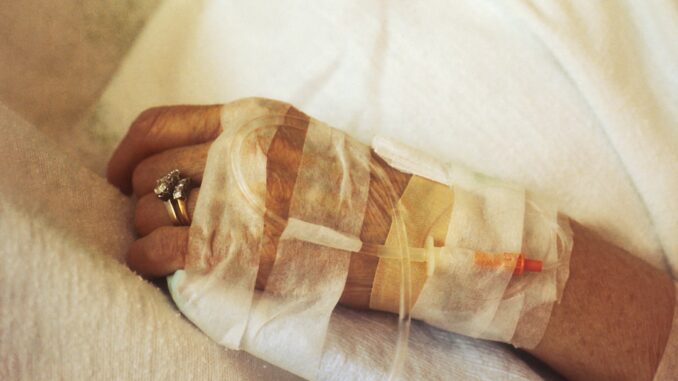
Chemotherapy: Advancements, Challenges, and the Evolving Landscape of Targeted Therapies
Abstract
Chemotherapy, a cornerstone of cancer treatment for over half a century, encompasses a diverse range of cytotoxic drugs that target rapidly dividing cells. While effective in many contexts, its systemic nature and lack of specificity lead to significant off-target toxicities and, in some cases, the development of drug resistance. This research report delves into the multifaceted world of chemotherapy, examining the mechanisms of action of various chemotherapeutic agents, their clinical applications across different cancer types, the challenges associated with their use, and the innovative strategies being developed to enhance efficacy and minimize adverse effects. Furthermore, the report explores the evolving landscape of targeted therapies and immunotherapies and considers the role of chemotherapy in combination with these novel approaches. Finally, we present a forward-looking perspective on the future of chemotherapy, considering personalized medicine and emerging delivery systems.
1. Introduction
Chemotherapy’s introduction in the mid-20th century marked a paradigm shift in cancer treatment, offering systemic approaches to eradicate malignant cells. The early agents, derived from natural products and chemical warfare research, exhibited broad cytotoxicity, targeting fundamental cellular processes like DNA replication and mitosis. This indiscriminate action, while effective against rapidly proliferating cancer cells, also affected healthy tissues, resulting in the characteristic side effects associated with chemotherapy, such as myelosuppression, mucositis, and alopecia [1].
Despite these limitations, chemotherapy remains a crucial component of cancer treatment regimens for many malignancies. Its role has evolved significantly with the development of new chemotherapeutic agents, refined dosing schedules, and improved supportive care strategies to mitigate toxicities. Furthermore, the advent of targeted therapies and immunotherapies has prompted a re-evaluation of chemotherapy’s place in the treatment algorithm, with combination approaches becoming increasingly prevalent.
This report aims to provide a comprehensive overview of chemotherapy, encompassing its historical development, mechanisms of action, clinical applications, limitations, and future directions. We will examine the different classes of chemotherapeutic agents, the challenges associated with drug resistance and systemic toxicities, and the innovative strategies being developed to overcome these hurdles. Finally, we will explore the integration of chemotherapy with targeted therapies and immunotherapies, highlighting the potential for synergistic effects and improved patient outcomes.
2. Mechanisms of Action of Chemotherapeutic Agents
Chemotherapeutic agents exert their cytotoxic effects through a variety of mechanisms, targeting different stages of the cell cycle and critical cellular processes. These agents can be broadly classified into several categories based on their mechanism of action:
-
Alkylating Agents: These drugs, such as cyclophosphamide and cisplatin, covalently bind to DNA, forming adducts that interfere with DNA replication and transcription. Alkylation can occur at various sites on the DNA molecule, leading to cross-linking and strand breaks. The resulting DNA damage triggers cell cycle arrest and apoptosis [2]. Cisplatin, in particular, is a highly effective alkylating agent widely used in the treatment of various solid tumors. However, its use is often limited by nephrotoxicity and neurotoxicity.
-
Antimetabolites: Antimetabolites mimic naturally occurring molecules required for DNA and RNA synthesis. Examples include methotrexate, which inhibits dihydrofolate reductase, an enzyme essential for folate metabolism, and 5-fluorouracil (5-FU), which interferes with thymidylate synthase, a key enzyme in DNA synthesis. By disrupting nucleotide synthesis, antimetabolites halt DNA and RNA replication, leading to cell death [3].
-
Topoisomerase Inhibitors: Topoisomerases are enzymes that regulate DNA topology by cleaving and rejoining DNA strands. Topoisomerase inhibitors, such as etoposide and irinotecan, interfere with this process, causing DNA breaks to accumulate. Etoposide inhibits topoisomerase II, while irinotecan inhibits topoisomerase I. The resulting DNA damage triggers cell cycle arrest and apoptosis [4]. Irinotecan is a prodrug that is converted to its active metabolite, SN-38, in the liver. SN-38 is significantly more potent than irinotecan itself.
-
Microtubule Inhibitors: Microtubules are essential components of the mitotic spindle, which is responsible for segregating chromosomes during cell division. Microtubule inhibitors, such as paclitaxel and vincristine, disrupt microtubule dynamics, preventing the formation of a functional mitotic spindle. This leads to cell cycle arrest at the metaphase-anaphase transition and subsequent apoptosis [5]. Paclitaxel stabilizes microtubules, preventing their depolymerization, while vincristine inhibits microtubule polymerization. These drugs have distinct mechanisms of action but both disrupt cell division.
-
Anthracyclines: Anthracyclines, such as doxorubicin and daunorubicin, are potent cytotoxic agents that exert their effects through multiple mechanisms. They intercalate into DNA, disrupting DNA and RNA synthesis. They also inhibit topoisomerase II and generate free radicals, leading to DNA damage and lipid peroxidation [6]. Anthracyclines are associated with cardiotoxicity, which can limit their long-term use. The development of liposomal formulations of anthracyclines has helped to reduce cardiotoxicity.
The choice of chemotherapeutic agent depends on the specific type of cancer, its stage, and the patient’s overall health. Combination chemotherapy regimens often employ drugs with different mechanisms of action to maximize efficacy and minimize the development of drug resistance.
3. Clinical Applications of Chemotherapy
Chemotherapy plays a critical role in the treatment of a wide range of cancers, including both solid tumors and hematologic malignancies. Its applications can be broadly categorized into:
-
Curative Chemotherapy: In some cases, chemotherapy can lead to complete remission and long-term survival, effectively curing the cancer. This is often seen in hematologic malignancies such as acute lymphoblastic leukemia (ALL) and Hodgkin lymphoma, as well as certain solid tumors such as testicular cancer [7].
-
Adjuvant Chemotherapy: Adjuvant chemotherapy is administered after surgery or radiation therapy to eliminate any residual cancer cells that may be present and reduce the risk of recurrence. This approach is commonly used in breast cancer, colon cancer, and lung cancer [8].
-
Neoadjuvant Chemotherapy: Neoadjuvant chemotherapy is administered before surgery or radiation therapy to shrink the tumor, making it easier to remove or treat. This approach is often used in locally advanced breast cancer, esophageal cancer, and bladder cancer [9].
-
Palliative Chemotherapy: Palliative chemotherapy is used to relieve symptoms and improve quality of life in patients with advanced cancer that is not curable. This approach can help to control tumor growth, reduce pain, and improve overall function [10].
The specific chemotherapy regimen used depends on the type of cancer, its stage, and the patient’s overall health. Chemotherapy is often combined with other treatments, such as surgery, radiation therapy, targeted therapy, and immunotherapy, to achieve the best possible outcome.
4. Challenges in Chemotherapy: Drug Resistance and Toxicities
Despite its effectiveness, chemotherapy faces significant challenges, including the development of drug resistance and the occurrence of systemic toxicities.
4.1 Drug Resistance
Drug resistance is a major obstacle to successful chemotherapy. Cancer cells can develop resistance to chemotherapeutic agents through various mechanisms, including:
-
Increased Drug Efflux: Cancer cells can increase the expression of efflux pumps, such as P-glycoprotein (P-gp), which actively transport chemotherapeutic agents out of the cell, reducing their intracellular concentration and effectiveness [11].
-
Decreased Drug Uptake: Conversely, cancer cells can reduce the expression of transporters that facilitate the uptake of chemotherapeutic agents, limiting their access to intracellular targets.
-
Alterations in Drug Targets: Mutations in the genes encoding drug targets can alter the structure of the target protein, reducing its affinity for the chemotherapeutic agent.
-
Increased DNA Repair: Cancer cells can enhance their DNA repair mechanisms, allowing them to repair the DNA damage caused by chemotherapeutic agents more effectively.
-
Apoptosis Resistance: Cancer cells can develop resistance to apoptosis, the programmed cell death pathway, rendering them less susceptible to the cytotoxic effects of chemotherapeutic agents [12].
Overcoming drug resistance requires a multifaceted approach, including the development of new chemotherapeutic agents that are less susceptible to resistance mechanisms, the use of combination chemotherapy regimens, and the development of strategies to inhibit drug efflux pumps or enhance drug uptake.
4.2 Systemic Toxicities
Chemotherapy’s lack of specificity leads to significant off-target toxicities, affecting rapidly dividing normal cells in the bone marrow, gastrointestinal tract, and hair follicles. Common side effects include:
-
Myelosuppression: Suppression of bone marrow function leads to reduced production of blood cells, resulting in anemia, neutropenia, and thrombocytopenia. This can increase the risk of infection, bleeding, and fatigue [13].
-
Mucositis: Inflammation and ulceration of the mucous membranes lining the gastrointestinal tract, causing pain, difficulty swallowing, and diarrhea [14].
-
Alopecia: Hair loss due to damage to hair follicles [15].
-
Nausea and Vomiting: Activation of chemoreceptor trigger zone in the brain and damage to the gastrointestinal tract can cause nausea and vomiting [16].
-
Cardiotoxicity: Some chemotherapeutic agents, such as anthracyclines, can damage the heart muscle, leading to heart failure [6].
-
Nephrotoxicity: Damage to the kidneys, leading to decreased kidney function [2].
-
Neurotoxicity: Damage to the nervous system, causing peripheral neuropathy, cognitive impairment, and seizures [2].
Managing chemotherapy-induced toxicities requires a multidisciplinary approach, including the use of supportive care medications, such as antiemetics, growth factors, and antibiotics, as well as careful monitoring and dose adjustments. The development of more targeted therapies and drug delivery systems aims to reduce off-target toxicities and improve patient tolerability.
5. Strategies to Enhance Chemotherapy Efficacy and Reduce Toxicity
Numerous strategies are being explored to enhance the efficacy of chemotherapy and reduce its toxicities, including:
-
Targeted Drug Delivery: Nanoparticles, liposomes, and antibody-drug conjugates (ADCs) are being developed to deliver chemotherapeutic agents directly to cancer cells, sparing normal tissues. ADCs, in particular, combine the specificity of antibodies with the cytotoxic potency of chemotherapy, allowing for targeted delivery of drugs to cancer cells expressing specific antigens [17].
-
Prodrug Activation: Prodrugs are inactive forms of chemotherapeutic agents that are activated in the tumor microenvironment by enzymes or other stimuli. This approach can reduce systemic toxicity by minimizing exposure of normal tissues to the active drug [18].
-
Chemosensitization: Strategies to enhance the sensitivity of cancer cells to chemotherapy by inhibiting drug resistance mechanisms or modulating signaling pathways that promote cell survival. For example, inhibitors of P-glycoprotein can increase the intracellular concentration of chemotherapeutic agents in resistant cells [11].
-
Combination Therapies: Combining chemotherapy with targeted therapies or immunotherapies can lead to synergistic effects and improved patient outcomes. For example, combining chemotherapy with EGFR inhibitors in patients with non-small cell lung cancer has shown significant improvements in survival [19].
-
Personalized Medicine: Tailoring chemotherapy regimens to the individual patient based on their genetic profile, tumor characteristics, and overall health. This approach can help to optimize drug selection and dosing, maximizing efficacy and minimizing toxicity [20].
6. Chemotherapy in Combination with Targeted Therapies and Immunotherapies
The integration of chemotherapy with targeted therapies and immunotherapies represents a significant advancement in cancer treatment. Targeted therapies, such as tyrosine kinase inhibitors (TKIs) and monoclonal antibodies, target specific molecules involved in cancer cell growth and survival. Immunotherapies, such as immune checkpoint inhibitors, enhance the body’s own immune system to attack cancer cells.
Combining chemotherapy with targeted therapies or immunotherapies can lead to synergistic effects and improved patient outcomes. For example:
-
Chemotherapy + EGFR Inhibitors: In non-small cell lung cancer (NSCLC) patients with EGFR mutations, combining chemotherapy with EGFR inhibitors, such as gefitinib or erlotinib, has shown significant improvements in progression-free survival and overall survival [19].
-
Chemotherapy + VEGF Inhibitors: In metastatic colorectal cancer, combining chemotherapy with VEGF inhibitors, such as bevacizumab, has improved survival outcomes by inhibiting angiogenesis, the formation of new blood vessels that supply tumors [21].
-
Chemotherapy + Immune Checkpoint Inhibitors: In various cancers, including melanoma, lung cancer, and bladder cancer, combining chemotherapy with immune checkpoint inhibitors, such as pembrolizumab or nivolumab, has shown promising results by enhancing the anti-tumor immune response [22].
However, combining chemotherapy with targeted therapies or immunotherapies can also lead to increased toxicities. Careful monitoring and management of side effects are essential. Ongoing clinical trials are exploring optimal combinations and sequencing strategies to maximize efficacy and minimize toxicity.
7. The Future of Chemotherapy
The future of chemotherapy lies in personalized medicine, targeted drug delivery, and the development of novel agents that overcome drug resistance. Key areas of focus include:
-
Personalized Chemotherapy: Using genomic profiling and other biomarkers to identify patients who are most likely to benefit from specific chemotherapy regimens and to predict and prevent toxicities [20].
-
Novel Drug Delivery Systems: Developing more sophisticated drug delivery systems that can selectively target cancer cells and release chemotherapeutic agents in a controlled manner [17].
-
New Chemotherapeutic Agents: Discovering and developing new chemotherapeutic agents with novel mechanisms of action that are less susceptible to drug resistance [18].
-
Exploiting the Tumor Microenvironment: Developing strategies to target the tumor microenvironment, which plays a critical role in cancer cell growth, survival, and metastasis. This includes targeting cancer-associated fibroblasts, immune cells, and blood vessels [23].
-
Artificial Intelligence and Machine Learning: Applying AI and machine learning algorithms to analyze large datasets of patient data to identify patterns and predict treatment outcomes. This can help to personalize chemotherapy regimens and improve patient outcomes [24].
Chemotherapy will likely remain an important component of cancer treatment for the foreseeable future, but its role will continue to evolve as new therapies and technologies emerge. The goal is to develop more effective and less toxic treatments that can improve the lives of patients with cancer.
8. Conclusion
Chemotherapy has revolutionized cancer treatment, offering curative potential and improving survival rates for many patients. However, the challenges of drug resistance and systemic toxicities remain significant hurdles. Ongoing research is focused on developing innovative strategies to enhance chemotherapy efficacy, reduce toxicities, and personalize treatment approaches. The integration of chemotherapy with targeted therapies and immunotherapies holds great promise for improving patient outcomes. As we move towards a future of personalized medicine, chemotherapy will continue to evolve, playing a vital role in the fight against cancer.
References
[1] Chabner, B. A., & Roberts, T. G. (2005). Timeline: Chemotherapy and the war on cancer. Nature Reviews Cancer, 5(1), 65-75.
[2] Kelland, L. (2007). Preclinical development of new platinum-based anticancer drugs. Nature Reviews Cancer, 7(8), 573-584.
[3] Longley, D. B., & Johnston, P. G. (2005). 5-Fluorouracil: mechanisms of action and clinical strategies. Nature Reviews Cancer, 5(7), 505-517.
[4] Pommier, Y. (2006). DNA topoisomerase I and II inhibitors: chemistry, biology, and mechanisms of action. Biochemistry, 45(50), 14669-14686.
[5] Jordan, M. A., & Wilson, L. (2004). Microtubules as a target for anticancer drugs. Nature Reviews Cancer, 4(4), 253-265.
[6] Tacar, O., Sriamornsak, P., & Dass, C. R. (2013). Doxorubicin: an update on anticancer molecular mechanisms, targeted delivery and overcoming drug resistance. Journal of Pharmacy and Pharmacology, 65(2), 157-170.
[7] DeVita, V. T., Jr., Hellman, S., & Rosenberg, S. A. (Eds.). (2005). Cancer: principles & practice of oncology (7th ed.). Lippincott Williams & Wilkins.
[8] Early Breast Cancer Trialists’ Collaborative Group. (2005). Effects of chemotherapy and hormonal therapy for early breast cancer on recurrence and 15-year survival: an overview of the randomised trials. The Lancet, 365(9472), 1687-1717.
[9] Bear, H. D., Anderson, S., Smith, R. E., Mamounas, E. P., Geyer, C. E., Jr., Azar, C., … Wolmark, N. (2003). Sequential preoperative or postoperative docetaxel added to doxorubicin and cyclophosphamide for operable breast cancer: NSABP B-27. The Lancet, 362(9390), 1064-1074.
[10] Davis, M. P., Temel, J. S., Balboni, T. A., Zimmermann, C., & Mirabelli, K. J. (2017). Integration of early palliative care: Illustrating the role of chemotherapy. CA: a Cancer Journal for Clinicians, 67(2), 103-116.
[11] Gottesman, M. M., Fojo, A. T., & Bates, S. E. (2002). Multidrug resistance in cancer: role of ATP-dependent transporters. Nature Reviews Cancer, 2(1), 48-58.
[12] Lowe, S. W., & Lin, A. W. (2000). Apoptosis in cancer. Carcinogenesis, 21(3), 485-495.
[13] Crawford, J., Dale, D. C., Lyman, G. H. (2004). Chemotherapy-induced neutropenia: risks, consequences, and new directions for its management. Cancer, 100(2), 228-237.
[14] Sonis, S. T. (2004). Oral mucositis in cancer therapy. Journal of Supportive Oncology, 2(3 Suppl 2), 3-8.
[15] Trüeb, R. M. (2009). Chemotherapy-induced alopecia. Seminars in Cutaneous Medicine and Surgery, 28(1), 11-14.
[16] Hesketh, P. J. (2008). Chemotherapy-induced nausea and vomiting. New England Journal of Medicine, 358(23), 2482-2494.
[17] Lambert, J. M., & Morris, A. L. (2017). Antibody-drug conjugates (ADCs) for personalized cancer therapy. Antibodies, 6(4), 28.
[18] Rautio, J., Kumpulainen, H., Heimbach, T., Oliyai, R., Oh, D., Järvinen, T., & Savolainen, J. (2008). Prodrugs: design and clinical applications. Nature Reviews Drug Discovery, 7(3), 255-270.
[19] Mok, T. S., Wu, Y. L., Thongprasert, S., Yang, C. H., Chu, D. T., Saijo, N., … & Fukuoka, M. (2009). Gefitinib or carboplatin–paclitaxel in pulmonary adenocarcinoma. New England Journal of Medicine, 361(10), 947-957.
[20] Jameson, J. L., & Longo, D. L. (2015). Precision medicine—personalized, problematic, and promising. New England Journal of Medicine, 372(23), 2229-2234.
[21] Hurwitz, H., Fehrenbacher, L., Novotny, W., Cartwright, T., Hainsworth, J., Heim, W., … & Kabbinavar, F. (2004). Bevacizumab plus irinotecan, fluorouracil, and leucovorin for metastatic colorectal cancer. New England Journal of Medicine, 350(23), 2335-2342.
[22] Sharma, P., Allison, J. P., Brahmer, J. R., Narayan, S., Tang, J., de Oliveira, M. L., … & Wargo, J. A. (2015). Immune-related adverse events associated with immune checkpoint blockade. Clinical Cancer Research, 21(22), 4439-4446.
[23] Hanahan, D., & Weinberg, R. A. (2011). Hallmarks of cancer: the next generation. Cell, 144(5), 646-674.
[24] Kourou, K., Exarchos, T. P., Exarchos, K. P., Karamouzis, M. V., & Fotiadis, D. I. (2015). Machine learning applications in cancer prognosis and prediction. Computational and Structural Biotechnology Journal, 13, 8-17.
Targeted therapies sound fantastic, but what happens when the cancer cells decide to play hide-and-seek with the markers you’re targeting? Do we need a bloodhound for cancer now?
That’s a fantastic point! Resistance is a real challenge with targeted therapies. Researchers are exploring strategies to overcome this, including combination therapies that hit cancer cells through multiple pathways and developing new drugs that target different markers or even the tumor microenvironment. It’s definitely an ongoing hunt!
Editor: MedTechNews.Uk
Thank you to our Sponsor Esdebe
The discussion on personalized medicine is critical. Utilizing genomic profiling to predict treatment outcomes and tailor chemotherapy regimens holds immense potential for minimizing toxicity and maximizing efficacy.
Thank you for highlighting the critical role of personalized medicine! Genomic profiling is indeed paving the way for more tailored chemotherapy. Imagine a future where treatment plans are as unique as our individual genetic makeup, minimizing side effects and dramatically improving outcomes. Let’s continue exploring these possibilities!
Editor: MedTechNews.Uk
Thank you to our Sponsor Esdebe