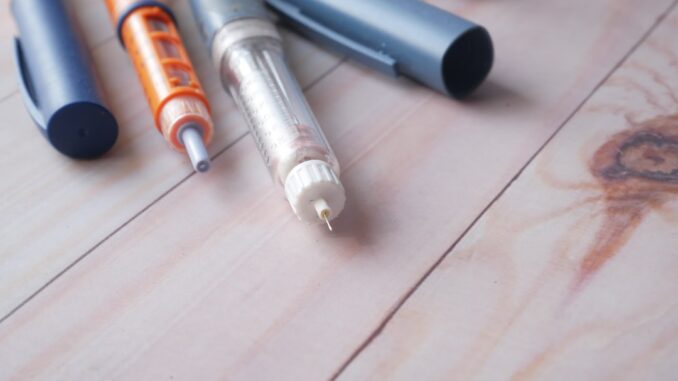
Abstract
Beta-cells, the sole producers of insulin within the pancreatic islets of Langerhans, are critical for glucose homeostasis. Their dysfunction or destruction leads to diabetes mellitus, with Type 1 Diabetes (T1D) characterized by autoimmune-mediated beta-cell ablation. While significant progress has been made in understanding the pathogenesis of T1D, the complexity of beta-cell biology and its intricate interplay with the immune system, metabolic demands, and genetic predispositions necessitate a broader perspective. This review delves into the multifaceted aspects of beta-cell biology, encompassing their development, function, and vulnerability in the context of both T1D and related metabolic disorders. We explore the intricate molecular mechanisms regulating insulin secretion, the impact of environmental factors on beta-cell health, and the genetic landscape influencing susceptibility to T1D. Furthermore, we critically evaluate current and emerging therapeutic strategies aimed at beta-cell protection, regeneration, and replacement, including immunomodulatory therapies, stem cell-derived beta-cells, and novel approaches targeting beta-cell metabolism and survival. Finally, we examine the role of beta-cell dysfunction in Type 2 Diabetes (T2D) and explore novel therapeutic interventions that promote beta cell health. A focus is given on the interplay between endoplasmic reticulum (ER) stress, oxidative stress, and islet amyloid polypeptide (IAPP) aggregation in the decline of beta cell function. Through a comprehensive analysis of these diverse areas, this review aims to provide a holistic understanding of beta-cell dynamics and highlight promising avenues for future research and therapeutic interventions targeting diabetes and associated metabolic complications.
Many thanks to our sponsor Esdebe who helped us prepare this research report.
1. Introduction
The central role of pancreatic beta-cells in maintaining glucose homeostasis is undeniable. These specialized endocrine cells, residing within the islets of Langerhans, respond to rising blood glucose levels by synthesizing and secreting insulin. Insulin, in turn, promotes glucose uptake and utilization by peripheral tissues, effectively lowering blood glucose and preventing hyperglycemia. The consequences of beta-cell dysfunction or loss are profound, leading to the development of diabetes mellitus. Type 1 Diabetes (T1D) is characterized by an autoimmune attack on beta-cells, resulting in their progressive destruction and absolute insulin deficiency. Type 2 Diabetes (T2D) is characterized by insulin resistance, but eventually includes a decline in beta cell function and cell number. Understanding the intricacies of beta-cell biology, including their development, function, and vulnerability, is therefore paramount for developing effective strategies to prevent and treat diabetes. While much research has focused on the autoimmune aspects of T1D, a deeper understanding of the beta-cell itself, its susceptibility to environmental insults, and its capacity for regeneration is crucial. Further, the role of the beta-cell in T2D has been under appreciated, and this report will address this subject.
This review aims to provide a comprehensive overview of beta-cell dynamics, encompassing molecular mechanisms, genetic factors, environmental influences, and therapeutic interventions. We will explore the following key areas:
- Beta-Cell Development and Differentiation: Examining the genetic and signaling pathways that govern beta-cell formation during embryonic development and the potential for generating beta-cells from stem cells.
- Beta-Cell Function and Insulin Secretion: Delving into the intricate molecular mechanisms underlying glucose-stimulated insulin secretion (GSIS) and the factors that modulate beta-cell responsiveness.
- Beta-Cell Vulnerability in T1D: Investigating the autoimmune processes that target beta-cells in T1D, including the role of autoantigens, immune cells, and inflammatory cytokines.
- Therapeutic Strategies for Beta-Cell Protection and Regeneration: Evaluating current and emerging approaches for preserving existing beta-cells and promoting the formation of new beta-cells, including immunomodulatory therapies, islet transplantation, stem cell-derived beta-cells, and pharmacological interventions.
- Beta-Cell Dysfunction in T2D: Understanding the role of beta-cell failure in the pathogenesis of T2D, including the impact of glucotoxicity, lipotoxicity, ER stress, and IAPP aggregation.
- Beta-Cell Biomarkers and Clinical Monitoring: Identifying and validating biomarkers for assessing beta-cell mass, function, and vulnerability in clinical settings.
By integrating these diverse perspectives, this review seeks to provide a holistic understanding of beta-cell dynamics and to highlight promising avenues for future research and therapeutic development.
Many thanks to our sponsor Esdebe who helped us prepare this research report.
2. Beta-Cell Development and Differentiation
The genesis of functional beta-cells is a complex and tightly regulated process, beginning during embryonic development and continuing, albeit at a much slower rate, throughout adulthood. Understanding the developmental pathways involved in beta-cell formation is critical for developing strategies to generate beta-cells from stem cells for therapeutic purposes.
2.1 Embryonic Beta-Cell Development
Beta-cell development originates from the definitive endoderm, the precursor to the pancreas, during early embryogenesis. Key transcription factors, including PDX1, NGN3, PAX4, and NKX6.1, play crucial roles in the differentiation of pancreatic progenitor cells into endocrine cells, specifically beta-cells [1]. PDX1, a homeodomain transcription factor, is essential for pancreas formation and beta-cell differentiation. NGN3, a basic helix-loop-helix transcription factor, is a master regulator of endocrine cell fate, driving progenitor cells towards an endocrine lineage. PAX4 and NKX6.1 are downstream targets of NGN3 and are specifically required for beta-cell and delta-cell development. The sequential expression and interaction of these transcription factors orchestrate the complex developmental program that leads to the formation of mature, insulin-secreting beta-cells.
2.2 Beta-Cell Neogenesis and Replication
While the majority of beta-cells are formed during embryonic development, evidence suggests that beta-cell neogenesis (formation of new beta-cells from progenitor cells) and replication of existing beta-cells can occur in adulthood [2]. Beta-cell neogenesis is thought to be stimulated by factors such as pregnancy, obesity, and certain growth factors. However, the extent to which beta-cell neogenesis contributes to beta-cell mass in adults is still debated. Beta-cell replication is another mechanism for expanding beta-cell mass, particularly in response to increased insulin demand. Glucose, growth factors, and hormones can stimulate beta-cell replication. However, the replicative capacity of beta-cells declines with age and in the context of diabetes.
2.3 Stem Cell-Derived Beta-Cells
The limited availability of cadaveric islets for transplantation and the challenges associated with beta-cell regeneration have spurred intense research into generating beta-cells from stem cells. Both embryonic stem cells (ESCs) and induced pluripotent stem cells (iPSCs) can be differentiated into beta-like cells in vitro using defined protocols that mimic the developmental pathways involved in beta-cell formation [3]. These stem cell-derived beta-cells (SC-beta-cells) have shown promising results in preclinical studies, demonstrating the ability to secrete insulin in response to glucose stimulation and to reverse hyperglycemia in diabetic animal models. However, several challenges remain before SC-beta-cells can be widely used for therapeutic purposes, including improving their glucose responsiveness, preventing immune rejection, and ensuring their long-term survival and function in vivo. Another limitation is the propensity for the stem cells to develop into non-desired cell types that can be detrimental in the final formulation for injection.
Many thanks to our sponsor Esdebe who helped us prepare this research report.
3. Beta-Cell Function and Insulin Secretion
The primary function of beta-cells is to synthesize, store, and secrete insulin in response to changes in blood glucose levels. Glucose-stimulated insulin secretion (GSIS) is a highly regulated process involving a series of intricate molecular events.
3.1 Molecular Mechanisms of GSIS
GSIS is initiated by the uptake of glucose into beta-cells via the GLUT2 transporter (in humans and rodents). Once inside the cell, glucose is phosphorylated by glucokinase (GCK), the rate-limiting enzyme in glucose metabolism [4]. The subsequent metabolism of glucose through glycolysis and oxidative phosphorylation leads to an increase in the ATP/ADP ratio. This increase in ATP inhibits ATP-sensitive potassium (KATP) channels, causing the plasma membrane to depolarize. Membrane depolarization activates voltage-gated calcium channels (VGCCs), allowing calcium ions to enter the cell. The rise in intracellular calcium triggers the fusion of insulin-containing granules with the plasma membrane and the release of insulin into the bloodstream. Other nutrients, such as amino acids and fatty acids, can also stimulate insulin secretion, albeit to a lesser extent than glucose. These nutrient stimuli enhance insulin secretion through various mechanisms, including increasing ATP production and modulating calcium influx.
3.2 Regulation of Insulin Secretion
Insulin secretion is tightly regulated by a variety of factors, including hormones, neurotransmitters, and paracrine signals. Glucagon-like peptide-1 (GLP-1), an incretin hormone released from the gut in response to food intake, enhances GSIS by activating GLP-1 receptors on beta-cells [5]. GLP-1 signaling increases cAMP levels, which potentiates calcium influx and insulin granule exocytosis. Somatostatin, a hormone produced by delta-cells within the islets, inhibits insulin secretion by activating somatostatin receptors on beta-cells. Activation of somatostatin receptors inhibits adenylate cyclase, reducing cAMP levels and suppressing calcium influx. The autonomic nervous system also plays a role in regulating insulin secretion. Sympathetic stimulation inhibits insulin secretion via alpha2-adrenergic receptors, while parasympathetic stimulation enhances insulin secretion via muscarinic receptors.
3.3 Factors Affecting Beta-Cell Responsiveness
Beta-cell responsiveness to glucose and other stimuli can be affected by a variety of factors, including age, obesity, inflammation, and genetic predisposition. Aging is associated with a decline in beta-cell function, characterized by decreased GSIS and reduced beta-cell mass. Obesity is a major risk factor for T2D and is associated with insulin resistance and compensatory hyperinsulinemia. Chronic exposure to high glucose and fatty acid levels (glucotoxicity and lipotoxicity, respectively) can impair beta-cell function and lead to beta-cell apoptosis. Inflammation, particularly in the context of obesity and T1D, can also impair beta-cell function by releasing inflammatory cytokines that disrupt insulin signaling and induce beta-cell stress. Genetic factors play a significant role in determining beta-cell function and susceptibility to diabetes. Variations in genes encoding key proteins involved in insulin secretion, such as GCK, KATP channels, and VGCCs, can affect beta-cell responsiveness and increase the risk of diabetes. Specifically, the GCK gene variants may lead to Maturity-Onset Diabetes of the Young type 2 (MODY2).
Many thanks to our sponsor Esdebe who helped us prepare this research report.
4. Beta-Cell Vulnerability in T1D
Type 1 Diabetes (T1D) is an autoimmune disease characterized by the selective destruction of insulin-producing beta-cells by the immune system. This immune-mediated destruction leads to absolute insulin deficiency and requires lifelong insulin therapy. Understanding the mechanisms underlying beta-cell autoimmunity is crucial for developing strategies to prevent and treat T1D.
4.1 Autoimmune Mechanisms in T1D
The pathogenesis of T1D is complex and involves a combination of genetic predisposition, environmental triggers, and immune dysregulation [6]. Individuals with certain human leukocyte antigen (HLA) alleles, particularly HLA-DR3 and HLA-DR4, have an increased risk of developing T1D. These HLA alleles present beta-cell autoantigens to T cells, initiating the autoimmune response. Environmental factors, such as viral infections, may also trigger T1D in genetically susceptible individuals. Viral infections can lead to the release of beta-cell antigens and the activation of autoreactive T cells. The immune response in T1D is characterized by the infiltration of immune cells, including T lymphocytes, B lymphocytes, and macrophages, into the pancreatic islets. These immune cells release inflammatory cytokines, such as IFN-γ, TNF-α, and IL-1β, which directly damage beta-cells and promote their apoptosis. Cytotoxic T lymphocytes (CTLs) directly kill beta-cells by recognizing beta-cell autoantigens presented on MHC class I molecules. Helper T cells (Th1 cells) release cytokines that activate macrophages and promote CTL activity. B lymphocytes produce autoantibodies against beta-cell antigens, which may contribute to beta-cell destruction through antibody-dependent cell-mediated cytotoxicity (ADCC) or complement-mediated cytotoxicity (CDC).
4.2 Beta-Cell Autoantigens
Several beta-cell antigens have been identified as targets of the autoimmune response in T1D. These include insulin, glutamic acid decarboxylase (GAD65), islet cell autoantigen 512 (ICA512, also known as IA-2), and zinc transporter 8 (ZnT8) [7]. Insulin is a major autoantigen in T1D, and autoantibodies against insulin are frequently detected in individuals at risk of developing T1D. GAD65 is an enzyme involved in the synthesis of GABA, an inhibitory neurotransmitter. ICA512 is a transmembrane protein involved in insulin granule trafficking. ZnT8 is a zinc transporter specifically expressed in beta-cells and is involved in insulin packaging and secretion. Autoantibodies and T cells recognizing these beta-cell antigens are thought to play a role in the pathogenesis of T1D.
4.3 Beta-Cell Stress and Autoimmunity
Beta-cell stress, induced by factors such as glucotoxicity, lipotoxicity, and ER stress, can exacerbate autoimmunity in T1D. Stressed beta-cells release danger-associated molecular patterns (DAMPs), which activate innate immune cells and promote inflammation. ER stress, caused by the accumulation of misfolded proteins in the endoplasmic reticulum, can trigger beta-cell apoptosis and release of autoantigens. Inflammatory cytokines, such as IL-1β, can induce beta-cell ER stress and further amplify the autoimmune response. Additionally, beta-cell dysfunction can alter the presentation of autoantigens, making them more susceptible to immune recognition. Overall, the autoimmune destruction of beta-cells in T1D is a complex process involving a combination of genetic predisposition, environmental triggers, and immune dysregulation, with beta-cell stress playing a significant role in amplifying the autoimmune response.
Many thanks to our sponsor Esdebe who helped us prepare this research report.
5. Therapeutic Strategies for Beta-Cell Protection and Regeneration
Given the critical role of beta-cells in glucose homeostasis, therapeutic strategies aimed at protecting existing beta-cells and promoting the regeneration of new beta-cells hold great promise for the prevention and treatment of diabetes.
5.1 Immunomodulatory Therapies
Immunomodulatory therapies aim to suppress the autoimmune response that destroys beta-cells in T1D. Several immunomodulatory agents have been evaluated in clinical trials, including anti-CD3 antibodies, anti-CD20 antibodies, and CTLA-4 agonists [8]. Anti-CD3 antibodies, such as teplizumab, bind to the CD3 receptor on T cells and modulate T cell function, leading to a transient depletion of T cells and a reduction in inflammation. Anti-CD20 antibodies, such as rituximab, deplete B cells, which are involved in autoantibody production and antigen presentation. CTLA-4 agonists, such as abatacept, block the interaction between CD28 on T cells and B7 on antigen-presenting cells, inhibiting T cell activation. While these immunomodulatory therapies have shown some promise in preserving beta-cell function in individuals with recent-onset T1D, they have not been able to completely prevent beta-cell destruction. Furthermore, these therapies are associated with potential side effects, such as infections and autoimmune complications. Combination therapies targeting multiple immune pathways may be more effective in preventing beta-cell destruction in T1D.
5.2 Islet Transplantation
Islet transplantation involves the infusion of isolated islets from deceased donors into the liver of individuals with T1D. Transplanted islets can engraft in the liver and produce insulin, reducing or eliminating the need for exogenous insulin injections [9]. However, islet transplantation requires lifelong immunosuppression to prevent rejection of the transplanted islets. Immunosuppression is associated with significant side effects, such as infections, kidney damage, and increased risk of cancer. Furthermore, the limited availability of donor islets is a major obstacle to the widespread use of islet transplantation. Researchers are exploring strategies to overcome these limitations, including encapsulating islets in biocompatible materials to protect them from immune attack, using stem cell-derived beta-cells as an alternative source of islets, and developing more targeted and less toxic immunosuppressive regimens.
5.3 Stem Cell-Derived Beta-Cells for Replacement Therapy
As mentioned previously, stem cell-derived beta-cells (SC-beta-cells) hold great promise as an alternative source of beta-cells for transplantation. Researchers have developed protocols to differentiate both embryonic stem cells (ESCs) and induced pluripotent stem cells (iPSCs) into beta-like cells that can secrete insulin in response to glucose stimulation [3]. These SC-beta-cells have shown promising results in preclinical studies, demonstrating the ability to reverse hyperglycemia in diabetic animal models. However, several challenges remain before SC-beta-cells can be widely used for therapeutic purposes, including improving their glucose responsiveness, preventing immune rejection, and ensuring their long-term survival and function in vivo. Furthermore, the high cost of stem cell therapy may limit the scalability of the treatment.
5.4 Pharmacological Interventions
Several pharmacological agents have been shown to promote beta-cell protection and regeneration in preclinical studies. Glucagon-like peptide-1 (GLP-1) receptor agonists, such as exenatide and liraglutide, enhance GSIS, stimulate beta-cell proliferation, and protect beta-cells from apoptosis [5]. Dipeptidyl peptidase-4 (DPP-4) inhibitors, such as sitagliptin and saxagliptin, prevent the degradation of endogenous GLP-1, thereby increasing GLP-1 levels and promoting beta-cell function. Other pharmacological agents that have shown promise for beta-cell protection and regeneration include antioxidants, anti-inflammatory agents, and growth factors. Combination therapies targeting multiple pathways involved in beta-cell survival and function may be more effective than single-agent therapies.
Many thanks to our sponsor Esdebe who helped us prepare this research report.
6. Beta-Cell Dysfunction in T2D
Type 2 Diabetes (T2D) is characterized by insulin resistance and progressive beta-cell dysfunction. While insulin resistance is often the initial defect in T2D, beta-cell failure is ultimately required for the development of sustained hyperglycemia. Understanding the mechanisms underlying beta-cell dysfunction in T2D is crucial for developing strategies to prevent and treat this increasingly prevalent disease.
6.1 Glucotoxicity and Lipotoxicity
Chronic exposure to high glucose and fatty acid levels (glucotoxicity and lipotoxicity, respectively) can impair beta-cell function and lead to beta-cell apoptosis [10]. Glucotoxicity impairs GSIS by desensitizing beta-cells to glucose stimulation and by disrupting insulin gene expression. Lipotoxicity impairs beta-cell function by accumulating toxic lipid intermediates, such as ceramides and diacylglycerols, which disrupt insulin signaling and induce ER stress. Elevated free fatty acids are also proposed to lead to oxidative stress.
6.2 ER Stress and Oxidative Stress
Endoplasmic reticulum (ER) stress, caused by the accumulation of misfolded proteins in the ER, is a major contributor to beta-cell dysfunction in T2D. ER stress activates the unfolded protein response (UPR), a cellular signaling pathway that attempts to restore ER homeostasis. However, chronic ER stress can lead to beta-cell apoptosis. Oxidative stress, caused by an imbalance between the production of reactive oxygen species (ROS) and the antioxidant capacity of the cell, can also impair beta-cell function and lead to beta-cell apoptosis. Hyperglycemia and lipotoxicity can both increase ROS production in beta-cells. The interplay between ER stress and oxidative stress creates a vicious cycle that exacerbates beta-cell dysfunction in T2D. Excessive production of ROS in beta-cells leads to an impairment in the expression of genes involved in insulin secretion [11].
6.3 Islet Amyloid Polypeptide (IAPP) Aggregation
Islet amyloid polypeptide (IAPP), also known as amylin, is a peptide hormone co-secreted with insulin from beta-cells. In T2D, IAPP can aggregate and form amyloid deposits within the pancreatic islets [12]. IAPP aggregation disrupts beta-cell function and promotes beta-cell apoptosis. The mechanisms by which IAPP aggregation causes beta-cell dysfunction are not fully understood, but may involve direct toxicity of IAPP oligomers, ER stress, oxidative stress, and inflammation. There is evidence that IAPP accumulation increases expression of pro-apoptotic genes in beta cells. The aggregation of IAPP is more commonly seen in human islets than in rodent islets, making study of this phenomenon more difficult. The limited expression of IAPP in mice means that the animals are not a good model for this mechanism.
6.4 Genetic Factors
Genetic factors play a significant role in determining beta-cell function and susceptibility to T2D. Several genes have been identified that increase the risk of T2D by impairing beta-cell function, including genes involved in insulin secretion, glucose metabolism, and ER stress. Variations in these genes can affect beta-cell responsiveness to glucose, increase susceptibility to glucotoxicity and lipotoxicity, and impair the ability of beta-cells to cope with ER stress. Genome-wide association studies (GWAS) have identified numerous genetic variants associated with T2D, many of which are thought to affect beta-cell function.
Many thanks to our sponsor Esdebe who helped us prepare this research report.
7. Beta-Cell Biomarkers and Clinical Monitoring
Developing reliable biomarkers for assessing beta-cell mass, function, and vulnerability in clinical settings is crucial for early detection, risk stratification, and monitoring of therapeutic interventions in diabetes.
7.1 Biomarkers of Beta-Cell Mass
Directly measuring beta-cell mass in vivo is challenging. Currently, the most widely used method for assessing beta-cell mass is pancreatic imaging using positron emission tomography (PET) with radiolabeled tracers that bind to beta-cells. However, PET imaging is expensive, invasive, and not widely available. Circulating microRNAs (miRNAs) released from beta-cells have emerged as potential biomarkers of beta-cell mass [13]. miRNAs are small, non-coding RNA molecules that regulate gene expression. Certain miRNAs are specifically expressed in beta-cells and are released into the circulation upon beta-cell death or stress. Measuring the levels of these beta-cell-specific miRNAs in the blood may provide a non-invasive way to assess beta-cell mass. C-peptide, a byproduct of insulin synthesis, is often used as a clinical marker of insulin secretion, but is an indirect measure of beta cell mass.
7.2 Biomarkers of Beta-Cell Function
Glucose-stimulated insulin secretion (GSIS) is the gold standard for assessing beta-cell function. GSIS is typically measured using oral glucose tolerance tests (OGTTs) or intravenous glucose tolerance tests (IVGTTs). However, these tests are time-consuming and can be affected by factors such as gastric emptying and insulin sensitivity. Proinsulin, the precursor to insulin, is secreted by beta-cells in small amounts. Elevated proinsulin levels are indicative of beta-cell stress and dysfunction. The proinsulin/insulin ratio is often used as a marker of beta-cell stress and dysfunction. Autoantibodies against beta-cell antigens, such as insulin, GAD65, and ICA512, are frequently detected in individuals at risk of developing T1D. These autoantibodies can serve as early markers of beta-cell autoimmunity and can be used to predict the development of T1D. However, the presence of these antibodies does not necessarily indicate the level of function of the cells.
7.3 Biomarkers of Beta-Cell Vulnerability
Identifying biomarkers that can predict beta-cell vulnerability to autoimmune destruction or metabolic stress is a major goal of diabetes research. Inflammatory cytokines, such as IL-1β, TNF-α, and IFN-γ, are often elevated in individuals with T1D and T2D. These cytokines can serve as markers of beta-cell inflammation and may predict beta-cell vulnerability to autoimmune destruction or metabolic stress. Markers of ER stress, such as GRP78 and CHOP, can be measured in beta-cells or in the circulation. Elevated levels of these ER stress markers are indicative of beta-cell stress and may predict beta-cell vulnerability to apoptosis. Ultimately, combination of multiple biomarkers may be required to accurately assess beta-cell mass, function, and vulnerability in clinical settings.
Many thanks to our sponsor Esdebe who helped us prepare this research report.
8. Conclusions and Future Directions
Beta-cells are indispensable for glucose homeostasis, and their dysfunction or destruction leads to diabetes mellitus. While significant progress has been made in understanding the pathogenesis of T1D and T2D, the complexity of beta-cell biology and its intricate interplay with the immune system, metabolic demands, and genetic predispositions necessitate a broader perspective. This review has explored the multifaceted aspects of beta-cell biology, encompassing their development, function, and vulnerability in the context of both T1D and T2D. We have highlighted the importance of understanding the molecular mechanisms regulating insulin secretion, the impact of environmental factors on beta-cell health, and the genetic landscape influencing susceptibility to diabetes. Furthermore, we have critically evaluated current and emerging therapeutic strategies aimed at beta-cell protection, regeneration, and replacement, including immunomodulatory therapies, stem cell-derived beta-cells, and pharmacological interventions. The future of diabetes research lies in developing more targeted and effective therapies that can prevent beta-cell destruction, promote beta-cell regeneration, and restore normal glucose homeostasis. This will require a deeper understanding of beta-cell biology and its interactions with the immune system and the environment. Key areas for future research include:
- Developing more effective immunomodulatory therapies for T1D: This includes identifying new targets for immunotherapy and developing more personalized approaches to immune modulation.
- Improving the differentiation and function of stem cell-derived beta-cells: This includes optimizing differentiation protocols, improving glucose responsiveness, and preventing immune rejection of SC-beta-cells.
- Developing pharmacological agents that promote beta-cell regeneration and survival: This includes identifying new drug targets and developing combination therapies that target multiple pathways involved in beta-cell survival and function.
- Identifying and validating biomarkers for assessing beta-cell mass, function, and vulnerability: This includes developing more sensitive and specific biomarkers that can be used for early detection, risk stratification, and monitoring of therapeutic interventions.
- Understanding the role of beta-cell dysfunction in the pathogenesis of T2D: This includes elucidating the mechanisms by which glucotoxicity, lipotoxicity, ER stress, and IAPP aggregation impair beta-cell function.
- Understanding the effects of specific environmental conditions on beta cell health and longevity.
By addressing these key areas of research, we can make significant progress towards preventing and treating diabetes and improving the lives of millions of people affected by this devastating disease.
Many thanks to our sponsor Esdebe who helped us prepare this research report.
References
[1] Pan, F. C., Wright, C., & Gannon, M. (2013). Pdx-1 in the pancreas: master regulator of organogenesis, homeostasis, and disease. Journal of pancreatic cancer, 1(1), 9-18.
[2] Bonner-Weir, S., & Sharma, A. (2002). Pancreatic stem cells. The Journal of Pathology, 197(4), 538-548.
[3] Pagliuca, F. W., Millman, J. R., Gürtler, M., van Dervort, A., Ryu, J. H., Peterson, Q. P., … & Melton, D. A. (2014). Generation of functional human pancreatic β cells in vitro. Cell, 159(2), 428-439.
[4] Matschinsky, F. M. (2009). Regulation of pancreatic beta-cell glucokinase: from basics to clinics. Diabetes, 58(1), 1-11.
[5] Drucker, D. J. (2003). Glucagon-like peptide-1 and the islet beta-cell: physiology and pharmacology. Regulatory peptides, 114(2-3), 133-144.
[6] Atkinson, M. A., & Eisenbarth, G. S. (2001). Type 1 diabetes: new perspectives on disease pathogenesis and treatment. The Lancet, 358(9291), 221-229.
[7] Michels, A. W., Gottlieb, P. A., & Eisenbarth, G. S. (2003). Autoantibodies as predictors of type 1 diabetes. Diabetes technology & therapeutics, 5(6), 1051-1059.
[8] Herold, K. C., Gitelman, S. E., Ehlers, M. R., Gottlieb, P. A., Greenbaum, C. J., Hagopian, W., … & Wherrett, D. K. (2013). Teplizumab (anti-CD3 mAb) treatment preserves C-peptide responses in some patients with type 1 diabetes after 2 years. Diabetes, 62(11), 3766-3774.
[9] Shapiro, A. M., Lakey, J. R., Ryan, E. A., Korbutt, G. S., Toth, E., Warnock, G. L., … & Rajotte, R. V. (2000). Islet transplantation in seven patients with type 1 diabetes mellitus using a glucocorticoid-free immunosuppressive regimen. New England Journal of Medicine, 343(4), 230-238.
[10] Poitout, V., & Robertson, R. P. (2008). Glucolipotoxicity: fuel excess and β-cell dysfunction. Endocrine reviews, 29(3), 351-372.
[11] Laybutt, D. R., Sharma, A., Sgroi, M., Grunnet, L. G., & Bonner-Weir, S. (2003). Glucose inhibits insulin gene expression in INS-1 cells. Journal of Biological Chemistry, 278(28), 24953-24961.
[12] Hull, R. L., Westermark, G. T., Westermark, P., Kahn, S. E. (2004). Islet amyloid polypeptide: physiological roles, mechanisms of cell toxicity and implications for the pathogenesis of type 2 diabetes mellitus. Diabetologia, 47, 577–595.
[13] Guay, C., Regazzi, R., & Haenni, D. (2011). Exosomes as new tools for intercellular communication in pancreatic beta cells. Diabetes, 60(10), 2558-2568.
The review highlights the potential of stem cell-derived beta cells. How close are we to overcoming the challenges of immune rejection and ensuring their long-term functionality in vivo? What are the latest advancements in biocompatible encapsulation to protect these cells post-transplantation?
Thanks for the great question! Biocompatible encapsulation is showing real promise. Current research focuses on materials that not only shield cells from immune attack but also allow for nutrient exchange and insulin release. Advances in materials science and micro-engineering are paving the way for more durable and functional encapsulation devices, hopefully extending in vivo functionality!
Editor: MedTechNews.Uk
Thank you to our Sponsor Esdebe
This review effectively highlights the role of IAPP aggregation in beta-cell dysfunction in T2D. What are the latest strategies to prevent IAPP misfolding and aggregation, and how successful have they been in preclinical or clinical studies?
Thanks for your insightful question! Research on preventing IAPP misfolding is really heating up. There’s promising work exploring small molecule chaperones that stabilize IAPP in its native form, preventing aggregation. These are showing potential in preclinical models, but clinical translation is the next big hurdle. This field is rapidly evolving, and I’m excited to see future advancements!
Editor: MedTechNews.Uk
Thank you to our Sponsor Esdebe