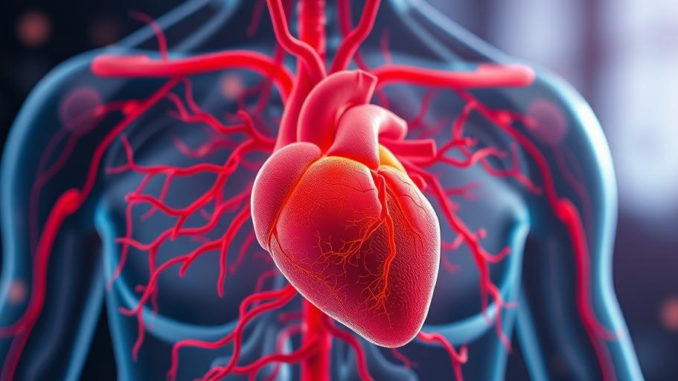
Abstract
Cancer therapy, while crucial for improving survival rates, is often associated with significant cardiovascular complications. This review comprehensively examines the mechanisms underlying cardiotoxicity induced by various cancer treatments, including chemotherapy, targeted therapies, radiation therapy, and immunotherapy. We delve into the specific cardiovascular risks associated with these modalities, focusing on mechanisms such as direct myocardial damage, endothelial dysfunction, inflammation, thrombosis, and metabolic derangements. Furthermore, we explore the importance of early and ongoing cardiovascular monitoring in patients undergoing cancer treatment, highlighting current guidelines and emerging imaging techniques for risk stratification and detection of subclinical cardiac dysfunction. Finally, we discuss potential cardioprotective strategies, including lifestyle modifications, pharmacological interventions (e.g., ACE inhibitors, beta-blockers, statins, and novel agents), and innovative approaches such as stem cell therapy and remote ischemic conditioning. This review aims to provide clinicians and researchers with an updated understanding of the cardiotoxic effects of cancer therapies and to guide the development of personalized strategies to minimize cardiovascular complications and improve long-term outcomes for cancer survivors.
Many thanks to our sponsor Esdebe who helped us prepare this research report.
1. Introduction
Cancer is a leading cause of morbidity and mortality worldwide. Advances in cancer diagnosis and treatment have led to significant improvements in survival rates. However, many cancer therapies, including chemotherapy, targeted therapies, radiation therapy, and immunotherapy, can cause significant cardiovascular complications. These complications, collectively known as cancer therapy-related cardiac dysfunction (CTRCD), can manifest as heart failure, arrhythmias, coronary artery disease, hypertension, thromboembolic events, and pericardial disease. The incidence of CTRCD varies depending on the type of cancer therapy, the dose and duration of treatment, patient-related risk factors (e.g., pre-existing cardiovascular disease, age, genetic predisposition), and the definition used to diagnose cardiotoxicity. Given the increasing number of cancer survivors and their longer life expectancy, the long-term cardiovascular consequences of cancer therapy are becoming a growing concern. There is a pressing need to understand the mechanisms underlying CTRCD, to develop strategies for early detection and risk stratification, and to implement effective cardioprotective interventions.
Many thanks to our sponsor Esdebe who helped us prepare this research report.
2. Mechanisms of Cancer Therapy-Related Cardiac Dysfunction
Cancer therapies can induce cardiac damage through various mechanisms, including:
2.1 Direct Myocardial Damage
Several chemotherapeutic agents, such as anthracyclines (e.g., doxorubicin, epirubicin) and tyrosine kinase inhibitors (TKIs), can directly damage cardiomyocytes. Anthracyclines, for instance, generate reactive oxygen species (ROS) that cause oxidative stress, mitochondrial dysfunction, and DNA damage in cardiomyocytes. These agents can also interfere with iron metabolism, leading to iron accumulation and further oxidative stress. TKIs, on the other hand, can inhibit various signaling pathways essential for cardiomyocyte survival and function, leading to apoptosis and necrosis. The precise mechanisms of TKI-induced cardiotoxicity are diverse and dependent on the specific drug and its target. For example, some TKIs inhibit vascular endothelial growth factor (VEGF) signaling, which can impair angiogenesis and lead to myocardial ischemia.
2.2 Endothelial Dysfunction
The endothelium plays a crucial role in regulating vascular tone, permeability, and coagulation. Several cancer therapies can disrupt endothelial function, leading to vasoconstriction, inflammation, and thrombosis. Alkylating agents (e.g., cyclophosphamide) and radiation therapy, in particular, can damage endothelial cells and impair their ability to produce nitric oxide (NO), a potent vasodilator. Endothelial dysfunction can contribute to hypertension, coronary artery disease, and thromboembolic events.
2.3 Inflammation
Inflammation is a key mediator of cardiac damage in CTRCD. Chemotherapy and radiation therapy can trigger the release of inflammatory cytokines, such as tumor necrosis factor-alpha (TNF-α) and interleukin-1β (IL-1β), which can directly damage cardiomyocytes and promote endothelial dysfunction. Immune checkpoint inhibitors (ICIs), such as anti-PD-1 and anti-CTLA-4 antibodies, can also induce immune-mediated myocarditis, a severe form of cardiac inflammation that can lead to heart failure and sudden cardiac death.
2.4 Thrombosis
Cancer and its treatment can increase the risk of thrombosis, both arterial and venous. Chemotherapy can activate the coagulation cascade and impair the fibrinolytic system, leading to a prothrombotic state. Certain cancer therapies, such as bevacizumab (an anti-VEGF antibody), are associated with an increased risk of arterial thromboembolic events, including myocardial infarction and stroke. Thrombotic microangiopathy (TMA) is another thrombotic complication associated with some cancer therapies, including gemcitabine and TKIs. TMA is characterized by endothelial damage, platelet activation, and microvascular thrombosis, which can lead to organ dysfunction.
2.5 Metabolic Derangements
Cancer therapies can also affect cardiac metabolism, leading to metabolic derangements that contribute to cardiac dysfunction. Anthracyclines, for example, can disrupt mitochondrial function and impair fatty acid oxidation, the primary energy source for the heart. Targeted therapies, such as TKIs, can also affect glucose metabolism and insulin sensitivity, potentially leading to metabolic syndrome and increased cardiovascular risk. Furthermore, cancer therapies can cause electrolyte imbalances, such as hypokalemia and hypomagnesemia, which can increase the risk of arrhythmias.
Many thanks to our sponsor Esdebe who helped us prepare this research report.
3. Cardiovascular Risks Associated with Specific Cancer Therapies
3.1 Chemotherapy
-
Anthracyclines: Doxorubicin, epirubicin, and daunorubicin are associated with a dose-dependent risk of cardiotoxicity, ranging from asymptomatic decreases in left ventricular ejection fraction (LVEF) to overt heart failure. The risk is higher in patients with pre-existing cardiovascular disease, older age, and concomitant use of other cardiotoxic agents. Mechanisms include ROS production, mitochondrial dysfunction, and DNA damage. The use of liposomal anthracyclines and continuous infusion regimens may reduce the risk of cardiotoxicity.
-
Alkylating Agents: Cyclophosphamide and ifosfamide can cause cardiotoxicity, particularly at high doses. Mechanisms include endothelial damage, inflammation, and direct myocardial toxicity. High-dose cyclophosphamide can also cause hemorrhagic myocarditis.
-
Platinum-Based Agents: Cisplatin and carboplatin can cause cardiotoxicity, including arrhythmias, myocardial ischemia, and hypertension. Mechanisms include endothelial dysfunction, inflammation, and oxidative stress.
-
Taxanes: Paclitaxel and docetaxel can cause bradycardia, arrhythmias, and myocardial ischemia. Mechanisms include direct myocardial toxicity and endothelial dysfunction.
-
5-Fluorouracil (5-FU): 5-FU can cause coronary artery vasospasm and myocardial ischemia. Mechanisms include endothelial dysfunction and increased production of endothelin-1, a potent vasoconstrictor.
3.2 Targeted Therapies
-
Tyrosine Kinase Inhibitors (TKIs): TKIs, such as imatinib, sunitinib, and sorafenib, can cause cardiotoxicity, including heart failure, hypertension, and arrhythmias. Mechanisms include inhibition of VEGF signaling, direct myocardial toxicity, and metabolic derangements. The specific cardiotoxic effects vary depending on the TKI and its target.
-
HER2-Targeted Therapies: Trastuzumab and pertuzumab can cause cardiotoxicity, particularly in combination with anthracyclines. Mechanisms include direct myocardial toxicity and inhibition of HER2 signaling, which is important for cardiomyocyte survival.
-
Proteasome Inhibitors: Bortezomib and carfilzomib can cause heart failure, hypertension, and arrhythmias. Mechanisms include direct myocardial toxicity and inflammation.
3.3 Radiation Therapy
Radiation therapy to the chest can cause cardiotoxicity, including pericardial disease, coronary artery disease, valvular heart disease, and cardiomyopathy. The risk of cardiotoxicity is higher with higher doses of radiation, larger radiation fields, and concurrent chemotherapy. Mechanisms include endothelial damage, inflammation, and fibrosis.
3.4 Immunotherapy
-
Immune Checkpoint Inhibitors (ICIs): ICIs, such as anti-PD-1 and anti-CTLA-4 antibodies, can cause immune-mediated myocarditis, a severe form of cardiac inflammation that can lead to heart failure and sudden cardiac death. The incidence of ICI-induced myocarditis is rare but can be fatal. Early diagnosis and treatment with immunosuppressive therapy are crucial.
-
CAR-T Cell Therapy: Chimeric antigen receptor (CAR) T-cell therapy can cause cytokine release syndrome (CRS), which can lead to cardiac dysfunction, including hypotension, arrhythmias, and heart failure. CRS is mediated by the release of inflammatory cytokines, such as IL-6, IL-1, and TNF-α.
Many thanks to our sponsor Esdebe who helped us prepare this research report.
4. Cardiovascular Monitoring During and After Cancer Therapy
Early and ongoing cardiovascular monitoring is essential for detecting and managing CTRCD. Current guidelines recommend baseline cardiovascular assessment before initiation of cancer therapy, followed by periodic monitoring during and after treatment. The frequency and type of monitoring depend on the specific cancer therapy, patient-related risk factors, and the presence of symptoms.
4.1 Baseline Assessment
Baseline assessment should include a thorough medical history, physical examination, electrocardiogram (ECG), and echocardiogram. The medical history should focus on pre-existing cardiovascular disease, risk factors for cardiovascular disease (e.g., hypertension, diabetes, hyperlipidemia, smoking), and previous cancer therapy. The physical examination should assess blood pressure, heart rate, and signs of heart failure (e.g., edema, jugular venous distension). The ECG can detect arrhythmias and ischemic changes. The echocardiogram is the primary imaging modality for assessing LVEF and detecting structural heart disease.
4.2 Monitoring During Cancer Therapy
During cancer therapy, patients should be monitored for signs and symptoms of cardiovascular complications, such as chest pain, shortness of breath, edema, and palpitations. ECG should be performed periodically to detect arrhythmias. LVEF should be monitored regularly, particularly in patients receiving anthracyclines or HER2-targeted therapies. The frequency of LVEF monitoring depends on the dose of anthracyclines and the presence of risk factors. Emerging imaging techniques, such as cardiac magnetic resonance imaging (CMR) and speckle tracking echocardiography (STE), can detect subclinical cardiac dysfunction and may be useful for early risk stratification. Biomarkers, such as troponin and natriuretic peptides (BNP or NT-proBNP), can also be used to monitor for cardiac damage.
4.3 Monitoring After Cancer Therapy
After cancer therapy, patients should continue to be monitored for long-term cardiovascular complications. The frequency and type of monitoring depend on the specific cancer therapy, the dose and duration of treatment, and the presence of risk factors. Patients who received anthracyclines or radiation therapy to the chest should undergo periodic LVEF monitoring and surveillance for coronary artery disease and valvular heart disease. All cancer survivors should be encouraged to adopt a heart-healthy lifestyle and manage cardiovascular risk factors.
Many thanks to our sponsor Esdebe who helped us prepare this research report.
5. Cardioprotective Strategies
5.1 Lifestyle Modifications
Lifestyle modifications, such as regular exercise, a healthy diet, and smoking cessation, are essential for reducing cardiovascular risk in cancer patients and survivors. Exercise can improve cardiovascular fitness, reduce inflammation, and improve endothelial function. A healthy diet, rich in fruits, vegetables, and whole grains, can lower blood pressure, cholesterol, and blood sugar. Smoking cessation is crucial for preventing coronary artery disease and other cardiovascular complications.
5.2 Pharmacological Interventions
-
ACE Inhibitors and Angiotensin Receptor Blockers (ARBs): ACE inhibitors and ARBs can protect against anthracycline-induced cardiotoxicity by reducing oxidative stress, inflammation, and myocardial remodeling. These agents are recommended for patients who develop asymptomatic decreases in LVEF during cancer therapy.
-
Beta-Blockers: Beta-blockers can protect against anthracycline-induced cardiotoxicity by reducing heart rate and blood pressure, and by inhibiting the effects of catecholamines on the heart. These agents are recommended for patients who develop asymptomatic decreases in LVEF during cancer therapy.
-
Statins: Statins can protect against anthracycline-induced cardiotoxicity by reducing cholesterol levels, improving endothelial function, and reducing inflammation. Some studies have shown that statins can prevent or delay the onset of heart failure in patients receiving anthracyclines.
-
Dexrazoxane: Dexrazoxane is an iron chelator that can protect against anthracycline-induced cardiotoxicity by reducing the formation of ROS. Dexrazoxane is approved for use in patients receiving high doses of doxorubicin for the treatment of metastatic breast cancer. However, concerns have been raised about its potential to interfere with the anti-tumor efficacy of anthracyclines.
-
Diuretics: Diuretics are used to manage heart failure symptoms, such as edema and shortness of breath.
-
Mineralocorticoid Receptor Antagonists (MRAs): Spironolactone and eplerenone can improve outcomes in patients with heart failure by blocking the effects of aldosterone on the heart and kidneys.
5.3 Novel Therapies
-
Stem Cell Therapy: Stem cell therapy is a promising approach for treating heart failure and repairing damaged myocardium. Several clinical trials are investigating the safety and efficacy of stem cell therapy in patients with CTRCD. However, more research is needed to determine the optimal cell type, delivery method, and timing of stem cell therapy.
-
Remote Ischemic Conditioning (RIC): RIC involves brief periods of ischemia and reperfusion in a remote organ, such as the arm or leg, to protect against ischemia-reperfusion injury in the heart. RIC has been shown to reduce myocardial infarct size and improve cardiac function in patients with acute myocardial infarction. Some studies have suggested that RIC may also protect against anthracycline-induced cardiotoxicity, but more research is needed to confirm these findings.
-
Targeted Therapies for Specific Mechanisms: As our understanding of the mechanisms underlying CTRCD improves, new targeted therapies are being developed to address specific pathways involved in cardiac damage. For example, therapies targeting inflammation, oxidative stress, and endothelial dysfunction are being investigated as potential cardioprotective strategies.
Many thanks to our sponsor Esdebe who helped us prepare this research report.
6. Future Directions
Future research should focus on:
- Identifying biomarkers that can predict the risk of CTRCD before, during, and after cancer therapy.
- Developing more sensitive and specific imaging techniques for early detection of subclinical cardiac dysfunction.
- Conducting large-scale randomized controlled trials to evaluate the efficacy of cardioprotective strategies in preventing or mitigating CTRCD.
- Investigating the long-term cardiovascular outcomes of cancer survivors and developing strategies to improve their quality of life.
- Developing personalized approaches to cancer therapy that take into account the individual patient’s cardiovascular risk profile.
Many thanks to our sponsor Esdebe who helped us prepare this research report.
7. Conclusion
Cancer therapy can cause significant cardiovascular complications, which can impact the quality of life and survival of cancer patients and survivors. Understanding the mechanisms underlying CTRCD is crucial for developing effective strategies for prevention, early detection, and management. Early and ongoing cardiovascular monitoring is essential for identifying patients at risk and detecting subclinical cardiac dysfunction. Lifestyle modifications and pharmacological interventions can help to reduce cardiovascular risk and improve outcomes. Novel therapies, such as stem cell therapy and RIC, hold promise for treating heart failure and repairing damaged myocardium. By implementing a comprehensive approach to cardiovascular care, we can minimize the cardiotoxic effects of cancer therapies and improve the long-term health of cancer survivors.
Many thanks to our sponsor Esdebe who helped us prepare this research report.
References
- Ewer, M. S., & Cardinale, D. (2023). Cardiotoxicity of anticancer treatments: what oncologists need to know. Nature Reviews Clinical Oncology, 20(1), 17-32.
- Zamora, E., et al. (2016). Cardiovascular complications from cancer therapy. World Journal of Cardiology, 8(8), 441–454.
- Carvalho, A. C., et al. (2018). Pathophysiology of anthracycline-induced cardiotoxicity: From bench to bedside. Current Cardiology Reports, 20(9), 74.
- Moslehi, J. J. (2016). Cardiovascular toxicities of targeted cancer therapies. New England Journal of Medicine, 375(15), 1457-1467.
- Yeh, E. T. H., & Bickford, C. L. (2009). Cardiovascular complications of cancer therapy: incidence, pathogenesis, diagnosis, and management. Journal of the American College of Cardiology, 53(24), 2231-2247.
- Curigliano, G., et al. (2016). Management of cardiac disease in cancer patients throughout oncological treatment: ESMO consensus recommendations. Annals of Oncology, 27(2), 256-274.
- Lyon, A. R., et al. (2022). 2022 ESC Guidelines for the diagnosis and treatment of acute and chronic heart failure. European Heart Journal, 43(36), 3627-3739.
- Gupta, D., et al. (2016). Cardio-oncology: A review of anthracycline-induced cardiotoxicity. Texas Heart Institute Journal, 43(1), 18-25.
- Felker, G. M., et al. (2000). Cyclophosphamide-induced cardiotoxicity: a clinical review. Journal of Clinical Oncology, 18(1), 93-98.
- Armenian, S. H., et al. (2018). Cardiovascular disease among adult survivors of childhood cancer: a report from the Childhood Cancer Survivor Study. Circulation, 138(2), 119-130.
- Cardinale, D., et al. (2010). Anthracycline-induced cardiotoxicity: clinical relevance and preventive strategies. European Heart Journal, 31(16), 1943-1949.
- Herrmann, J. (2015). Comprehensive management of cancer therapy-associated cardiovascular toxicity. Mayo Clinic Proceedings, 90(10), 1387-1406.
- Salem, J. E., et al. (2018). Cardiovascular toxicities associated with immune checkpoint inhibitors: an integrative approach. European Heart Journal, 39(39), 3541-3549.
- Esfahani, K., et al. (2019). Cardiotoxicity of immune checkpoint inhibitors: insights and challenges. Journal of the American Heart Association, 8(8), e011937.
- Larson, K. K., et al. (2020). Cardiovascular toxicities associated with chimeric antigen receptor T-cell therapy. Circulation, 141(16), 1339-1347.
- Zamorano, J. L., et al. (2016). Non-invasive cardiac imaging for the evaluation of cardiovascular complications of cancer therapy. European Heart Journal, 37(27), 2105-2114.
- McGowan, J. V., et al. (2017). Anthracycline cardiotoxicity: mechanisms, prevention and management. Expert Opinion on Drug Safety, 16(6), 691-700.
- Kalay, N., et al. (2006). Protective effect of carvedilol against anthracycline-induced cardiotoxicity in rats. Journal of the American College of Cardiology, 48(11), 2458-2462.
- Seicean, S., et al. (2012). Use of statins and incidence of heart failure after anthracycline therapy. Journal of the American College of Cardiology, 60(16), 1520-1526.
- Swain, S. M., et al. (2007). Cardioprotection with dexrazoxane for doxorubicin-containing therapy in advanced breast cancer. Journal of Clinical Oncology, 25(35), 5318-5326.
- Hausenloy, D. J., & Yellon, D. M. (2008). Remote ischaemic preconditioning: underlying mechanisms and clinical application. Cardiovascular Research, 79(2), 117-129.
The discussion of early detection of subclinical cardiac dysfunction through imaging techniques like CMR and STE is valuable. How readily available and cost-effective are these technologies for widespread use in monitoring cancer patients, particularly in community oncology settings?
That’s a great point! While CMR and STE are powerful tools, accessibility and cost are key challenges for widespread adoption, especially in community oncology. Further research into cost-effective strategies and point-of-care solutions could significantly improve early detection efforts in these settings. Let’s discuss potential solutions!
Editor: MedTechNews.Uk
Thank you to our Sponsor Esdebe
So, lifestyle modifications are key, got it! Does that mean my daily dose of dark chocolate counts as a heart-healthy intervention? Asking for a friend… who really likes chocolate.
That’s a great question! While dark chocolate can offer some benefits, remember moderation is key. Focus on incorporating regular exercise and a balanced diet rich in fruits and vegetables for optimal cardiovascular health alongside those occasional treats! Let’s keep this discussion going and share our favorite healthy habits.
Editor: MedTechNews.Uk
Thank you to our Sponsor Esdebe
So, basically, beating cancer might give your heart a hard time! Good thing we’re thinking ahead with monitoring and strategies. Guess I’ll add “avoid becoming a science experiment” to my to-do list.