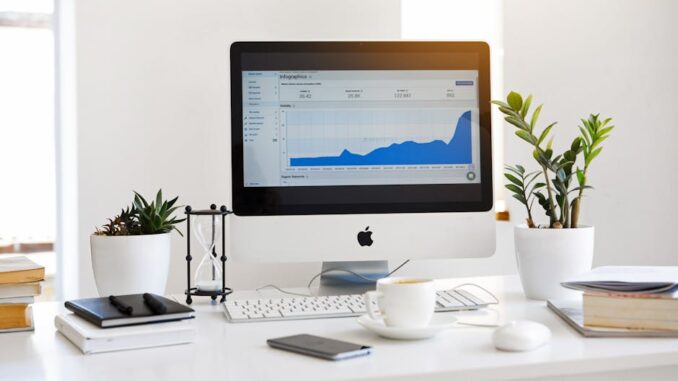
Continuous Monitoring Technologies: Beyond Glucose and Towards Holistic Physiological Profiling
Abstract
Continuous monitoring technologies (CMTs), initially pioneered for glucose management in diabetes, are undergoing a significant evolution, expanding their scope to encompass a broader array of physiological parameters. This report provides a comprehensive overview of the current state and future trajectory of CMTs, moving beyond the well-established field of continuous glucose monitoring (CGM) to explore emerging applications in areas such as lactate, ketones, vital signs, and even electrophysiological signals. We delve into the underlying principles of these technologies, examining both invasive and non-invasive methodologies, and discuss the challenges and opportunities associated with their development and deployment. Furthermore, we analyze the potential impact of holistic physiological profiling on personalized medicine, disease prevention, and athletic performance, emphasizing the need for robust data analytics, integration with artificial intelligence (AI), and careful consideration of ethical and privacy implications. This report aims to provide experts in the field with a forward-looking perspective on the transformative potential of CMTs and the key areas that warrant further research and innovation.
1. Introduction
The advent of continuous glucose monitoring (CGM) systems has revolutionized diabetes management, offering individuals with diabetes real-time insights into their glucose levels and facilitating more precise insulin dosing [1]. The success of CGM technology has spurred interest in developing continuous monitoring devices for other physiological parameters, driven by the recognition that continuous data streams provide a more comprehensive and dynamic picture of an individual’s health status than intermittent measurements. This is particularly relevant in the context of chronic diseases, where subtle changes in physiological parameters can precede the onset of acute events. Furthermore, continuous monitoring enables personalized interventions tailored to an individual’s unique physiological profile, potentially improving treatment efficacy and patient outcomes. The purpose of this report is to provide a review of current and future CMTs, expanding beyond the focus on CGM. We will examine the technological underpinnings of various CMTs, including their strengths, limitations, and potential applications, as well as the challenges that must be addressed to realize their full potential.
2. Technological Foundations of Continuous Monitoring
CMTs rely on a diverse range of sensing modalities to continuously measure physiological parameters. These modalities can be broadly classified as invasive, minimally invasive, and non-invasive. Each approach has its own advantages and disadvantages in terms of accuracy, stability, biocompatibility, and patient comfort.
2.1 Invasive and Minimally Invasive Methods
Invasive CMTs typically involve the insertion of a sensor directly into the bloodstream or interstitial fluid (ISF). These methods often provide the most accurate and reliable measurements, but they also carry a higher risk of infection, inflammation, and discomfort. Minimally invasive methods, such as CGM sensors that are inserted subcutaneously, represent a compromise between accuracy and patient burden. CGM sensors generally employ electrochemical biosensors that detect glucose in the ISF, generating an electrical signal proportional to the glucose concentration [2]. The signal is then processed and transmitted wirelessly to a receiver or smartphone. Other minimally invasive sensors are being developed for continuous monitoring of electrolytes, metabolites (e.g., lactate, ketones), and even specific proteins or biomarkers. These sensors often utilize similar electrochemical principles or optical techniques, such as fluorescence or surface plasmon resonance (SPR), to detect the target analyte. The challenge here lies in ensuring long-term sensor stability and biocompatibility, as well as minimizing the inflammatory response at the sensor-tissue interface. This is generally addressed by various biocompatible coatings, often based on hydrogels and polymers, which both reduce inflammation and also improve the signal to noise ratio.
2.2 Non-Invasive Methods
Non-invasive CMTs offer the advantage of being completely painless and risk-free, making them more appealing to patients and suitable for long-term monitoring. However, non-invasive methods often face significant challenges in terms of accuracy, sensitivity, and specificity. Some examples of non-invasive CMTs include:
- Transdermal Sensors: These sensors use techniques such as reverse iontophoresis or sonophoresis to extract analytes from the skin for measurement [3]. While promising, the amount of analyte extracted is often very low, requiring highly sensitive detection methods. Furthermore, the accuracy of transdermal measurements can be affected by skin hydration, temperature, and other factors.
- Optical Sensors: Optical sensors, such as near-infrared spectroscopy (NIRS), can non-invasively measure the concentration of certain analytes in the blood or tissue by analyzing the absorption and scattering of light [4]. NIRS has been used to monitor oxygen saturation, cerebral blood flow, and, in some cases, glucose levels. However, the accuracy of NIRS measurements can be affected by tissue thickness, skin pigmentation, and other physiological factors. One of the primary challenges of NIRS for glucose sensing is the relatively low absorption coefficient of glucose in the near-infrared spectrum, which makes it difficult to differentiate glucose signals from background noise.
- Wearable Sensors: Wearable sensors, such as wristbands or patches, can continuously monitor vital signs such as heart rate, respiratory rate, skin temperature, and activity levels [5]. These sensors typically employ accelerometers, gyroscopes, and photoplethysmography (PPG) to collect data. While wearable sensors can provide valuable insights into an individual’s overall health status, their accuracy can be affected by movement artifacts, skin contact, and other factors. Furthermore, many wearable sensors rely on proprietary algorithms to process the data, which can make it difficult to compare results across different devices.
2.3. Emerging Sensing Technologies
Research continues into novel sensing technologies. Microneedle based sensors allow for almost pain free access to the ISF for more accurate measurements [6]. These can also be combined with microfluidic channels to facilitate continuous extraction and measurement of analytes. Another area of interest is breath analysis, which could provide a non-invasive method for monitoring volatile organic compounds (VOCs) that are indicative of certain diseases or metabolic states [7].
3. Expanding the Scope of Continuous Monitoring: Beyond Glucose
While CGM has paved the way for continuous monitoring, the technology is now being extended to monitor a wider range of physiological parameters. This expansion holds the potential to revolutionize personalized medicine and disease management.
3.1 Continuous Lactate Monitoring
Lactate is a key indicator of tissue hypoxia and metabolic stress. Continuous lactate monitoring can be valuable in critical care settings, where it can help to identify patients at risk of developing sepsis or other life-threatening conditions [8]. It is also useful in sports performance for optimizing training and preventing overtraining. Several companies are developing continuous lactate sensors based on electrochemical or optical principles. These sensors can be implanted subcutaneously or integrated into wearable devices. However, challenges remain in ensuring the accuracy and stability of lactate sensors, particularly in the presence of other interfering substances in the ISF.
3.2 Continuous Ketone Monitoring
Ketones are produced when the body breaks down fat for energy, and elevated ketone levels can be indicative of diabetic ketoacidosis (DKA) or other metabolic disorders. Continuous ketone monitoring can be particularly useful for individuals with type 1 diabetes, who are at risk of developing DKA. Several companies are developing continuous ketone sensors based on electrochemical or optical principles. These sensors can be integrated into CGM devices or worn as standalone devices. The integration of ketone monitoring with CGM systems offers the potential for more comprehensive diabetes management, enabling individuals to detect and respond to early signs of DKA [9].
3.3 Continuous Vital Signs Monitoring
Continuous monitoring of vital signs, such as heart rate, respiratory rate, blood pressure, and body temperature, is essential for early detection of disease and monitoring treatment effectiveness. Wearable sensors are increasingly being used to continuously monitor vital signs, providing valuable data for personalized healthcare. These devices typically use PPG to measure heart rate and pulse oximetry to measure oxygen saturation. More advanced devices may also incorporate accelerometers and gyroscopes to monitor activity levels and sleep patterns. Continuous blood pressure monitoring remains a challenge, but several companies are developing cuffless blood pressure sensors based on oscillometric or tonometric principles [10].
3.4 Continuous Electrophysiological Monitoring
Continuous monitoring of electrophysiological signals, such as electrocardiogram (ECG) and electroencephalogram (EEG), can provide valuable insights into cardiovascular and neurological function. Wearable ECG monitors are increasingly being used to detect arrhythmias and other heart conditions [11]. These devices typically use electrodes that are placed on the chest or wrists to record the electrical activity of the heart. Continuous EEG monitoring can be used to diagnose and manage epilepsy, sleep disorders, and other neurological conditions. However, continuous EEG monitoring typically requires the placement of electrodes on the scalp, which can be uncomfortable and inconvenient.
4. Integration with Other Health Tools and Artificial Intelligence
CMTs generate vast amounts of data, which can be challenging to interpret and utilize effectively. Integrating CMT data with other health tools, such as electronic health records (EHRs), mobile health (mHealth) apps, and telehealth platforms, can facilitate data sharing and collaboration among patients, healthcare providers, and researchers. Furthermore, artificial intelligence (AI) and machine learning (ML) algorithms can be used to analyze CMT data and identify patterns, predict future events, and personalize interventions [12]. For example, AI algorithms can be used to predict glucose fluctuations based on CGM data, insulin doses, and meal intake. These predictions can then be used to optimize insulin dosing and prevent hypoglycemia or hyperglycemia. AI algorithms can also be used to analyze vital signs data and detect early signs of infection or other medical conditions. The integration of CMTs with AI and other health tools holds the potential to transform healthcare from a reactive to a proactive and personalized approach.
5. Closed-Loop Insulin Delivery Systems (Artificial Pancreas)
One of the most promising applications of CGM technology is in closed-loop insulin delivery systems, also known as artificial pancreas systems. These systems automatically adjust insulin delivery based on real-time glucose levels, eliminating the need for manual insulin injections or boluses [13]. Artificial pancreas systems typically consist of a CGM sensor, an insulin pump, and a control algorithm that links the two devices. The control algorithm analyzes the CGM data and calculates the appropriate insulin dose, which is then delivered by the insulin pump. Several artificial pancreas systems have been approved by regulatory agencies and are now commercially available. These systems have been shown to improve glycemic control, reduce the risk of hypoglycemia, and improve quality of life for individuals with type 1 diabetes [14]. Future artificial pancreas systems may incorporate additional sensors, such as ketone sensors, to further optimize insulin delivery and prevent DKA.
6. Impact on Patient Outcomes and Healthcare Costs
CMTs have been shown to improve patient outcomes and reduce healthcare costs in several clinical areas. In diabetes management, CGM has been shown to improve glycemic control, reduce the risk of hypoglycemia, and improve quality of life [15]. Studies have also shown that CGM can reduce healthcare costs by reducing the need for hospitalizations and emergency room visits. Continuous vital signs monitoring has been shown to improve outcomes in patients with chronic heart failure, by enabling early detection of deterioration and preventing hospitalizations [16]. CMTs can also be used to monitor patients remotely, reducing the need for in-person visits and improving access to care. The widespread adoption of CMTs has the potential to significantly improve the efficiency and effectiveness of healthcare delivery.
7. Challenges and Future Directions
Despite the significant progress made in CMT technology, several challenges remain. These include:
- Accuracy and Reliability: CMTs need to be highly accurate and reliable to provide meaningful data for clinical decision-making. Further research is needed to improve the accuracy of existing sensors and develop new sensing technologies with improved performance characteristics.
- Biocompatibility and Longevity: CMTs need to be biocompatible and long-lasting to minimize the risk of inflammation and infection. Further research is needed to develop new biocompatible materials and sensor designs that can withstand the harsh environment of the human body.
- Data Security and Privacy: CMTs generate sensitive personal data that needs to be protected from unauthorized access. Robust security measures are needed to ensure the privacy and confidentiality of patient data.
- Data Integration and Interoperability: CMT data needs to be seamlessly integrated with other health tools and systems to facilitate data sharing and collaboration. Standards are needed to ensure the interoperability of different CMT devices and platforms.
- Cost and Accessibility: CMTs need to be affordable and accessible to all individuals who could benefit from them. Efforts are needed to reduce the cost of CMTs and expand access to these technologies in underserved communities.
Future research directions in CMTs include:
- Development of new sensing technologies: Research is ongoing to develop new sensing technologies that can measure a wider range of physiological parameters with improved accuracy and reliability.
- Integration of AI and ML: AI and ML algorithms are being developed to analyze CMT data and provide personalized insights and recommendations.
- Development of closed-loop systems: Research is ongoing to develop closed-loop systems that can automatically adjust medication doses or other interventions based on real-time physiological data.
- Expansion of CMT applications: CMTs are being explored for use in a wide range of clinical areas, including diabetes, cardiovascular disease, neurological disorders, and infectious diseases.
- Focus on preventative health: As technology improves, these devices will become more widely available for preventative health. For example, monitoring lactate and glucose in athletes to improve their performance and health.
8. Ethical and Societal Implications
The widespread adoption of CMTs raises several ethical and societal implications that need to be carefully considered. These include:
- Data ownership and control: Who owns the data generated by CMTs, and who has the right to access and use it? Patients need to have control over their own data and the ability to decide how it is used.
- Privacy and security: How can we ensure the privacy and security of sensitive personal data generated by CMTs? Robust security measures are needed to protect data from unauthorized access and misuse.
- Algorithmic bias: How can we ensure that AI algorithms used to analyze CMT data are fair and unbiased? Algorithmic bias can lead to inaccurate or unfair predictions, which can have serious consequences for patients.
- Digital divide: How can we ensure that all individuals have access to CMTs, regardless of their socioeconomic status or geographic location? Efforts are needed to address the digital divide and ensure that CMTs are accessible to all who could benefit from them.
- Over-reliance and deskilling: There is a risk that clinicians may become over-reliant on CMT data and deskilled in traditional diagnostic methods. It is important to maintain a balance between technology and clinical judgment.
9. Conclusion
Continuous monitoring technologies are transforming healthcare by providing real-time insights into an individual’s physiological status. While CGM has paved the way for continuous monitoring, the technology is now being extended to monitor a wider range of physiological parameters, including lactate, ketones, vital signs, and electrophysiological signals. The integration of CMTs with other health tools and AI holds the potential to revolutionize personalized medicine, disease prevention, and athletic performance. However, several challenges remain, including accuracy, biocompatibility, data security, and ethical considerations. By addressing these challenges and investing in further research and innovation, we can unlock the full potential of CMTs and improve the health and well-being of individuals worldwide. The future lies in the development of holistic physiological profiling systems that provide a comprehensive and dynamic picture of an individual’s health status, enabling personalized interventions and proactive disease management.
References
[1] Heinemann, L., & Freckmann, G. (2015). Continuous glucose monitoring in people with diabetes: a perspective on its current use and future potential. Diabetic Medicine, 32(3), 284-293.
[2] Pickup, J. C. (2012). Developments in glucose biosensors–a review. Biosensors and Bioelectronics, 26(10), 3699-3700.
[3] Prausnitz, M. R., Mitragotri, S., & Langer, R. (2004). Transdermal drug delivery. Nature Reviews Drug Discovery, 3(2), 115-126.
[4] Burns, D. A., & Ciurczak, E. W. (2007). Handbook of near-infrared analysis. CRC press.
[5] Patel, S., Park, H., Bonato, P., Chan, L., & Rodgers, M. (2012). A review of wearable sensors and systems with application in rehabilitation. Journal of Neuroengineering and Rehabilitation, 9(1), 21.
[6] Roxhed, N., Samel, B., Nordquist, L., Griss, P., & Stemme, G. (2008). Porous silicon microneedles for transdermal drug delivery. Journal of Micromechanics and Microengineering, 18(7), 075028.
[7] Buszewski, B., Kesy, M., Ligor, T., & Amann, A. (2007). Human exhaled air analytics: biomarkers of diseases. Biomedical Chromatography, 21(6), 553-566.
[8] Bakker, J., Nijsten, M. W., Jansen, T. C., Hayes, M. A., Veenstra, G., van Waardenburg, D. A., … & Oudemans-van Straaten, H. M. (2013). Clinical use of lactate monitoring in critically ill patients. Annals of Intensive Care, 3(1), 12.
[9] Singh, P., Anjum, D., & Mandal, S. K. (2022). Continuous ketone monitoring for diabetes management: A review of current technologies and future perspectives. Journal of Diabetes Science and Technology, 16(6), 1382-1392.
[10] Elgendi, M., Fletcher, R., Liang, Y., Howard, N., Lovell, N. H., Abbott, D., & Lim, K. (2017). The use of photoplethysmography for assessing hypertension. IEEE Reviews in Biomedical Engineering, 10, 62-73.
[11] Perez, M. V., Mahaffey, K. W., Quinto, K., Wyatt, K. D., Whooley, M. A. (2019). Large-scale assessment of a smartwatch to identify atrial fibrillation. New England Journal of Medicine, 381(20), 1909-1917.
[12] Lonini, L., Mainardi, L., Magenes, G., & Vanzulli, L. (2020). Machine learning in continuous glucose monitoring (CGM): a systematic review. Journal of Diabetes Science and Technology, 14(3), 533-545.
[13] Bekiari, E., Kitsios, K., Tsalikian, E., & Ghanim, M. T. (2018). Artificial pancreas: current status and future perspectives. Journal of Diabetes Science and Technology, 12(1), 21-34.
[14] Bergenstal, R. M., Nimri, R., Beck, R. W., Criego, A., Laffel, L., Schatz, D., … & Weinzimer, S. A. (2016). A comparison of two hybrid closed-loop systems in adolescents and adults with type 1 diabetes. The Lancet, 388(10042), 449-458.
[15] Beck, R. W., Riddlesworth, T. D., Ruedy, K. J., Ahmann, A. J., Bergenstal, R. M., Haller, M. J., … & Tamborlane, W. V. (2017). Effect of continuous glucose monitoring on glycemic control in adults with type 1 diabetes using insulin injections: The DIAMOND randomized clinical trial. JAMA, 317(4), 371-382.
[16] Chaudhry, S. I., Mattera, J. A., Curtis, J. P., Spertus, J. A., Herrin, J., Lin, Z. Q., … & Krumholz, H. M. (2010). Telemonitoring in patients with heart failure. New England Journal of Medicine, 363(24), 2301-2309.
Holistic physiological profiling? Sounds like my Fitbit is about to get a serious promotion! Soon we’ll be diagnosing diseases before they even *think* about showing symptoms. Just imagine the possibilities… and the potential for hypochondria to reach Olympic levels! Are we ready for this brave new world of over-sharing bodily data?
That’s a great point! The potential for increased awareness *and* over-analysis is definitely something to consider. It’s important to develop tools and education to help people interpret this data responsibly and avoid unnecessary anxiety, while still leveraging the benefits for early detection and personalized care.
Editor: MedTechNews.Uk
Thank you to our Sponsor Esdebe
The discussion of ethical implications is crucial. How do we ensure equitable access to these technologies and prevent exacerbation of existing health disparities, particularly in underserved communities?
That’s such an important question! Equitable access is paramount. Perhaps subsidies or community-based programs could help bridge the gap and ensure underserved communities benefit from these advancements. We should explore collaborative solutions with healthcare providers and tech companies.
Editor: MedTechNews.Uk
Thank you to our Sponsor Esdebe