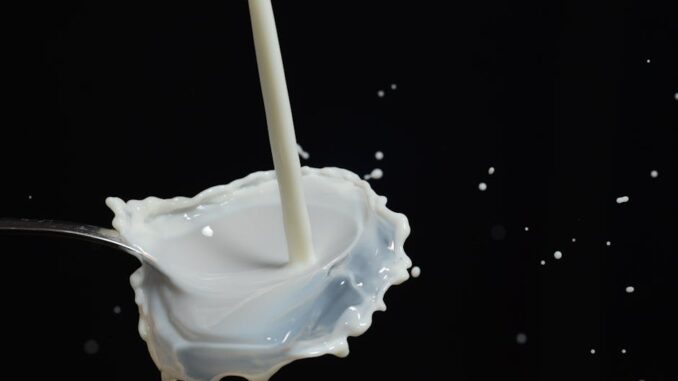
Abstract
Calcium, an essential element for life, plays a multifaceted role in biological systems, ranging from structural support in bone and teeth to critical signaling functions in cells. This review explores the diverse aspects of calcium, beginning with its fundamental biomineralization process in skeletal tissues, then delving into the pathological deposition of calcium in soft tissues, particularly within the vasculature. We examine the molecular mechanisms underlying both physiological and pathological calcification, including the roles of key regulatory proteins, signaling pathways, and cellular players. The prevalence and clinical significance of vascular calcification in various patient populations, such as those with chronic kidney disease, diabetes, and cardiovascular disease, are discussed. Finally, we critically evaluate current and emerging therapeutic strategies targeting calcium dysregulation, with a particular focus on novel approaches aimed at preventing and reversing vascular calcification. This review aims to provide a comprehensive overview of calcium’s dual role in health and disease, highlighting the complexity of calcium regulation and the potential for innovative therapies to address calcium-related disorders.
Many thanks to our sponsor Esdebe who helped us prepare this research report.
1. Introduction
Calcium (Ca2+) is a ubiquitous and vital element, constituting approximately 2% of the human body mass. Its significance extends beyond structural roles, serving as a critical intracellular and extracellular signaling molecule involved in a vast array of physiological processes. These include muscle contraction, nerve impulse transmission, blood clotting, hormone secretion, and enzyme regulation [1]. The intricate balance of calcium homeostasis is essential for maintaining cellular function and overall health. Disruption of this balance, whether through genetic defects, dietary imbalances, or underlying disease states, can lead to a spectrum of disorders characterized by either calcium deficiency or pathological calcium deposition.
The primary reservoir of calcium within the body resides in the skeletal system, where it exists as hydroxyapatite (Ca10(PO4)6(OH)2), a crystalline mineral that provides structural rigidity to bones and teeth [2]. The process of biomineralization, whereby calcium and phosphate ions are precisely orchestrated to form hydroxyapatite crystals, is tightly regulated by a complex interplay of cells, matrix proteins, and systemic hormones. However, under certain conditions, calcium can be deposited in soft tissues, a process known as ectopic calcification. This pathological calcification can occur in various organs, including the kidneys, heart valves, and vasculature, contributing to significant morbidity and mortality.
This review aims to provide a comprehensive overview of calcium, encompassing its physiological role in bone biomineralization and its pathological deposition in soft tissues, with a particular focus on vascular calcification. We will explore the molecular mechanisms underlying these processes, examine the clinical implications of calcium dysregulation, and discuss current and emerging therapeutic strategies targeting calcium-related disorders. The focus will be on understanding the nuanced duality of calcium in biological systems.
Many thanks to our sponsor Esdebe who helped us prepare this research report.
2. Calcium Homeostasis and Biomineralization
The maintenance of calcium homeostasis is crucial for proper physiological function. This intricate process is primarily regulated by the interplay of three key hormones: parathyroid hormone (PTH), vitamin D, and calcitonin [3]. PTH, secreted by the parathyroid glands in response to low serum calcium levels, stimulates bone resorption, increases renal calcium reabsorption, and promotes the synthesis of active vitamin D (calcitriol) in the kidneys. Calcitriol, in turn, enhances intestinal calcium absorption. Calcitonin, secreted by the thyroid gland in response to high serum calcium levels, inhibits bone resorption and promotes renal calcium excretion, although its role in calcium homeostasis is generally considered less significant than that of PTH and vitamin D [4].
The process of bone biomineralization is a highly controlled and complex phenomenon involving osteoblasts, specialized bone-forming cells. Osteoblasts secrete a collagen-rich extracellular matrix (ECM) that serves as a scaffold for mineral deposition. This matrix contains various non-collagenous proteins, including osteocalcin, osteopontin, and bone sialoprotein, which play critical roles in regulating the nucleation and growth of hydroxyapatite crystals [5]. The precise mechanisms governing biomineralization are not fully understood, but it is believed that matrix vesicles, small membrane-bound structures secreted by osteoblasts, initiate the process by concentrating calcium and phosphate ions within their lumen, leading to the formation of initial mineral deposits. These deposits then propagate and coalesce, eventually forming the mature hydroxyapatite crystals that characterize bone tissue [6].
Genetic factors also play a significant role in bone mineral density and susceptibility to osteoporosis. Polymorphisms in genes encoding vitamin D receptors, collagen type I, and other bone-related proteins have been associated with variations in bone mass and fracture risk [7]. Furthermore, mechanical loading is a crucial determinant of bone remodeling, with increased mechanical stress stimulating bone formation and decreased stress leading to bone resorption [8]. This mechanosensitivity allows bone to adapt its structure and density in response to changing physical demands. Disruption to any of these processes will result in either too little or too much mineralization of the bone tissues.
Many thanks to our sponsor Esdebe who helped us prepare this research report.
3. Vascular Calcification: Mechanisms and Pathophysiology
Vascular calcification, the abnormal deposition of calcium phosphate minerals in the arterial wall, is a hallmark of several cardiovascular diseases, including atherosclerosis, hypertension, and chronic kidney disease (CKD) [9]. It is no longer considered a passive degenerative process but rather an actively regulated phenomenon resembling bone formation, involving complex cellular and molecular mechanisms.
There are two main types of vascular calcification: intimal and medial. Intimal calcification occurs in the intima, the innermost layer of the artery, and is typically associated with atherosclerotic plaques. It is characterized by the formation of microcalcifications within the lipid core of the plaque, which can destabilize the plaque and increase the risk of rupture, leading to acute thrombotic events such as myocardial infarction and stroke [10]. Medial calcification, also known as Mönckeberg’s sclerosis, occurs in the media, the middle layer of the artery, and is characterized by the deposition of calcium phosphate minerals in the smooth muscle cell layer. Medial calcification is particularly prevalent in patients with CKD and diabetes and contributes to arterial stiffness and increased pulse pressure [11].
The cellular and molecular mechanisms underlying vascular calcification are complex and involve a variety of factors, including: (a) Phenotypic modulation of vascular smooth muscle cells (VSMCs): VSMCs, the predominant cell type in the arterial media, can undergo phenotypic switching from a contractile to an osteoblast-like phenotype, characterized by the expression of bone-related proteins such as osteocalcin, osteopontin, and alkaline phosphatase [12]. This phenotypic transition is driven by various factors, including inflammatory cytokines, oxidative stress, and elevated phosphate levels. (b) Matrix vesicle release: Similar to bone biomineralization, matrix vesicles released by VSMCs can initiate calcification by concentrating calcium and phosphate ions within their lumen [13]. (c) Inhibition of calcification inhibitors: Several endogenous inhibitors of calcification, such as matrix Gla protein (MGP) and fetuin-A, play a crucial role in preventing vascular calcification. MGP is a vitamin K-dependent protein that binds to calcium phosphate crystals and inhibits their growth [14]. Fetuin-A is a circulating glycoprotein that binds to calcium and phosphate ions, forming colloidal particles that prevent their precipitation [15]. Deficiencies in these inhibitors, either due to genetic defects or acquired conditions, can promote vascular calcification. (d) Inflammation: Chronic inflammation plays a critical role in vascular calcification. Inflammatory cytokines, such as interleukin-6 (IL-6) and tumor necrosis factor-alpha (TNF-α), can promote VSMC phenotypic switching and induce the expression of bone-related proteins [16]. (e) Phosphate dysregulation: Elevated serum phosphate levels are a major driver of vascular calcification, particularly in patients with CKD. Hyperphosphatemia directly stimulates VSMC calcification and inhibits the expression of calcification inhibitors [17].
The interplay of these factors creates a microenvironment conducive to calcium phosphate deposition within the arterial wall, leading to the development of vascular calcification. The specific type and location of calcification depend on the underlying etiology and the relative contribution of different factors. Therefore targeting the underlying risk factors is vital.
Many thanks to our sponsor Esdebe who helped us prepare this research report.
4. Prevalence and Clinical Significance of Vascular Calcification
Vascular calcification is highly prevalent in various patient populations, including those with CKD, diabetes, cardiovascular disease, and aging individuals [18]. The prevalence and severity of vascular calcification increase with age, reflecting the cumulative effects of chronic exposure to risk factors such as hypertension, hyperlipidemia, and inflammation. In patients with CKD, vascular calcification is particularly severe and accelerated due to impaired phosphate excretion, secondary hyperparathyroidism, and reduced levels of calcification inhibitors [19].
The clinical significance of vascular calcification is multifaceted and depends on the location and extent of calcification. Intimal calcification in atherosclerotic plaques increases the risk of plaque rupture and thrombotic events, leading to myocardial infarction, stroke, and peripheral artery disease [20]. Medial calcification, on the other hand, contributes to arterial stiffness and increased pulse pressure, leading to left ventricular hypertrophy, heart failure, and increased cardiovascular mortality [21]. Vascular calcification can also impair the function of prosthetic heart valves and vascular grafts, leading to valve stenosis, graft failure, and the need for re-intervention [22].
Several studies have demonstrated a strong association between vascular calcification and adverse cardiovascular outcomes. For example, the presence and severity of coronary artery calcification, as assessed by computed tomography (CT) scanning, is a powerful predictor of future cardiovascular events, independent of traditional risk factors such as age, gender, and cholesterol levels [23]. Similarly, the presence of aortic valve calcification is associated with increased risk of aortic stenosis, heart failure, and death [24].
Beyond cardiovascular events, vascular calcification has also been linked to other adverse outcomes, such as dementia and cognitive decline. Arterial stiffness and reduced cerebral blood flow, resulting from vascular calcification, can contribute to neuronal damage and impaired cognitive function [25]. Furthermore, vascular calcification in the lower extremities can lead to peripheral artery disease, causing leg pain, impaired mobility, and increased risk of amputation [26].
The widespread prevalence and significant clinical consequences of vascular calcification highlight the need for effective strategies to prevent and treat this condition. Therefore early diagnosis and preventative measures is essential for at risk patients.
Many thanks to our sponsor Esdebe who helped us prepare this research report.
5. Diagnostic Modalities for Vascular Calcification
Several imaging techniques are available for detecting and quantifying vascular calcification. These techniques vary in their sensitivity, specificity, and invasiveness, and the choice of imaging modality depends on the clinical context and the specific vascular bed being evaluated.
- Plain Radiography: Plain radiographs can detect macroscopic calcification in arteries, particularly in the aorta and peripheral arteries. However, plain radiography is relatively insensitive and cannot detect early or subtle calcification [27].
- Computed Tomography (CT): CT scanning is a highly sensitive and accurate method for detecting and quantifying vascular calcification. Electron beam CT (EBCT) and multidetector CT (MDCT) are commonly used to assess coronary artery calcification (CAC). The Agatston score, derived from CT images, is a widely used measure of CAC burden and is a strong predictor of cardiovascular events [28].
- Ultrasound: Ultrasound imaging can detect calcification in carotid arteries and peripheral arteries. Carotid intima-media thickness (CIMT) measurement, a non-invasive ultrasound technique, is a surrogate marker of atherosclerosis and is associated with vascular calcification [29].
- Magnetic Resonance Imaging (MRI): MRI can detect vascular calcification, but its sensitivity and specificity are lower than those of CT. MRI is particularly useful for assessing aortic calcification and for differentiating between intimal and medial calcification [30].
- Nuclear Imaging: Bone scintigraphy, using technetium-99m-labeled bisphosphonates, can detect areas of active calcification in arteries. However, bone scintigraphy is not specific for vascular calcification and can be affected by other bone-related conditions [31].
In addition to imaging techniques, several biomarkers have been proposed as potential indicators of vascular calcification. These include: (a) Osteoprotegerin (OPG): OPG is a decoy receptor for receptor activator of nuclear factor kappa-B ligand (RANKL), a key regulator of bone resorption. Elevated levels of OPG have been associated with vascular calcification and increased cardiovascular risk [32]. (b) Matrix Gla protein (MGP): Uncarboxylated MGP (ucMGP) is a marker of vitamin K deficiency and is associated with increased vascular calcification [33]. (c) Fetuin-A: Low levels of fetuin-A have been associated with increased vascular calcification and cardiovascular mortality [34].
The combination of imaging techniques and biomarkers can provide a comprehensive assessment of vascular calcification and help to identify individuals at high risk for cardiovascular events. However, further research is needed to validate the clinical utility of these biomarkers and to develop more sensitive and specific diagnostic tools.
Many thanks to our sponsor Esdebe who helped us prepare this research report.
6. Therapeutic Strategies Targeting Vascular Calcification
The management of vascular calcification is challenging, and there are currently no approved therapies specifically designed to reverse or prevent its progression. However, several strategies have been proposed to mitigate the risk factors associated with vascular calcification and to slow its progression.
- Phosphate Control: In patients with CKD, phosphate control is a cornerstone of therapy. Dietary phosphate restriction, phosphate binders (e.g., calcium-based binders, sevelamer, lanthanum carbonate), and dialysis are used to lower serum phosphate levels and reduce the risk of vascular calcification [35].
- Vitamin K Supplementation: Vitamin K is essential for the carboxylation of MGP, a key inhibitor of vascular calcification. Vitamin K deficiency is common in patients with CKD and diabetes, and vitamin K supplementation has been shown to reduce ucMGP levels and potentially slow the progression of vascular calcification [36]. However, the optimal dose and form of vitamin K (K1 or K2) are still under investigation.
- Statins: Statins, HMG-CoA reductase inhibitors, are widely used to lower cholesterol levels and reduce the risk of cardiovascular events. Statins have also been shown to have pleiotropic effects, including anti-inflammatory and anti-calcification properties [37]. Some studies have suggested that statins can slow the progression of coronary artery calcification, but the evidence is not conclusive.
- Bisphosphonates: Bisphosphonates, inhibitors of bone resorption, have been investigated as potential therapies for vascular calcification. Some studies have shown that bisphosphonates can reduce vascular calcification in animal models, but clinical trials in humans have yielded mixed results [38]. The potential risks and benefits of bisphosphonates in patients with vascular calcification need to be carefully evaluated.
- Calcimimetics: Calcimimetics, such as cinacalcet, are drugs that increase the sensitivity of the calcium-sensing receptor (CaSR) on parathyroid cells, leading to a reduction in PTH secretion. Calcimimetics are used to treat secondary hyperparathyroidism in patients with CKD and have been shown to reduce vascular calcification in some studies [39].
In addition to these established therapies, several novel approaches are being investigated for the prevention and treatment of vascular calcification. These include: (a) Inhibition of bone morphogenetic proteins (BMPs): BMPs are signaling molecules that play a key role in bone formation and have been implicated in vascular calcification. Inhibitors of BMP signaling, such as noggin and gremlin, have shown promise in reducing vascular calcification in animal models [40]. (b) Gene therapy: Gene therapy approaches aimed at increasing the expression of calcification inhibitors, such as MGP and fetuin-A, are being developed for the treatment of vascular calcification [41]. (c) Nanoparticles: Nanoparticles loaded with calcification inhibitors or drugs that promote calcium phosphate dissolution are being investigated as targeted therapies for vascular calcification [42].
The development of effective therapies for vascular calcification remains a major challenge. A combination of lifestyle modifications, risk factor management, and targeted therapies may be necessary to prevent and reverse this condition. Further research is needed to identify novel therapeutic targets and to develop safe and effective therapies for vascular calcification.
Many thanks to our sponsor Esdebe who helped us prepare this research report.
7. Conclusion
Calcium plays a crucial role in human physiology, serving as both a structural component of bone and a vital signaling molecule. However, dysregulation of calcium homeostasis can lead to pathological calcification in soft tissues, particularly the vasculature. Vascular calcification is a complex process involving multiple cellular and molecular mechanisms, and it is highly prevalent in patients with CKD, diabetes, and cardiovascular disease.
The clinical significance of vascular calcification is multifaceted, contributing to increased arterial stiffness, plaque instability, and adverse cardiovascular outcomes. Diagnostic modalities such as CT scanning and ultrasound imaging are used to detect and quantify vascular calcification, while biomarkers such as OPG, MGP, and fetuin-A may provide additional insights into the pathogenesis of this condition.
The management of vascular calcification is challenging, and there are currently no approved therapies specifically designed to reverse or prevent its progression. However, strategies such as phosphate control, vitamin K supplementation, and statin therapy can help to mitigate the risk factors associated with vascular calcification and to slow its progression. Novel approaches, such as inhibition of BMP signaling, gene therapy, and nanoparticles, are being investigated as potential therapies for vascular calcification.
Future research should focus on elucidating the precise mechanisms underlying vascular calcification, identifying novel therapeutic targets, and developing safe and effective therapies to prevent and reverse this condition. A deeper understanding of the complex interplay between calcium homeostasis, vascular biology, and systemic disease is essential for improving the outcomes of patients with vascular calcification.
Many thanks to our sponsor Esdebe who helped us prepare this research report.
References
[1] Berridge, M. J., Bootman, M. D., & Roderick, H. L. (2003). Calcium signalling: dynamics, homeostasis and remodelling. Nature Reviews Molecular Cell Biology, 4(7), 517-529.
[2] Glimcher, M. J. (2006). Bone: always remodeling, age-related osteoporosis results from adaptive failure of remodeling. Calcified Tissue International, 79(3), 145-149.
[3] Brown, E. M. (2003). Extracellular Ca2+ sensing, regulation of parathyroid cell function, and role in pathophysiology. American Journal of Physiology-Renal Physiology, 284(6), F1179-F1189.
[4] Austin, L. A., & Heath III, H. (1981). Calcitonin physiology and pathophysiology. New England Journal of Medicine, 304(5), 269-278.
[5] Lian, J. B., Stein, G. S., Gerstenfeld, L. C., & Owen, T. A. (1993). Anabolic agents for bone: principles and therapeutic potential. Endocrine Reviews, 14(4), 491-511.
[6] Anderson, H. C. (1995). Molecular biology of matrix vesicles. Clinical Orthopaedics and Related Research, (314), 266-280.
[7] Ralston, S. H. (2010). Genetic determinants of bone mass. Arthritis Research & Therapy, 12(5), 224.
[8] Frost, H. M. (1997). Bone “mass” and the “mechanostat”: a proposal. Anatomical Record, 247(1), 1-10.
[9] Demer, L. L., & Tintut, Y. (2008). Vascular calcification: pathobiology of a multifaceted disease. Circulation, 117(22), 2938-2948.
[10] Vasan, R. S. (2006). Biomarkers of cardiovascular disease: molecular basis and practical considerations. Circulation, 113(19), 2335-2362.
[11] London, G. M., Marchais, S. J., Guerin, A. P., Metivier, F., de Vernejoul, M. C., Aldigier, J. C., … & Sassier, P. (1990). Arterial media calcification in end-stage renal disease: impact on all-cause and cardiovascular mortality. Nephrology Dialysis Transplantation, 5(7), 561-572.
[12] Shanahan, C. M. (2007). Mechanisms of vascular calcification. Journal of the American Society of Nephrology, 18(10), 2931-2939.
[13] Kapustin, A. N., Chatrou, M. L. L., Drozdov, I., Zheng, Y., Davidson, S. M., Soong, D., … & Davies, P. F. (2015). Vascular smooth muscle cell–derived matrix vesicles: an important mechanism of vascular calcification. Circulation Research, 116(7), 1095-1104.
[14] Luo, G., Ducy, P., McKee, M. D., Pinero, G. J., Loyer, E., Behringer, R. R., & Karsenty, G. (1997). Spontaneous calcification of arteries and cartilage in mice lacking matrix Gla protein. Nature, 386(6620), 78-81.
[15] Heiss, A., Duewell, P., Freiberger, S. N., Görg, C., Schöttker, B., Raffael, S., … & März, W. (2010). Fetuin-A is independently associated with all-cause and cardiovascular mortality in a large German prospective cohort. Arteriosclerosis, Thrombosis, and Vascular Biology, 30(7), 1524-1530.
[16] Libby, P. (2002). Inflammation in atherosclerosis. Nature, 420(6917), 868-874.
[17] Jono, S., Nishizawa, Y., Shioi, A., Morii, H. (1998). Vascular calcification in chronic kidney disease. American Journal of Kidney Diseases, 32(6 Suppl 4), S4-S10.
[18] Leopold, J. A. (2015). Vascular calcification: mechanisms and clinical implications. Circulation, 131(8), 725-744.
[19] Goodman, W. G., Goldin, J., Kuizon, B. D., Yoon, C., Gales, B., Sider, D., … & Salusky, I. B. (2000). Coronary-artery calcification in young adults with end-stage renal disease who are undergoing dialysis. New England Journal of Medicine, 342(20), 1478-1483.
[20] Nakashima, Y., Fujii, H., Kobayashi, M., Wight, T. N., & Sueishi, K. (2007). Localization of chondroitin sulfate proteoglycans in human coronary arteries and their functional roles in intimal thickening and calcification. Arteriosclerosis, Thrombosis, and Vascular Biology, 27(1), 166-173.
[21] Laurent, S., Boutouyrie, P., Asmar, R., Gautier, I., Laloux, B., Guize, L., … & Safar, M. E. (2001). Aortic stiffness is an independent predictor of all-cause and cardiovascular mortality in hypertensive patients. Hypertension, 37(5), 1236-1241.
[22] Schoen, F. J., Levy, R. J., Piehler, J. M., Didisheim, P., & Clark, R. E. (1987). Pathobiology of calcific valve disease: initiation, propagation, and prevention. Journal of Heart Valve Disease, 17(1), 1-17.
[23] Greenland, P., LaBree, L., Azen, S. P., Doherty, T. M., Detrano, R. C. (2004). Coronary artery calcium score combined with Framingham risk assessment for risk prediction in asymptomatic individuals. JAMA, 291(2), 210-217.
[24] Otto, C. M., Lind, B. K., Kitzman, D. W., Gersh, B. J., Siscovick, D. S., & Alderman, E. L. (1999). Association of aortic-valve sclerosis with cardiovascular mortality and morbidity in the elderly. New England Journal of Medicine, 341(3), 142-147.
[25] Tzourio, C. (2007). Epidemiology of cognitive decline and dementia. Dialogues in Clinical Neuroscience, 9(1), 11-21.
[26] Hirsch, A. T., Haskal, Z. J., Hertzer, N. R., Bakal, C. W., Creager, M. A., Halperin, J. L., … & Treat-Jacobson, D. (2006). ACC/AHA 2005 Practice Guidelines for the management of patients with peripheral arterial disease (lower extremity, renal, mesenteric, and abdominal aortic) a collaborative report from the American Association for Vascular Surgery/Society for Vascular Surgery, Society for Cardiovascular Angiography and Interventions, Society for Vascular Medicine and Biology, Society of Interventional Radiology, and the ACC/AHA Task Force on Practice Guidelines. Journal of the American College of Cardiology, 47(6), 1239-1312.
[27] Braun, J., Oldendorf, M., Moshage, W., Heidler, R., Zeitler, E., & Luft, F. C. (1996). Electron beam computed tomography in the evaluation of cardiac calcification. American Heart Journal, 131(4), 810-817.
[28] Agatston, A. S., Janowitz, W. R., Hildner, F. J., Zusmer, O., Viamonte Jr, M., & Detrano, R. (1990). Quantification of coronary artery calcium using ultrafast computed tomography. Journal of the American College of Cardiology, 15(4), 827-832.
[29] O’Leary, D. H., Polak, J. F., Kronmal, R. A., Manolio, T. A., Burke, G. L., & Wolfson, S. K. (1999). Carotid-artery intima and media thickness as a risk factor for myocardial infarction and stroke in older adults. New England Journal of Medicine, 340(1), 14-22.
[30] Tatsugami, F., Higaki, T., Sakatani, T., Kitagawa, Y., Tamura, S., Awai, K., … & Yamashita, Y. (2004). Non-contrast-enhanced MR imaging to assess arterial calcification: Comparison with multi-detector row computed tomography. European Radiology, 14(12), 2188-2195.
[31] Yardley, J. E., Clunie, G., & Bird, N. J. (2008). Cardiovascular uptake of bone seeking agents and its implications. Nuclear Medicine Communications, 29(12), 1039-1044.
[32] Kiechl, S., Lorenz, E., Reindl, M., Wiedermann, F., Oberhollenzer, M., Bonora, E., … & Willeit, J. (2003). Osteoprotegerin is a risk factor for cardiovascular disease and mortality in the general population. Circulation, 107(25), 2920-2926.
[33] Schurgers, L. J., Vermeer, C. (2000). Determination of the coagulation factor-binding capacity of vitamin K-dependent carboxylase. Journal of Thrombosis and Haemostasis, 83(6), 906-911.
[34] Ix, J. H., Shlipak, M. G., Wassel, C. L., Whooley, M. A., Harrington, R., Hoefner, D., … & Kestenbaum, B. (2008). Association of fetuin-A with mortality, cardiovascular events, and incident diabetes in persons with coronary heart disease: data from the Heart and Soul Study. Circulation, 117(24), 2914-2922.
[35] Block, G. A., Hulbert-Shearon, T. E., Levin, N. W., Port, F. K. (2005). Association of serum phosphorus and calcium × phosphate product with mortality risk in chronic hemodialysis patients: a national study. American Journal of Kidney Diseases, 46(2), 212-220.
[36] Geleijnse, J. M., Vermeer, C., Grobbee, D. E., Schurgers, L. J., Knapen, M. H. J., Van Der Meer, I. M., … & Witteman, J. C. M. (2004). Dietary intake of menaquinone is associated with a reduced risk of coronary heart disease: the Rotterdam Study. The Journal of Nutrition, 134(11), 3100-3105.
[37] Athyros, V. G., Mikhailidis, D. P., Papageorgiou, A. A., Symeonidis, A. N., Peiou, C., Demitriadis, D. S., … & Didangelos, T. P. (2002). Statins and inflammation in coronary heart disease. American Heart Journal, 143(6), 953-963.
[38] Price, P. A., Faus, S. A., Williamson, M. K. (2001). Warfarin-induced arteriopathy is a generalized process dependent on vitamin K epoxide reductase. The American Journal of Pathology, 158(2), 561-567.
[39] Block, G. A., Klassen, P. S., Snow, A. L., Chertow, G. M., DiMarco, L., Kumar, J., … & Lazarus, J. M. (2004). Cinacalcet HCl for secondary hyperparathyroidism in patients receiving hemodialysis. Kidney International, 66(3), 1132-1140.
[40] Wang, H., Wetmore, J. B., Zhang, S., Shah, D. R., Hum, J., Misra, M., … & Hoofnagle, A. N. (2010). Circulating bone morphogenetic protein 7 levels are inversely associated with mortality in hemodialysis patients. Journal of the American Society of Nephrology, 21(1), 155-162.
[41] Dhore, C. R., Cleutjens, J. P., Esnard, F. E., Schurgers, L. J., De Mey, J. G. R., & Vermeer, C. (2001). Vitamin K-dependent γ-carboxylation is required for inhibition of arterial calcification. Journal of Thrombosis and Haemostasis, 85(3), 520-525.
[42] Villa, C. H., Anselmo, A. C., Mitragotri, S. (2010). Nanoparticles in vaccine delivery. Chemical Engineering Journal, 162(1), 2-22.
The discussion on therapeutic strategies is crucial. How might personalized medicine approaches, considering individual genetic predispositions and disease stages, optimize the effectiveness of these therapies in managing vascular calcification?
That’s a fantastic point! Personalizing treatment based on genetics and disease stage could significantly improve outcomes. Imagine tailoring phosphate control or vitamin K supplementation based on an individual’s specific risk profile for vascular calcification. It opens exciting avenues for more effective therapies.
Editor: MedTechNews.Uk
Thank you to our Sponsor Esdebe
This is a great overview of the therapeutic landscape. Considering the role of inflammation, could interventions targeting specific inflammatory pathways offer a complementary approach to managing vascular calcification, potentially improving outcomes alongside existing strategies?
Thanks for the insightful comment! You’re absolutely right, exploring interventions targeting inflammatory pathways could be a game-changer. Perhaps research focusing on specific cytokines or immune cell interactions within the vasculature might reveal new therapeutic targets. It opens up exciting new avenues for vascular calcification management!
Editor: MedTechNews.Uk
Thank you to our Sponsor Esdebe