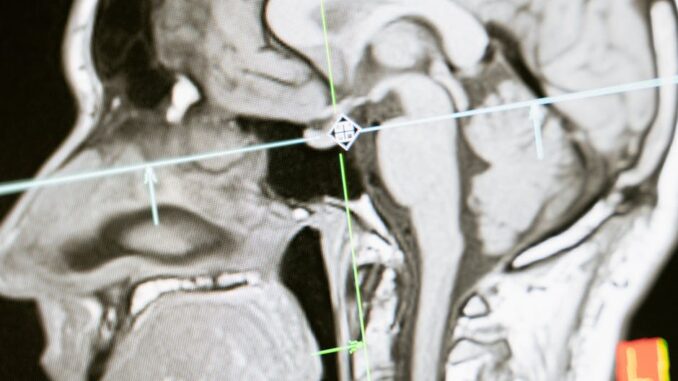
Concussion: Evolving Diagnostic and Therapeutic Strategies Across the Lifespan
Abstract
Concussion, a mild traumatic brain injury (mTBI), presents a significant public health challenge, affecting individuals of all ages with varying degrees of severity and recovery trajectories. While often considered a transient neurological disturbance, concussions can lead to persistent symptoms and long-term sequelae, especially in vulnerable populations such as children and adolescents. This research report provides a comprehensive overview of current understanding regarding concussion, encompassing its pathophysiology, diagnostic modalities, and treatment strategies. It critically examines the challenges associated with concussion management, focusing on advancements in neuroimaging, the complexities of pediatric concussion, the role of genetics and pre-existing conditions, and the comparative effectiveness of different treatment protocols. Finally, the report identifies key areas for future research to improve concussion diagnosis, management, and ultimately, patient outcomes across the lifespan.
1. Introduction
Concussion, defined as a traumatically induced transient disturbance of brain function, remains a complex and evolving area of research and clinical practice. It is estimated that millions of concussions occur annually worldwide, resulting from a variety of mechanisms, including falls, motor vehicle accidents, and sports-related injuries [1]. While the majority of individuals recover within a few weeks, a significant subset experiences prolonged symptoms and functional impairments, a condition often referred to as post-concussion syndrome (PCS) [2]. This heterogeneity in recovery underscores the need for a more nuanced understanding of concussion pathophysiology, improved diagnostic capabilities, and personalized treatment approaches.
This report will delve into the latest advancements in concussion research, spanning from the molecular level to clinical practice. It will explore the neuropathological mechanisms underlying concussion, the evolving landscape of diagnostic tools (including advanced neuroimaging techniques), and the comparative effectiveness of various treatment strategies. Special attention will be given to the unique challenges presented by pediatric concussions and the potential role of genetic and pre-existing conditions in influencing recovery outcomes.
2. Pathophysiology of Concussion: A Multifaceted Perspective
The pathophysiology of concussion is complex and multifactorial, involving a cascade of neurochemical, metabolic, and structural alterations within the brain [3]. At the initial impact, mechanical forces induce neuronal depolarization, resulting in the release of excitatory neurotransmitters such as glutamate. This excitotoxicity can lead to neuronal dysfunction and damage. Subsequently, there is a disruption of ionic homeostasis, particularly involving potassium and calcium channels, leading to metabolic crisis and reduced cerebral blood flow [4].
Emerging research highlights the role of axonal injury in concussion. Diffuse axonal injury (DAI), characterized by stretching and shearing of axons, is a common finding in concussion and is believed to contribute to long-term cognitive deficits [5]. Furthermore, neuroinflammation plays a critical role in the secondary injury phase following concussion. Activated microglia and astrocytes release pro-inflammatory cytokines, which can exacerbate neuronal damage and impair recovery processes [6].
The contribution of vascular dysfunction to concussion pathophysiology is also gaining increasing attention. Disruption of the blood-brain barrier (BBB) can occur following concussion, leading to increased permeability and infiltration of inflammatory molecules into the brain parenchyma [7]. This BBB disruption may contribute to the development of cerebral edema and further exacerbate neuronal damage.
It is crucial to acknowledge that the precise sequence and relative contribution of these pathophysiological events may vary depending on the severity of the impact, individual susceptibility factors, and time elapsed since the injury. Future research should focus on further elucidating the temporal dynamics of these processes to identify potential therapeutic targets for mitigating the long-term effects of concussion.
3. Advancements in Diagnostic Techniques
Diagnosing concussion accurately and objectively remains a significant challenge. Current diagnostic approaches rely primarily on clinical assessment, including symptom evaluation, cognitive testing, and neurological examination [8]. However, these methods are subjective and can be influenced by factors such as pre-existing conditions, medications, and effort levels.
3.1. Neuroimaging Techniques:
Neuroimaging techniques are increasingly being used to aid in concussion diagnosis and management. Conventional structural imaging, such as computed tomography (CT) and magnetic resonance imaging (MRI), are primarily used to rule out more severe brain injuries, such as hematomas or fractures. However, these modalities often appear normal in patients with concussion [9].
Advanced neuroimaging techniques, such as diffusion tensor imaging (DTI), functional MRI (fMRI), and magnetic resonance spectroscopy (MRS), offer greater sensitivity in detecting subtle brain abnormalities associated with concussion [10]. DTI can detect alterations in white matter microstructure, reflecting axonal injury. fMRI can assess changes in brain activity patterns during cognitive tasks or at rest. MRS can measure the concentration of various metabolites in the brain, providing insights into metabolic dysfunction.
3.2. Biomarkers:
The identification of reliable biomarkers for concussion diagnosis and prognosis has been a major focus of research. Several blood-based biomarkers, including glial fibrillary acidic protein (GFAP), ubiquitin C-terminal hydrolase-L1 (UCH-L1), and S100B, have shown promise in detecting acute concussion [11]. However, the sensitivity and specificity of these biomarkers can vary depending on the time elapsed since the injury and the severity of the concussion.
Other potential biomarkers include those derived from cerebrospinal fluid (CSF) and saliva. CSF biomarkers may provide a more direct measure of brain injury, while salivary biomarkers offer a less invasive approach [12]. Further research is needed to validate these biomarkers and determine their clinical utility in concussion management.
3.3. Digital Technologies:
Digital technologies, such as wearable sensors and mobile apps, are emerging as valuable tools for concussion assessment and monitoring [13]. Wearable sensors can track head impact biomechanics and provide real-time alerts for potentially concussive events. Mobile apps can be used to administer cognitive tests and monitor symptom changes over time. These technologies offer the potential to improve concussion detection, facilitate early intervention, and track recovery progress.
The integration of multiple diagnostic modalities, including clinical assessment, neuroimaging, biomarkers, and digital technologies, holds the promise of providing a more comprehensive and objective evaluation of concussion. This multi-modal approach may lead to more accurate diagnoses, improved risk stratification, and personalized treatment plans.
4. Pediatric Concussion: Unique Challenges and Considerations
Concussions in children and adolescents present unique challenges due to the ongoing development of the brain [14]. Pediatric concussions often result in longer recovery times and a higher risk of persistent symptoms compared to adults [15]. Furthermore, the developing brain may be more vulnerable to the long-term effects of concussion.
4.1. Extended Recovery Times:
Several factors may contribute to the extended recovery times observed in pediatric concussions. The immature brain may be more susceptible to axonal injury and metabolic dysfunction following concussion. Additionally, children and adolescents may have difficulty articulating their symptoms, leading to delayed diagnosis and treatment. The psychosocial impact of concussion, including academic stress and social isolation, can also contribute to prolonged recovery [16].
4.2. Neurocognitive and Behavioral Impacts:
Pediatric concussions can have significant impacts on neurocognitive and behavioral functioning. Common cognitive deficits include impairments in attention, memory, and executive functions [17]. Behavioral changes, such as irritability, anxiety, and depression, are also frequently observed [18]. These cognitive and behavioral changes can interfere with academic performance, social interactions, and overall quality of life.
4.3. Management Approaches:
Management of pediatric concussions requires a multidisciplinary approach involving physicians, neuropsychologists, physical therapists, and school personnel [19]. Rest, both physical and cognitive, is a cornerstone of concussion management. However, prolonged rest can have negative consequences, such as deconditioning and social isolation. Therefore, a gradual return-to-activity protocol is recommended, guided by symptom monitoring and neurocognitive testing [20].
Special considerations are required for managing pediatric concussions in specific populations, such as student-athletes and children with pre-existing conditions. Return-to-play decisions should be made on an individualized basis, considering the severity of the concussion, the athlete’s symptoms, and the risk of further injury. Children with pre-existing conditions, such as learning disabilities or mental health disorders, may require more intensive and specialized interventions.
5. The Role of Genetics and Pre-Existing Conditions in Concussion Recovery
The variability in concussion recovery outcomes suggests that genetic and pre-existing conditions may play a significant role [21]. Certain genetic polymorphisms may predispose individuals to increased susceptibility to concussion or prolonged recovery times. For example, genes involved in neurotransmitter metabolism, inflammation, and neuroplasticity may influence concussion outcomes [22].
Pre-existing conditions, such as attention-deficit/hyperactivity disorder (ADHD), learning disabilities, and mental health disorders, have been associated with increased risk of concussion and prolonged recovery [23]. These conditions may compromise the brain’s ability to adapt to injury and recover effectively. Furthermore, pre-existing conditions can complicate the assessment and management of concussion, as symptoms may overlap or be exacerbated.
Understanding the interplay between genetics, pre-existing conditions, and concussion recovery is crucial for developing personalized treatment strategies. Future research should focus on identifying specific genetic markers and pre-existing conditions that predict concussion outcomes. This knowledge can inform risk stratification and guide the selection of appropriate interventions.
6. Comparative Effectiveness of Different Treatment Protocols
Numerous treatment protocols have been proposed for managing concussion, ranging from rest and rehabilitation to pharmacological interventions [24]. However, the comparative effectiveness of these protocols remains a topic of ongoing research and debate.
6.1. Rest and Rehabilitation:
Rest, both physical and cognitive, is a fundamental component of concussion management. However, the optimal duration and intensity of rest are not well-defined. Recent evidence suggests that prolonged strict rest may be detrimental and that early, graded activity may promote recovery [25].
Rehabilitation therapies, such as physical therapy, occupational therapy, and speech therapy, can address specific functional impairments associated with concussion. Physical therapy can improve balance, coordination, and vestibular function. Occupational therapy can address cognitive and visual impairments. Speech therapy can improve communication and swallowing difficulties.
6.2. Pharmacological Interventions:
Several pharmacological interventions have been investigated for managing concussion symptoms, such as headaches, sleep disturbances, and mood disorders [26]. However, there is limited evidence to support the use of specific medications for concussion. Furthermore, the potential side effects of these medications must be carefully considered, especially in children and adolescents.
6.3. Emerging Therapies:
Emerging therapies for concussion include transcranial magnetic stimulation (TMS), neurofeedback, and hyperbaric oxygen therapy (HBOT) [27]. TMS involves non-invasive stimulation of the brain using magnetic pulses. Neurofeedback involves training individuals to self-regulate their brain activity patterns. HBOT involves breathing pure oxygen in a pressurized chamber. While these therapies have shown some promise in preliminary studies, further research is needed to determine their efficacy and safety.
The selection of appropriate treatment protocols should be individualized, based on the patient’s symptoms, medical history, and response to previous interventions. A multidisciplinary approach involving physicians, therapists, and other healthcare professionals is essential for optimizing treatment outcomes. Furthermore, ongoing monitoring and adjustments to the treatment plan are necessary to ensure that the patient is progressing towards recovery.
7. Future Directions and Research Priorities
Concussion research is a rapidly evolving field, with many unanswered questions and opportunities for future investigation. Key areas for future research include:
- Improving concussion diagnosis: Developing more sensitive and objective diagnostic tools, including advanced neuroimaging techniques and biomarkers, is crucial for improving concussion detection and risk stratification.
- Understanding the long-term effects of concussion: Longitudinal studies are needed to investigate the long-term cognitive, emotional, and behavioral consequences of concussion, especially in vulnerable populations such as children and athletes.
- Developing personalized treatment strategies: Identifying genetic and pre-existing factors that influence concussion recovery is essential for tailoring treatment plans to individual patients.
- Evaluating the effectiveness of different treatment protocols: Randomized controlled trials are needed to compare the efficacy of various treatment strategies, including rest, rehabilitation, pharmacological interventions, and emerging therapies.
- Developing strategies for concussion prevention: Implementing evidence-based strategies for concussion prevention, such as helmet use and rule changes in sports, can reduce the incidence and severity of concussions.
8. Conclusion
Concussion remains a complex and challenging condition that requires a multidisciplinary approach to diagnosis and management. Advances in neuroimaging, biomarker discovery, and digital technologies are providing new insights into the pathophysiology of concussion and improving diagnostic capabilities. Special considerations are required for managing pediatric concussions, given the unique vulnerability of the developing brain. Understanding the role of genetics and pre-existing conditions in concussion recovery is crucial for developing personalized treatment strategies. Future research should focus on addressing the remaining knowledge gaps and developing more effective strategies for concussion prevention, diagnosis, and treatment across the lifespan.
References
[1] Gardner, A. J., & Veggeberg, R. (2017). Concussion. BMJ: British Medical Journal, 357, j1527.
[2] McCrory, P., Meeuwisse, W. H., Dvorak, J., Aubry, M., Cantu, R. C., Drezner, J. A., … & Vos, P. E. (2017). Consensus statement on concussion in sport—the 5th international conference on concussion in sport held in Berlin, October 2016. British Journal of Sports Medicine, 51(11), 838-847.
[3] Giza, C. C., & Hovda, D. A. (2014). The neurometabolic cascade of concussion. Journal of Athletic Training, 36(3), 228.
[4] TBI Model Systems Knowledge Translation Center. (2022). Understanding TBI: What Happens to the Brain During and After a TBI. https://msktc.org/tbi/factsheets/understanding-tbi
[5] Blennow, K., Hardy, J., & Zetterberg, H. (2012). The neuropathology and neurobiology of traumatic brain injury. Neuron, 76(5), 886-899.
[6] Kumar, A., Loane, D. J., Stoica, B. A., Zhang, J., Faden, A. I. (2016). Acute neuroinflammation after traumatic brain injury. Trends in Neurosciences, 39(8), 521-534.
[7] Garza, D., Campbell, A. I., Moore, C. M., et al. Blood-brain barrier disruption and the microvascular response to traumatic brain injury. J Neurotrauma. 2020;37(1):1-14.
[8] Broglio, S. P., Macciocchi, S. N., Ferrara, M. S. (2007). Neurocognitive performance of concussed athletes when symptom free. Journal of Athletic Training, 42(4), 503.
[9] Lipton, M. L., & Dietch, J. M. (2015). Conventional MRI in mild traumatic brain injury. Handbook of Clinical Neurology, 128, 299-318.
[10] Churchill, N. W., Hutchison, M. G., Richards, D., Graham, S. J., & Schweizer, T. A. (2017). Resting-state fMRI in acute sport-related concussion. Journal of Neurotrauma, 34(12), 2043-2053.
[11] Shahim, P., Tegner, Y., Gustafsson, L., Olsson, R. T., Weibull, A., Nellgard, B., … & Zetterberg, H. (2016). Plasma biomarkers in acute sport-related concussion. Neurology, 87(3), 254-262.
[12] Gill, J. M., Bazarian, J. J., Vassar, M. J., & Zetterberg, H. (2015). Cerebrospinal fluid biomarkers of traumatic brain injury: a systematic review. PloS One, 10(8), e0133864.
[13] Allen, M., Smirl, J. D., Gandevia, S. C., & Middleton, J. W. (2021). The use of wearable sensors for concussion diagnosis and management: A systematic review. Sensors, 21(3), 872.
[14] Kirkwood, M. W., Yeates, K. O., & Wilson, P. E. (2006). Pediatric traumatic brain injury: age-related differences in injury mechanism, severity, and outcome. Developmental Neuropsychology, 29(1), 1-20.
[15] Bloom, J. E., Fischer, L., & Manasco, A. (2019). Pediatric concussion: A review. Missouri Medicine, 116(4), 301.
[16] Elbin, R. J., Kontos, A. P., Collins, M. W., Stearns, R. L., Maroon, J. C., & Lovell, M. R. (2011). A repeated measures examination of symptom severity and neurocognitive performance in concussed athletes. The American Journal of Sports Medicine, 39(12), 2733-2741.
[17] Gioia, G. A., Collins, M. W., & Isquith, P. K. (2004). Improving identification of mild traumatic brain injury in children and adolescents. The Clinical Neuropsychologist, 18(3), 453-471.
[18] Barlow, K. M., Crawford, S., Stevenson, A., & Waddell, B. (2010). The effect of pediatric mild traumatic brain injury on behavior and emotional functioning: A systematic review. The Journal of Head Trauma Rehabilitation, 25(2), 149-162.
[19] Halstead, M. E., Walter, K. D., Moffatt, K. E., American Academy of Pediatrics Council on Sports Medicine and Fitness. (2013). Clinical report—sport-related concussion in children and adolescents. Pediatrics, 132(5), 957-965.
[20] Thomas, D. G., Apps, J. N., Comper, P., Gioia, G. A., &ровос J. A. (2015). Management of concussion in sport: recommendations from the 4th International Consensus Conference on Concussion in Sport, Zurich 2012. British Journal of Sports Medicine, 49(23), 1537-1538.
[21] Iverson, G. L. (2018). Predictors of poor outcome following mild traumatic brain injury: A systematic review. Brain Injury, 20(13-14), 1259-1283.
[22] Ramage, J. L., Vella, A., & Schneider, K. J. (2021). Genetic contributions to concussion outcomes: A systematic review. Frontiers in Neurology, 12, 655547.
[23] Covassin, T., Elbin, R. J., Harris, W., Parker, T., & Kontos, A. P. (2012). The role of pre-existing attention-deficit/hyperactivity disorder on initial symptoms and recovery following sport-related concussion. The American Journal of Sports Medicine, 40(6), 1299-1304.
[24] Leddy, J. J., & Willer, B. (2013). Use of an exercise treadmill test in assessing exertional tolerance and predicting the recovery of sport-related concussion in adolescents. Journal of Athletic Training, 48(4), 514-520.
[25] Thomas, D. G., Apps, J. N., Comper, P., Gioia, G. A., &ровос J. A. (2015). Management of concussion in sport: recommendations from the 4th International Consensus Conference on Concussion in Sport, Zurich 2012. British Journal of Sports Medicine, 49(23), 1537-1538.
[26] Prakash, S., Hosseini, A., Kumar, A., Sharma, S., Graham, S. H., & Signore, A. P. (2014). Pharmacological interventions for the treatment of traumatic brain injury. Frontiers in Neurology, 5, 218.
[27] Larson-Prior, L. J., Simpson, R., Desmond, J. E., & Riedy, G. (2017). Nonpharmacological interventions for chronic traumatic brain injury: a systematic review. Journal of Rehabilitation Research & Development, 54(4), 317.
This report’s discussion of digital technologies for concussion assessment is particularly compelling. I wonder if the data collected from wearable sensors and mobile apps could be integrated with machine learning algorithms to predict recovery trajectories more accurately.
That’s a fantastic point! Integrating wearable sensor data with machine learning could really revolutionize how we predict concussion recovery. Imagine personalized recovery plans based on real-time data analysis. It opens up exciting possibilities for more targeted interventions and improved patient outcomes. Thanks for sparking this important discussion!
Editor: MedTechNews.Uk
Thank you to our Sponsor Esdebe