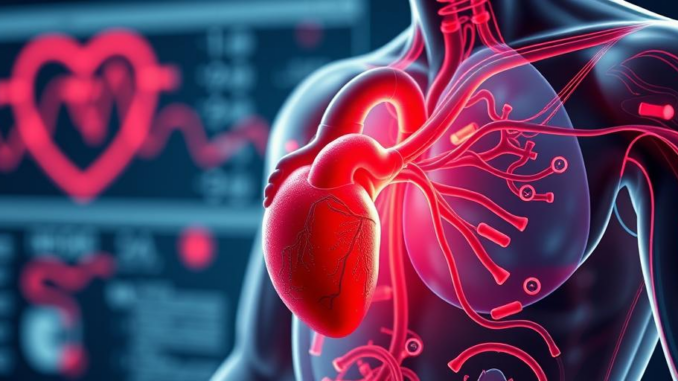
Abstract
Cardiovascular disease (CVD) remains a leading cause of morbidity and mortality worldwide, encompassing a complex interplay of genetic predisposition, lifestyle factors, and underlying pathophysiological mechanisms. This comprehensive review delves into the multifaceted nature of CVD, exploring its diverse etiologies, established and emerging risk factors, and the evolving landscape of therapeutic interventions. Special attention is given to the unexpected cardiovascular benefits observed with sodium-glucose cotransporter-2 (SGLT2) inhibitors, initially developed for the treatment of type 2 diabetes. We critically evaluate the proposed mechanisms underlying these benefits, including their impact on hemodynamic parameters, myocardial metabolism, and inflammatory pathways. Furthermore, we discuss the clinical evidence supporting the use of SGLT2 inhibitors in various cardiovascular conditions, such as heart failure and atherosclerotic cardiovascular disease, comparing their efficacy to traditional therapies. Finally, we address the long-term cardiovascular outcomes associated with SGLT2 inhibitor use and highlight potential risks, particularly in vulnerable patient populations. This review aims to provide a comprehensive and up-to-date overview of CVD, offering insights for clinicians and researchers in this rapidly evolving field.
Many thanks to our sponsor Esdebe who helped us prepare this research report.
1. Introduction
Cardiovascular disease (CVD) is an umbrella term encompassing a range of disorders affecting the heart and blood vessels. These include coronary artery disease (CAD), cerebrovascular disease, peripheral artery disease (PAD), aortic disease, heart failure (HF), and congenital heart defects. Despite significant advances in prevention and treatment, CVD continues to be a major global health burden, accounting for a substantial proportion of deaths and disability-adjusted life years (DALYs) worldwide [1]. The complex etiology of CVD involves a combination of genetic factors, modifiable risk factors, and environmental influences. Understanding the interplay of these factors is crucial for developing effective strategies for primary and secondary prevention.
Traditional risk factors for CVD, such as hypertension, hyperlipidemia, diabetes mellitus, smoking, and obesity, have been extensively studied and are well-established contributors to the development and progression of atherosclerotic disease [2]. However, emerging evidence suggests that other factors, including chronic inflammation, oxidative stress, and endothelial dysfunction, also play significant roles [3]. Furthermore, the gut microbiome has recently emerged as a potential modulator of CVD risk, with specific microbial metabolites implicated in the pathogenesis of atherosclerosis and heart failure [4]. The complexity of CVD underscores the need for a holistic approach to prevention and treatment, targeting multiple risk factors and addressing the underlying pathophysiological mechanisms.
The therapeutic landscape for CVD has evolved significantly over the past decades, with the introduction of novel pharmacological agents and interventional procedures. Statins, angiotensin-converting enzyme (ACE) inhibitors, beta-blockers, and antiplatelet agents remain cornerstones of CVD management, but their effectiveness is often limited by incomplete risk reduction and the development of adverse effects [5]. In recent years, sodium-glucose cotransporter-2 (SGLT2) inhibitors, initially developed for the treatment of type 2 diabetes, have emerged as promising therapeutic agents for CVD, demonstrating unexpected cardiovascular benefits beyond glycemic control [6]. This review aims to provide a comprehensive overview of CVD, encompassing its pathophysiology, risk factors, therapeutic options, and the emerging role of SGLT2 inhibitors in cardiovascular protection.
Many thanks to our sponsor Esdebe who helped us prepare this research report.
2. Pathophysiology of Cardiovascular Disease
The pathophysiology of CVD is diverse and varies depending on the specific condition. However, several key mechanisms contribute to the development and progression of most forms of CVD, including:
2.1 Atherosclerosis
Atherosclerosis is the underlying cause of most cases of CAD, cerebrovascular disease, and PAD. It is a chronic inflammatory disease characterized by the accumulation of lipids, inflammatory cells, and fibrous tissue within the arterial wall, leading to the formation of atherosclerotic plaques [7]. The process begins with endothelial dysfunction, which allows lipoproteins, particularly low-density lipoprotein (LDL), to enter the intima, the innermost layer of the arterial wall. Modified LDL particles trigger an inflammatory response, attracting monocytes and T lymphocytes to the site. Monocytes differentiate into macrophages, which engulf the modified LDL, becoming foam cells. These foam cells contribute to the formation of fatty streaks, the earliest visible lesions of atherosclerosis. Over time, the fatty streaks progress to more complex plaques, characterized by a lipid-rich core, a fibrous cap, and inflammatory cells. Plaque rupture or erosion can lead to thrombosis and acute ischemic events, such as myocardial infarction or stroke [8].
2.2 Hypertension
Hypertension is a major risk factor for CVD, contributing to the development of atherosclerosis, heart failure, stroke, and kidney disease. Elevated blood pressure increases the workload on the heart, leading to left ventricular hypertrophy and diastolic dysfunction. Hypertension also damages the endothelial lining of blood vessels, accelerating the atherosclerotic process. The pathophysiology of hypertension is complex and multifactorial, involving dysregulation of the renin-angiotensin-aldosterone system (RAAS), sympathetic nervous system, and endothelial function [9]. Genetic factors, lifestyle factors, and environmental influences also play a significant role in the development of hypertension.
2.3 Heart Failure
Heart failure is a clinical syndrome characterized by the inability of the heart to pump sufficient blood to meet the body’s needs. It can result from a variety of underlying conditions, including CAD, hypertension, valvular heart disease, and cardiomyopathy. The pathophysiology of heart failure involves complex interactions between hemodynamic overload, neurohormonal activation, and myocardial remodeling [10]. Neurohormonal activation, particularly of the RAAS and sympathetic nervous system, leads to sodium and water retention, vasoconstriction, and increased cardiac workload. Myocardial remodeling, characterized by changes in the size, shape, and function of the heart, further contributes to the progression of heart failure. Heart failure can be classified based on left ventricular ejection fraction (LVEF) into heart failure with reduced ejection fraction (HFrEF), heart failure with preserved ejection fraction (HFpEF), and heart failure with mid-range ejection fraction (HFmrEF). The pathophysiology and treatment strategies differ depending on the type of heart failure.
2.4 Arrhythmias
Cardiac arrhythmias are abnormalities in the heart’s rhythm, ranging from benign palpitations to life-threatening ventricular fibrillation. Arrhythmias can result from a variety of underlying conditions, including CAD, heart failure, valvular heart disease, and electrolyte imbalances. The pathophysiology of arrhythmias involves abnormalities in the electrical impulse formation, conduction, or both [11]. These abnormalities can be caused by changes in ion channel function, structural heart disease, or autonomic nervous system dysfunction. Atrial fibrillation (AF) is the most common sustained arrhythmia and is associated with an increased risk of stroke, heart failure, and death. Ventricular arrhythmias, such as ventricular tachycardia and ventricular fibrillation, can lead to sudden cardiac death.
Many thanks to our sponsor Esdebe who helped us prepare this research report.
3. Risk Factors for Cardiovascular Disease
Identifying and managing risk factors is crucial for preventing and managing CVD. Risk factors can be broadly classified into modifiable and non-modifiable categories.
3.1 Modifiable Risk Factors
- Hypertension: Elevated blood pressure is a major risk factor for CVD. Management involves lifestyle modifications (diet, exercise, weight loss) and pharmacological therapy (ACE inhibitors, angiotensin receptor blockers, beta-blockers, diuretics, calcium channel blockers).
- Hyperlipidemia: Elevated levels of LDL cholesterol are associated with an increased risk of atherosclerosis. Management involves lifestyle modifications (diet, exercise) and pharmacological therapy (statins, ezetimibe, PCSK9 inhibitors).
- Diabetes Mellitus: Diabetes increases the risk of CVD by promoting atherosclerosis, endothelial dysfunction, and inflammation. Management involves lifestyle modifications (diet, exercise, weight loss), pharmacological therapy (metformin, sulfonylureas, thiazolidinediones, SGLT2 inhibitors, GLP-1 receptor agonists, insulin), and blood glucose control.
- Smoking: Smoking damages the endothelial lining of blood vessels, promotes atherosclerosis, and increases the risk of thrombosis. Smoking cessation is crucial for reducing CVD risk.
- Obesity: Obesity is associated with increased risk of hypertension, hyperlipidemia, diabetes, and heart failure. Weight loss through lifestyle modifications (diet, exercise) or bariatric surgery can reduce CVD risk.
- Physical Inactivity: Lack of physical activity increases the risk of CVD. Regular exercise improves cardiovascular health and reduces CVD risk.
- Unhealthy Diet: Diets high in saturated and trans fats, cholesterol, sodium, and sugar increase CVD risk. A heart-healthy diet, rich in fruits, vegetables, whole grains, and lean protein, reduces CVD risk.
- Stress: Chronic stress can contribute to CVD by increasing blood pressure, heart rate, and inflammation. Stress management techniques, such as yoga, meditation, and deep breathing, can reduce CVD risk.
3.2 Non-Modifiable Risk Factors
- Age: The risk of CVD increases with age.
- Sex: Men are generally at higher risk of CVD than women, although the risk increases for women after menopause.
- Family History: A family history of premature CVD increases an individual’s risk.
- Genetics: Genetic factors play a significant role in CVD susceptibility. Genome-wide association studies (GWAS) have identified numerous genetic variants associated with CVD risk.
Many thanks to our sponsor Esdebe who helped us prepare this research report.
4. Emerging Therapies for Cardiovascular Disease
Beyond traditional therapies, several emerging approaches are showing promise in the prevention and treatment of CVD:
4.1 PCSK9 Inhibitors
Proprotein convertase subtilisin/kexin type 9 (PCSK9) inhibitors are monoclonal antibodies that lower LDL cholesterol levels by preventing the degradation of LDL receptors on hepatocytes [12]. Clinical trials have demonstrated that PCSK9 inhibitors significantly reduce LDL cholesterol levels and cardiovascular events in patients at high risk for CVD. They are typically used in patients who are not able to achieve adequate LDL cholesterol lowering with statins alone.
4.2 Inclisiran
Inclisiran is a small interfering RNA (siRNA) molecule that inhibits the synthesis of PCSK9 in the liver. It provides sustained LDL cholesterol lowering with twice-yearly subcutaneous injections. Clinical trials have shown that inclisiran significantly reduces LDL cholesterol levels and cardiovascular events [13].
4.3 Vericiguat
Vericiguat is a soluble guanylate cyclase (sGC) stimulator that enhances the production of cyclic GMP (cGMP), a signaling molecule that promotes vasodilation and reduces inflammation. Clinical trials have demonstrated that vericiguat reduces the risk of cardiovascular death and heart failure hospitalization in patients with heart failure and reduced ejection fraction [14].
4.4 Gene Therapy
Gene therapy is an emerging approach for treating CVD by delivering therapeutic genes to target cells. Gene therapy can be used to increase the expression of beneficial genes (e.g., those encoding for anti-inflammatory cytokines or angiogenesis-promoting factors) or to silence the expression of harmful genes (e.g., those encoding for pro-inflammatory cytokines or pro-thrombotic factors) [15]. Gene therapy is still in the early stages of development, but it holds great promise for treating a variety of cardiovascular conditions.
Many thanks to our sponsor Esdebe who helped us prepare this research report.
5. SGLT2 Inhibitors and Cardiovascular Disease: Unexpected Benefits
Sodium-glucose cotransporter-2 (SGLT2) inhibitors were initially developed for the treatment of type 2 diabetes. These drugs work by inhibiting the reabsorption of glucose in the proximal tubule of the kidney, leading to increased urinary glucose excretion and reduced blood glucose levels. Surprisingly, clinical trials have demonstrated that SGLT2 inhibitors also have significant cardiovascular benefits, independent of their glucose-lowering effects [6].
5.1 Mechanisms of Cardiovascular Protection
The mechanisms underlying the cardiovascular benefits of SGLT2 inhibitors are complex and multifactorial. Several potential mechanisms have been proposed, including:
- Hemodynamic Effects: SGLT2 inhibitors reduce blood pressure and weight, both of which can improve cardiovascular function [16]. They achieve this by inducing osmotic diuresis, reducing plasma volume, and promoting natriuresis. Furthermore, studies have shown that SGLT2i use may improve arterial stiffness, which is a known predictor of adverse cardiovascular events. They also cause a mild reduction in blood pressure, which could also improve cardiovascular function.
- Metabolic Effects: SGLT2 inhibitors improve glucose control and reduce insulin resistance, which can reduce the risk of atherosclerosis and other cardiovascular complications. They shift the primary cardiac fuel source from glucose to ketone bodies. This metabolic shift has been suggested to improve cardiac efficiency, particularly in heart failure where cardiac energy production is often compromised.
- Myocardial Remodeling: SGLT2 inhibitors have been shown to reduce left ventricular hypertrophy and improve cardiac function in patients with heart failure [17]. They decrease myocardial fibrosis, which is associated with improved diastolic function. This could be particularly beneficial in HFpEF.
- Anti-Inflammatory Effects: SGLT2 inhibitors have been shown to reduce inflammation, which can protect against atherosclerosis and other cardiovascular complications [18]. They may modulate immune cell activity, reducing the pro-inflammatory milieu that contributes to CVD progression.
- Improved Endothelial Function: SGLT2 inhibitors may improve endothelial function, which is crucial for maintaining vascular health.
- Reduced Oxidative Stress: They are thought to reduce oxidative stress through various mechanisms, including reducing glucose-mediated oxidative pathways and improving mitochondrial function. Oxidative stress is a key contributor to endothelial dysfunction and atherosclerosis.
5.2 Clinical Evidence for Cardiovascular Benefits
Several large-scale clinical trials have demonstrated the cardiovascular benefits of SGLT2 inhibitors in patients with type 2 diabetes and CVD. These trials have shown that SGLT2 inhibitors reduce the risk of major adverse cardiovascular events (MACE), including cardiovascular death, myocardial infarction, and stroke [19].
Furthermore, clinical trials have shown that SGLT2 inhibitors reduce the risk of heart failure hospitalization and cardiovascular death in patients with heart failure, regardless of whether they have diabetes [20]. These findings have led to the widespread adoption of SGLT2 inhibitors as a first-line therapy for heart failure with reduced ejection fraction (HFrEF).
The EMPEROR-Preserved trial demonstrated the benefit of empagliflozin in patients with HFpEF, extending the benefits of SGLT2 inhibitors to this previously underserved population [21]. This result was a significant breakthrough, as effective treatments for HFpEF have been limited.
5.3 Comparison to Other Cardiovascular Medications
SGLT2 inhibitors offer several advantages over traditional cardiovascular medications, including their ability to reduce blood glucose levels, blood pressure, and weight, in addition to their cardiovascular benefits. They have also been shown to be well-tolerated, with a low risk of hypoglycemia [22].
However, SGLT2 inhibitors are not without their limitations. They can increase the risk of genital infections, particularly in women, and can also cause euglycemic diabetic ketoacidosis in rare cases [23]. Furthermore, the long-term cardiovascular outcomes associated with SGLT2 inhibitor use are still being investigated.
5.4 Long-Term Cardiovascular Outcomes and Potential Risks
While the short- to medium-term cardiovascular benefits of SGLT2 inhibitors are well-established, the long-term cardiovascular outcomes are still being investigated. Some studies have suggested that the cardiovascular benefits of SGLT2 inhibitors may wane over time, while others have shown sustained benefits over several years [24].
Potential risks associated with SGLT2 inhibitor use include genital infections, euglycemic diabetic ketoacidosis, and volume depletion. These risks are generally low, but it is important to monitor patients for these complications [23]. Furthermore, SGLT2 inhibitors should be used with caution in patients with pre-existing kidney disease, as they can potentially worsen renal function. A meta-analysis showed a slightly elevated risk for lower limb amputations, although subsequent trials have largely mitigated this concern. Careful patient selection and monitoring are crucial to mitigate potential risks.
Many thanks to our sponsor Esdebe who helped us prepare this research report.
6. Conclusion
Cardiovascular disease remains a major global health challenge, requiring a multifaceted approach to prevention and treatment. Traditional risk factors, such as hypertension, hyperlipidemia, diabetes, and smoking, continue to play a significant role in the development and progression of CVD. However, emerging evidence suggests that other factors, including chronic inflammation, oxidative stress, and the gut microbiome, also contribute to CVD risk. The therapeutic landscape for CVD is constantly evolving, with the introduction of novel pharmacological agents and interventional procedures. SGLT2 inhibitors, initially developed for the treatment of type 2 diabetes, have emerged as promising therapeutic agents for CVD, demonstrating unexpected cardiovascular benefits beyond glycemic control. These benefits are likely mediated by a combination of hemodynamic, metabolic, and anti-inflammatory effects. Clinical trials have demonstrated that SGLT2 inhibitors reduce the risk of MACE, heart failure hospitalization, and cardiovascular death in patients with type 2 diabetes and CVD. Furthermore, SGLT2 inhibitors have been shown to be effective in patients with heart failure, regardless of whether they have diabetes. While the long-term cardiovascular outcomes associated with SGLT2 inhibitor use are still being investigated, the current evidence suggests that these drugs offer significant cardiovascular benefits, particularly in patients with type 2 diabetes and heart failure. Future research should focus on further elucidating the mechanisms underlying the cardiovascular benefits of SGLT2 inhibitors and identifying the patient populations that are most likely to benefit from these drugs. Further research should also investigate optimal dosing strategies and long-term safety profiles, particularly in specific patient subgroups with existing cardiovascular issues and to explore the potential benefits in those without diabetes.
Many thanks to our sponsor Esdebe who helped us prepare this research report.
References
[1] Roth, G. A., et al. (2020). Global burden of cardiovascular diseases and risk factors, 1990-2019: Update From the GBD 2019 Study. Journal of the American College of Cardiology, 76(25), 2982-3021.
[2] Yusuf, S., et al. (2004). Effect of potentially modifiable risk factors associated with myocardial infarction in 52 countries (the INTERHEART study): case-control study. The Lancet, 364(9438), 937-952.
[3] Libby, P. (2021). Inflammation in atherosclerosis. Nature, 592(7855), 542-551.
[4] Tang, W. H. W., et al. (2017). Intestinal microbial metabolism of phosphatidylcholine and cardiovascular risk. New England Journal of Medicine, 368(17), 1575-1584.
[5] Collins, R., et al. (2016). Interpretation of the evidence for the efficacy and safety of statin therapy. The Lancet, 388(10059), 2532-2561.
[6] Packer, M., et al. (2020). Cardiovascular and renal outcomes with empagliflozin in heart failure. New England Journal of Medicine, 383(15), 1413-1424.
[7] Lusis, A. J. (2000). Atherosclerosis. Nature, 407(6801), 233-241.
[8] Libby, P., et al. (2019). Inflammation in atherosclerosis from pathophysiology to practice. Journal of the American College of Cardiology, 74(17), 2292-2309.
[9] Carey, R. M., et al. (2018). Resistance to mineralocorticoid hormones: Hypertension, cardiovascular, and metabolic syndrome. Endocrine Reviews, 39(3), 289-335.
[10] Cohn, J. N., et al. (1998). The neurohormonal hypothesis in heart failure: pathophysiology and implications for therapy. American Journal of Hypertension, 11(8 Pt 2), 88S-98S.
[11] Zipes, D. P., & Jalife, J. (2009). Cardiac electrophysiology: from cell to bedside. Elsevier Health Sciences.
[12] Sabatine, M. S., et al. (2017). Evolocumab and clinical outcomes in patients with cardiovascular disease. New England Journal of Medicine, 376(18), 1713-1722.
[13] Ray, K. K., et al. (2020). Inclisiran in patients at high cardiovascular risk with elevated LDL cholesterol. New England Journal of Medicine, 382(16), 1507-1516.
[14] Armstrong, P. W., et al. (2020). Vericiguat in patients with heart failure and reduced ejection fraction. New England Journal of Medicine, 382(20), 1883-1893.
[15] Hajjar, R. J., & Schmidt-Lucke, C. (2009). Gene therapy for heart failure. Nature Reviews Cardiology, 6(4), 254-263.
[16] Lambers Heerspink, H. J., et al. (2016). Dapagliflozin reduces albuminuria in patients with diabetes and chronic kidney disease. Nature Reviews Nephrology, 12(9), 553-561.
[17] Verma, S., et al. (2019). Empagliflozin in heart failure with a preserved ejection fraction. Circulation, 139(3), 351-353.
[18] Chilton, R., et al. (2015). Potential mechanisms by which sodium-glucose cotransporter 2 (SGLT2) inhibitors reduce heart failure in type 2 diabetes. Diabetic Medicine, 32(1), 4-14.
[19] Zinman, B., et al. (2015). Empagliflozin, cardiovascular outcomes, and mortality in type 2 diabetes. New England Journal of Medicine, 373(22), 2117-2128.
[20] McMurray, J. J. V., et al. (2019). Dapagliflozin in patients with heart failure and reduced ejection fraction. New England Journal of Medicine, 381(21), 1995-2008.
[21] Anker, S. D., et al. (2021). Empagliflozin in Heart Failure with a Preserved Ejection Fraction. New England Journal of Medicine, 385(16), 1451-1461.
[22] Neal, B., et al. (2017). Canagliflozin and cardiovascular and renal events in type 2 diabetes. New England Journal of Medicine, 377(7), 644-657.
[23] Watts, V., et al. (2016). SGLT2 inhibitors: risks and benefits beyond glycaemic control. The Lancet Diabetes & Endocrinology, 4(10), 823-833.
[24] Zannad, F., et al. (2020). SGLT2 inhibitors in heart failure: consensus recommendations from the European Society of Cardiology heart failure association. European Journal of Heart Failure, 22(1), 26-38.
So, SGLT2 inhibitors make us pee out sugar, and *that’s* good for the heart? Are we sure the answer isn’t just aggressive hydration and a really efficient colander?
That’s a brilliant and funny take! While the ‘pee out sugar’ simplification is true, it’s more nuanced. The aggressive hydration aspect you mentioned is definitely a piece of the puzzle, affecting blood volume and pressure, which in turn influences cardiovascular health. SGLT2 inhibitors impact several other pathways too though, so keep reading!
Editor: MedTechNews.Uk
Thank you to our Sponsor Esdebe
SGLT2 inhibitors reducing inflammation? So, are we saying my gummy bear intake is now a legitimate therapeutic strategy, or is it more complicated than just ‘less inflammation = happy heart’? Asking for a friend…who *really* likes gummy bears.