our sponsor Esdebe who helped us prepare this research report.
5. Apoptosis in Disease
Dysregulation of apoptosis is implicated in a wide range of human diseases.
5.1 Cancer
In cancer, apoptosis is often inhibited, allowing cells with DNA damage or other abnormalities to survive and proliferate uncontrollably. This resistance to apoptosis is a hallmark of cancer and contributes to tumor growth, metastasis, and resistance to therapy. Many cancer cells acquire mutations in genes that regulate apoptosis, such as p53, Bcl-2 family members, and caspases. Overexpression of anti-apoptotic proteins, such as Bcl-2, is a common mechanism of apoptosis resistance in cancer.
5.2 Autoimmune Disorders
In autoimmune disorders, apoptosis is often impaired, leading to the survival of autoreactive lymphocytes that attack the body’s own tissues. This can result in chronic inflammation and tissue damage. Defects in apoptosis pathways have been implicated in several autoimmune diseases, including systemic lupus erythematosus (SLE), rheumatoid arthritis, and multiple sclerosis.
5.3 Neurodegenerative Diseases
In neurodegenerative diseases, such as Alzheimer’s disease, Parkinson’s disease, and Huntington’s disease, excessive apoptosis of neurons contributes to neuronal loss and cognitive decline. Various factors can trigger apoptosis in neurons, including oxidative stress, excitotoxicity, and protein misfolding. Accumulation of misfolded proteins, such as amyloid-beta plaques in Alzheimer’s disease and alpha-synuclein aggregates in Parkinson’s disease, can activate apoptotic pathways.
5.4 Infectious Diseases
In some infectious diseases, pathogens can either inhibit or induce apoptosis in host cells to promote their own survival and replication. For example, some viruses, such as HIV, can inhibit apoptosis in infected cells, allowing them to persist and produce more virus. Conversely, other pathogens can induce apoptosis in immune cells, suppressing the host’s immune response.
In the context of Tuberculosis (TB), Mycobacterium tuberculosis (Mtb) can manipulate apoptosis in infected macrophages to its advantage. Initially, Mtb inhibits apoptosis to establish an intracellular niche. However, at later stages, it can induce necrosis, a form of cell death that promotes bacterial dissemination. Shifting the balance from necrosis to apoptosis in TB-infected cells, as suggested by the article referring to navitoclax, could be a therapeutic strategy to contain the infection by promoting the clearance of infected cells without triggering the inflammatory response associated with necrosis. Apoptotic macrophages are efficiently phagocytosed by other macrophages, a process that can enhance antigen presentation and promote a more effective adaptive immune response. Moreover, intracellular Mtb is killed during the apoptotic process by lysosomal enzymes and Reactive oxygen species. Therefore, induction of apoptosis can also suppress the growth and spread of TB.
Many thanks to our sponsor Esdebe who helped us prepare this research report.
6. Therapeutic Modulation of Apoptosis
Given the critical role of apoptosis in disease, therapeutic modulation of apoptotic pathways has emerged as a promising strategy for treating a variety of conditions. Both pro-apoptotic and anti-apoptotic approaches are being explored.
6.1 Pro-apoptotic Therapies
Pro-apoptotic therapies aim to induce apoptosis in diseased cells, particularly cancer cells. Several strategies are being developed to achieve this goal, including:
- BH3 mimetics: These are small-molecule drugs that mimic the action of BH3-only proteins, such as Bid and Bim. They bind to and inhibit anti-apoptotic Bcl-2 family members, such as Bcl-2, Bcl-xL, and Mcl-1, thereby releasing Bax and Bak and triggering MOMP. Navitoclax, ABT-199 (venetoclax), and obatoclax are examples of BH3 mimetics that have shown promising results in clinical trials.
- Death receptor agonists: These are antibodies or recombinant ligands that bind to and activate death receptors, such as Fas, TNF-R1, TRAIL-R1, and TRAIL-R2, triggering the extrinsic pathway of apoptosis. TRAIL-based therapies have shown some success in preclinical studies and clinical trials, but resistance mechanisms remain a challenge.
- Caspase activators: These are drugs that directly activate caspases, bypassing the need for upstream signaling events. Several caspase activators are in development, but their selectivity and potential toxicity need to be carefully evaluated.
- Inhibitors of IAPs: These are small-molecule drugs that inhibit the activity of IAPs, such as XIAP, cIAP1, and cIAP2, thereby promoting caspase activation and apoptosis. Smac mimetics are examples of IAP inhibitors that have shown promising results in preclinical studies and clinical trials.
- DNA damaging agents: Many conventional chemotherapeutic agents, such as cisplatin and doxorubicin, induce apoptosis by damaging DNA. However, these agents are often toxic to normal cells as well.
6.2 Anti-apoptotic Therapies
Anti-apoptotic therapies aim to inhibit apoptosis in diseases where excessive cell death contributes to pathogenesis, such as neurodegenerative diseases and ischemic injury. Several strategies are being developed to achieve this goal, including:
- Bcl-2 inhibitors: Antisense oligonucleotides or small interfering RNAs (siRNAs) that target Bcl-2 mRNA can reduce Bcl-2 expression and promote cell survival in certain contexts.
- Caspase inhibitors: These are drugs that directly inhibit caspases, preventing their activation and downstream signaling events. Caspase inhibitors have shown some promise in preclinical studies of neurodegenerative diseases and ischemic injury, but their clinical efficacy remains to be established.
- Growth factors: Growth factors, such as nerve growth factor (NGF) and brain-derived neurotrophic factor (BDNF), can promote cell survival by activating intracellular signaling pathways that inhibit apoptosis.
6.3 Challenges and Opportunities
Therapeutic modulation of apoptosis faces several challenges, including:
- Selectivity: Many pro-apoptotic and anti-apoptotic agents lack sufficient selectivity, leading to off-target effects and toxicity to normal cells. Developing targeted therapies that selectively manipulate apoptotic pathways in specific cell types is a major goal.
- Resistance: Cancer cells can develop resistance to pro-apoptotic therapies through various mechanisms, such as mutations in apoptotic genes, overexpression of anti-apoptotic proteins, and activation of alternative survival pathways. Overcoming resistance mechanisms is crucial for improving the efficacy of pro-apoptotic therapies.
- Delivery: Efficient delivery of therapeutic agents to target cells can be a challenge, particularly in the brain and other difficult-to-reach tissues. Developing novel drug delivery systems, such as nanoparticles and viral vectors, can improve the delivery of apoptotic modulators.
Despite these challenges, therapeutic modulation of apoptosis holds great promise for treating a wide range of diseases. Advances in our understanding of the molecular mechanisms of apoptosis and the development of new technologies are paving the way for the development of more effective and targeted therapies.
Many thanks to our sponsor Esdebe who helped us prepare this research report.
7. Conclusion
Apoptosis is a fundamental biological process that plays a critical role in development, immunity, and tissue homeostasis. Dysregulation of apoptosis is implicated in a wide range of diseases, including cancer, autoimmune disorders, neurodegenerative diseases, and infectious diseases. Understanding the intricate molecular mechanisms that govern apoptosis is crucial for developing effective therapeutic strategies to target these diseases. Therapeutic modulation of apoptotic pathways, using both pro-apoptotic and anti-apoptotic approaches, holds great promise for treating a variety of conditions. However, challenges remain in achieving selectivity, overcoming resistance, and improving drug delivery. Continued research in this area is essential for developing more effective and targeted therapies that can harness the power of apoptosis to improve human health.
Many thanks to our sponsor Esdebe who helped us prepare this research report.
References
- Elmore, S. (2007). Apoptosis: a review of programmed cell death. Toxicologic Pathology, 35(4), 495-516.
- Green, D. R. (2011). Means to an end: apoptosis and other cell death mechanisms. Science translational medicine, 3(81), 81rv3.
- Kerr, J. F., Wyllie, A. H., & Currie, A. R. (1972). Apoptosis: a basic biological phenomenon with wide-ranging implications in tissue kinetics. British journal of cancer, 26(4), 239.
- Kroemer, G., Galluzzi, L., Vandenabeele, P., Abrams, J., Alnemri, E. S., Baehrecke, E. H., … & Madeo, F. (2009). Classification of cell death: recommendations of the Nomenclature Committee on Cell Death 2009. Cell death & differentiation, 16(1), 3-11.
- Lakhani, S. A., Camilleri, P., Kole, L., Keeble, J., Kumar, S., Allen, A. H., … & Strasser, A. (2012). Targeting Bcl-2 family proteins for cancer therapy. Nature reviews Drug discovery, 11(11), 841-852.
- Ola, M. S., Nawaz, M., & Ahsan, H. (2011). Role of Bcl-2 family proteins and caspases in the regulation of apoptosis. Molecular and cellular biochemistry, 351(1-2), 41-58.
- Wong, R. S. (2011). Apoptosis in cancer: from pathogenesis to treatment. Journal of experimental & clinical cancer research, 30(1), 87.
- Zumla, A., George, A., Sharma, V., Herbert, L., Oliver, M., Meermeier, E., … & Maecker, H. (2013). Host-directed therapies for tuberculosis: current status and future perspectives. The Lancet infectious diseases, 13(11), 993-1007.
Wow, I never thought I’d be contemplating cell death mechanisms over my morning coffee! Shifting the balance from necrosis to apoptosis in TB-infected cells? Suddenly, I’m picturing tiny cellular dance-offs where the winner gets… engulfed? Riveting stuff!
Glad you found it riveting! The idea of cellular dance-offs is definitely a memorable way to think about it. It really highlights how dynamic these processes are, especially when considering the shift from necrosis to apoptosis in diseases like TB. Perhaps we should choreograph the next scientific animation!
Editor: MedTechNews.Uk
Thank you to our Sponsor Esdebe
So, apoptosis sculpts our digits! Does that mean my clumsy typing is just a developmental delay waiting to be… corrected? Perhaps a bit more programmed cell death in the right places could finally make me a coding ninja.
That’s a hilarious thought! While apoptosis gives us our fingers, I suspect coding ninja skills are more about synaptic plasticity than cellular pruning. Perhaps focusing on rewiring those neural pathways through practice could be more effective (and less drastic) than hoping for targeted cell death!
Editor: MedTechNews.Uk
Thank you to our Sponsor Esdebe
The discussion on shifting the balance from necrosis to apoptosis in TB-infected cells is compelling. Exploiting the perforin/granzyme pathway to enhance apoptosis in these cells could be a promising avenue for therapeutic intervention, potentially minimizing inflammation and improving immune response.