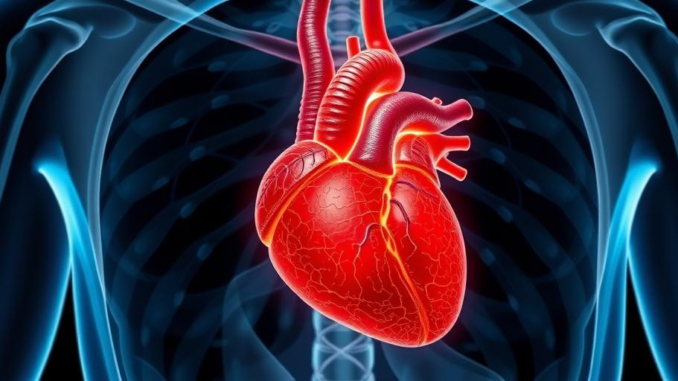
Abstract
Coronary artery disease (CAD) is a leading cause of morbidity and mortality worldwide, with percutaneous coronary intervention (PCI) utilizing coronary stents as a cornerstone of treatment. However, the presence of arterial calcification significantly hinders optimal stent deployment and increases the risk of procedural complications and adverse long-term outcomes. This report provides a comprehensive review of the challenges posed by calcified lesions during stent placement, focusing on advanced imaging modalities for lesion characterization, lesion preparation techniques including atherectomy and intravascular lithotripsy (IVL), and emerging stent technologies designed to enhance deliverability and conformability in heavily calcified vessels. Furthermore, we delve into the role of pharmacological strategies to mitigate the risk of stent thrombosis and restenosis in this high-risk patient population, and propose future research directions aimed at improving the efficacy and safety of coronary stenting in the presence of severe calcification.
Many thanks to our sponsor Esdebe who helped us prepare this research report.
1. Introduction
Percutaneous coronary intervention (PCI) with stent implantation has revolutionized the treatment of coronary artery disease (CAD), offering a less invasive alternative to bypass surgery for many patients. Stents provide mechanical support to the diseased vessel, restoring luminal diameter and improving blood flow to the myocardium. While advancements in stent technology, including the development of drug-eluting stents (DES), have significantly reduced the incidence of restenosis compared to bare-metal stents (BMS), challenges persist in specific patient subgroups and lesion subsets. One of the most significant obstacles to successful PCI is the presence of arterial calcification, which is frequently encountered in patients with advanced age, diabetes mellitus, chronic kidney disease, and prior history of CAD [1].
Arterial calcification is a complex process involving the deposition of calcium phosphate crystals within the intimal and medial layers of the arterial wall. This process leads to increased vessel stiffness, reduced compliance, and impaired vasoreactivity. In the context of PCI, calcified lesions present several challenges. First, they hinder stent delivery and expansion, potentially leading to incomplete stent apposition (ISA) and malapposition. Second, calcified plaques are more prone to fracture and dissection during balloon angioplasty or stent deployment, increasing the risk of procedural complications such as perforation and abrupt vessel closure. Third, suboptimal stent expansion and apposition in calcified lesions are associated with higher rates of stent thrombosis and restenosis, negating the benefits of DES [2].
Given the increasing prevalence of CAD and the aging of the population, the management of calcified coronary lesions has become a critical area of focus in interventional cardiology. This report aims to provide a comprehensive overview of the challenges posed by calcified coronary lesions during PCI, to discuss the current strategies for lesion preparation and stent deployment, and to explore future directions for improving outcomes in this challenging patient population. The analysis will address technological and pharmacological strategies, as well as patient-specific risk stratification.
Many thanks to our sponsor Esdebe who helped us prepare this research report.
2. Pathophysiology and Clinical Implications of Arterial Calcification
Arterial calcification is not merely a passive accumulation of calcium deposits but rather an active and highly regulated biological process that shares similarities with bone formation. It is broadly classified into two main types: intimal calcification and medial calcification. Intimal calcification, typically associated with atherosclerosis, involves the deposition of calcium phosphate crystals within the atherosclerotic plaque. This type of calcification is characterized by its irregular distribution and its association with inflammatory processes, lipid accumulation, and neovascularization [3]. Medial calcification, also known as Mönckeberg’s sclerosis, involves the deposition of calcium within the medial layer of the arterial wall. It is more commonly observed in patients with diabetes mellitus and chronic kidney disease and is characterized by its circumferential distribution and its association with smooth muscle cell dysfunction and extracellular matrix remodeling [4].
The presence of arterial calcification has significant clinical implications. Calcified lesions are more likely to be complex, with a higher plaque burden and a greater degree of stenosis. These lesions are also more resistant to balloon angioplasty and stent deployment, increasing the risk of procedural complications such as vessel dissection, perforation, and incomplete stent apposition. Furthermore, calcification reduces arterial compliance, impairing vasodilation and increasing the risk of myocardial ischemia. Studies have consistently shown that the presence of severe calcification is associated with higher rates of major adverse cardiovascular events (MACE) following PCI, including death, myocardial infarction, and target lesion revascularization (TLR) [5].
Importantly, the extent and severity of calcification can be assessed using various imaging modalities, including angiography, intravascular ultrasound (IVUS), and optical coherence tomography (OCT). Angiography is the traditional imaging modality used to assess coronary artery disease, but it has limited sensitivity for detecting calcification. IVUS provides cross-sectional images of the vessel wall, allowing for the visualization of calcified plaques and the assessment of their extent and distribution. OCT offers higher resolution imaging compared to IVUS, enabling more detailed characterization of the plaque morphology and the detection of microcalcifications [6].
Many thanks to our sponsor Esdebe who helped us prepare this research report.
3. Diagnostic Imaging for Assessing Calcified Lesions
Accurate assessment of lesion morphology, including the presence and extent of calcification, is crucial for planning PCI and selecting the appropriate strategy for lesion preparation and stent deployment. Traditional angiography has limited sensitivity for detecting calcification, often underestimating its severity. Intravascular imaging modalities, such as IVUS and OCT, provide more detailed information about the composition of the plaque and the degree of calcification. These techniques allow for a more informed decision-making process, potentially leading to improved procedural outcomes [7].
3.1 Intravascular Ultrasound (IVUS)
IVUS is a catheter-based ultrasound imaging technique that provides real-time, cross-sectional images of the vessel wall. IVUS can differentiate between soft plaque, fibrous tissue, and calcified lesions. Calcified plaques appear as echogenic regions with acoustic shadowing. IVUS can also be used to assess the extent and distribution of calcification, as well as the presence of plaque rupture and dissection. Quantitative IVUS analysis can provide measurements of the lumen area, plaque burden, and calcium arc. Studies have shown that IVUS guidance during PCI can improve stent deployment and reduce the risk of adverse events, particularly in complex lesions [8].
3.2 Optical Coherence Tomography (OCT)
OCT is a high-resolution intravascular imaging technique that uses near-infrared light to create cross-sectional images of the vessel wall. OCT provides superior image resolution compared to IVUS, allowing for more detailed visualization of plaque morphology and microstructural features. Calcified plaques appear as signal-poor regions with well-defined borders. OCT can also detect superficial calcium and calcium nodules, which may be missed by IVUS. OCT has been shown to be useful for assessing stent apposition, edge dissection, and neointimal hyperplasia following stent implantation. Several studies have demonstrated that OCT-guided PCI can improve stent optimization and reduce the risk of target lesion failure [9].
3.3 Comparison of IVUS and OCT
While both IVUS and OCT are valuable tools for assessing calcified lesions, they have different strengths and limitations. IVUS has better penetration depth and can visualize the entire vessel wall, including the adventitia. OCT has higher resolution and can provide more detailed information about plaque morphology and microstructural features. OCT requires a blood-free field of view, which may be challenging to achieve in some cases. The choice between IVUS and OCT depends on the specific clinical scenario and the information needed to guide PCI. In general, OCT is preferred for assessing stent apposition and detecting superficial calcium, while IVUS is preferred for assessing the overall plaque burden and the presence of external elastic membrane remodeling [10].
3.4 Emerging Imaging Technologies
Several emerging imaging technologies are being developed to further improve the assessment of calcified lesions. These include near-infrared spectroscopy (NIRS) for detecting lipid-rich plaques, artificial intelligence (AI)-enhanced imaging for automated plaque characterization, and hybrid imaging modalities that combine IVUS and OCT to provide comprehensive information about the vessel wall. These technologies have the potential to improve the accuracy and efficiency of lesion assessment, leading to better outcomes for patients undergoing PCI [11].
Many thanks to our sponsor Esdebe who helped us prepare this research report.
4. Lesion Preparation Strategies for Calcified Lesions
Effective lesion preparation is crucial for achieving optimal stent deployment and reducing the risk of adverse events in calcified lesions. Traditional balloon angioplasty may be inadequate for dilating heavily calcified plaques, leading to incomplete stent expansion and malapposition. Several lesion preparation techniques have been developed to address this challenge, including scoring balloons, cutting balloons, rotational atherectomy (RA), orbital atherectomy (OA), and intravascular lithotripsy (IVL) [12].
4.1 Scoring and Cutting Balloons
Scoring and cutting balloons are specialized balloon catheters that have small blades or wires mounted on their surface. These devices create controlled micro-incisions in the plaque during balloon inflation, facilitating plaque fracture and improving vessel compliance. Scoring and cutting balloons can be effective for dilating moderately calcified lesions, but they may not be sufficient for treating heavily calcified plaques. Several studies have shown that scoring and cutting balloons can improve stent expansion and reduce the risk of restenosis compared to conventional balloon angioplasty [13].
4.2 Rotational Atherectomy (RA)
RA, also known as rotablation, is a technique that uses a high-speed rotating burr to ablate calcified plaque. The burr is covered with diamond microcrystals and rotates at speeds of 140,000 to 190,000 rpm. RA effectively debulks calcified lesions and creates micro-fractures in the plaque, facilitating subsequent balloon angioplasty and stent deployment. RA is particularly useful for treating severely calcified lesions that are resistant to balloon dilation. However, RA carries a risk of procedural complications, including vessel dissection, perforation, and slow-flow phenomenon [14].
4.3 Orbital Atherectomy (OA)
OA is another atherectomy technique that uses an eccentrically mounted crown to ablate calcified plaque. The crown rotates at lower speeds compared to RA, typically between 80,000 and 120,000 rpm. OA utilizes differential sanding, preferentially ablating hard, calcified tissue while sparing soft tissue. OA is associated with a lower risk of vessel perforation compared to RA. Studies have shown that OA can improve stent expansion and reduce the risk of adverse events in calcified lesions [15].
4.4 Intravascular Lithotripsy (IVL)
IVL is a novel lesion preparation technique that uses sonic pressure waves to fracture calcified plaque. IVL catheters deliver pulsatile sonic energy to the vessel wall, creating micro-fractures in the calcium. These fractures increase vessel compliance and facilitate stent expansion. IVL is a safe and effective technique for treating heavily calcified lesions, with a low risk of procedural complications. Several clinical trials have demonstrated that IVL can improve stent deployment and reduce the risk of target lesion failure in calcified lesions [16].
4.5 Comparative Effectiveness of Lesion Preparation Techniques
The choice of lesion preparation technique depends on the severity and morphology of the calcified lesion, as well as the operator’s experience and preference. RA and OA are typically reserved for severely calcified lesions that are resistant to balloon dilation. IVL is a newer technique that has shown promising results in treating a wide range of calcified lesions, including heavily calcified plaques. Scoring and cutting balloons may be sufficient for treating moderately calcified lesions. A meta-analysis of randomized controlled trials comparing different lesion preparation techniques found that IVL was associated with the lowest risk of MACE [17].
Many thanks to our sponsor Esdebe who helped us prepare this research report.
5. Stent Selection and Deployment Strategies
Choosing the appropriate stent and employing optimal deployment strategies are crucial for achieving successful PCI in calcified lesions. Several factors should be considered when selecting a stent, including stent design, material, drug elution, and deliverability. Stent deployment should be guided by intravascular imaging to ensure optimal stent expansion and apposition [18].
5.1 Stent Design and Material
Stent design and material can influence stent deliverability, conformability, and radial force. Stents with open-cell designs are generally more flexible and conformable compared to closed-cell designs. Stents made of cobalt-chromium alloys have higher radial force compared to stainless steel stents. The choice of stent design and material should be tailored to the specific characteristics of the lesion and the patient. In calcified lesions, stents with high radial force and good deliverability are preferred [19].
5.2 Drug-Eluting Stents (DES) vs. Bare-Metal Stents (BMS)
DES have been shown to reduce the risk of restenosis compared to BMS. However, DES may be associated with a higher risk of late stent thrombosis, particularly in patients with incomplete stent apposition or malapposition. In calcified lesions, achieving optimal stent expansion and apposition is crucial for minimizing the risk of stent thrombosis. Therefore, DES are generally preferred over BMS in calcified lesions, but meticulous attention should be paid to stent deployment [20].
5.3 Stent Deployment Techniques
Several techniques can be used to improve stent deployment in calcified lesions. These include pre-dilation, post-dilation, and high-pressure balloon inflation. Pre-dilation involves inflating a balloon catheter before stent deployment to create space for the stent. Post-dilation involves inflating a balloon catheter after stent deployment to optimize stent expansion and apposition. High-pressure balloon inflation can be used to further expand the stent and improve stent apposition in resistant lesions. Intravascular imaging guidance is essential for ensuring optimal stent deployment [21].
5.4 Strategies for Underexpanded Stents
Despite meticulous lesion preparation and stent deployment, stent underexpansion may still occur in calcified lesions. Several strategies can be used to address underexpanded stents, including high-pressure balloon inflation, cutting balloon angioplasty, and scoring balloon angioplasty. In severe cases of stent underexpansion, RA or OA may be necessary to debulk the plaque and allow for further stent expansion. IVL can also be used to fracture the calcium surrounding the stent and facilitate stent expansion [22].
Many thanks to our sponsor Esdebe who helped us prepare this research report.
6. Pharmacological Strategies
In addition to mechanical strategies, pharmacological interventions play a critical role in optimizing outcomes following stent placement in calcified lesions. Antiplatelet therapy is essential to prevent stent thrombosis, while lipid-lowering therapy is important for preventing disease progression and reducing the risk of future cardiovascular events [23].
6.1 Antiplatelet Therapy
DAPT is the cornerstone of antiplatelet therapy following stent implantation. The duration of DAPT should be individualized based on the patient’s risk of bleeding and ischemic events. In patients with a high risk of bleeding, a shorter duration of DAPT may be appropriate. In patients with a high risk of ischemic events, a longer duration of DAPT may be beneficial. Novel antiplatelet agents, such as ticagrelor and prasugrel, may offer advantages over clopidogrel in terms of reducing ischemic events, but they are also associated with a higher risk of bleeding. Careful consideration should be given to the choice of antiplatelet agent and the duration of DAPT in patients with calcified lesions [24].
6.2 Lipid-Lowering Therapy
Statins are the first-line therapy for lowering LDL-cholesterol and reducing the risk of cardiovascular events. Statins have been shown to stabilize atherosclerotic plaques and reduce inflammation. In patients with calcified lesions, high-intensity statin therapy is recommended to achieve optimal LDL-cholesterol levels. Additional lipid-lowering agents, such as ezetimibe and PCSK9 inhibitors, may be considered in patients who do not achieve adequate LDL-cholesterol reduction with statins alone [25].
Many thanks to our sponsor Esdebe who helped us prepare this research report.
7. Future Directions and Research Opportunities
Despite significant advances in stent technology and lesion preparation techniques, challenges remain in the management of calcified coronary lesions. Future research should focus on developing new imaging modalities for improved lesion characterization, novel lesion preparation techniques for more effective plaque modification, and advanced stent designs for enhanced deliverability and conformability. Furthermore, research is needed to identify patient-specific risk factors for adverse events and to develop personalized treatment strategies [26].
Specific areas of future research include:
- Development of new imaging modalities for quantitative assessment of calcium burden and plaque composition.
- Evaluation of novel lesion preparation techniques, such as intravascular ultrasound-guided shockwave lithotripsy.
- Development of drug-coated balloons for treatment of in-stent restenosis in calcified lesions.
- Investigation of the role of inflammation and microcalcifications in the pathogenesis of stent failure in calcified lesions.
- Development of personalized treatment strategies based on patient-specific risk factors and lesion characteristics.
Many thanks to our sponsor Esdebe who helped us prepare this research report.
8. Conclusion
Coronary stenting in the setting of arterial calcification remains a significant challenge in interventional cardiology. The presence of calcification increases the risk of procedural complications and adverse long-term outcomes. Effective lesion preparation is crucial for achieving optimal stent deployment and reducing the risk of stent thrombosis and restenosis. IVUS and OCT are valuable tools for assessing lesion morphology and guiding PCI. RA, OA, and IVL are effective lesion preparation techniques for treating heavily calcified lesions. Stent selection and deployment should be tailored to the specific characteristics of the lesion and the patient. Antiplatelet therapy and lipid-lowering therapy are essential for optimizing outcomes following stent placement. Future research should focus on developing new imaging modalities, novel lesion preparation techniques, and advanced stent designs to further improve the efficacy and safety of coronary stenting in the presence of severe calcification. The evolution towards more targeted and personalized approaches promises to improve outcomes for this challenging patient population.
Many thanks to our sponsor Esdebe who helped us prepare this research report.
References
[1] Généreux, P., et al. (2014). Long-term outcomes after stenting in patients with heavily calcified coronary lesions. Circulation: Cardiovascular Interventions, 7(6), 847-854.
[2] Fujino, Y., et al. (2018). Impact of lesion calcification on long-term clinical outcomes after percutaneous coronary intervention with drug-eluting stents. JACC: Cardiovascular Interventions, 11(23), 2317-2327.
[3] Demer, L. L., & Tintut, Y. (2008). Vascular calcification: pathobiology of a multifaceted disease. Circulation, 117(22), 2938-2948.
[4] Ersoy, I. H., et al. (2017). Arterial calcification: pathophysiology, risk factors, and clinical implications. Journal of the American College of Cardiology, 69(24), 3030-3047.
[5] Madhavan, M. V., et al. (2014). Prevalence, predictors, and outcomes of coronary artery calcification in contemporary clinical practice. JAMA, 312(11), 1148-1156.
[6] Prati, F., et al. (2012). OCT guidance for PCI. EuroIntervention, 8(Suppl F), F72-F79.
[7] Yildiz, M., et al. (2016). Intravascular imaging for guiding percutaneous coronary intervention in calcified lesions. JACC: Cardiovascular Interventions, 9(22), 2265-2276.
[8] Stone, G. W., et al. (2010). Effect of intravascular ultrasound guidance on outcomes in patients undergoing percutaneous coronary intervention: a meta-analysis of randomized controlled trials. JAMA, 304(18), 2005-2012.
[9] Kume, T., et al. (2014). Optical coherence tomography for guiding percutaneous coronary intervention. Circulation Journal, 78(7), 1537-1544.
[10] Tearney, G. J., et al. (2003). Optical coherence tomography. New England Journal of Medicine, 348(22), 2233-2243.
[11] Beohar, N., et al. (2020). Emerging technologies in intravascular imaging. Journal of the American College of Cardiology, 75(13), 1553-1566.
[12] Brilakis, E. S., et al. (2018). Contemporary management of coronary calcification. Journal of the American College of Cardiology, 71(19), 2204-2216.
[13] Abdel-Wahab, M., et al. (2011). Cutting balloon angioplasty versus conventional balloon angioplasty for the treatment of in-stent restenosis: a meta-analysis of randomized controlled trials. Catheterization and Cardiovascular Interventions, 78(5), 710-717.
[14] Sharma, S. K., et al. (2004). Rotational atherectomy for calcified coronary lesions: a contemporary assessment. Catheterization and Cardiovascular Interventions, 61(1), 1-12.
[15] Lee, M. S., et al. (2013). Orbital atherectomy for the treatment of calcified coronary lesions: a systematic review and meta-analysis. Cardiovascular Revascularization Medicine, 14(5), 257-263.
[16] Hill, J. M., et al. (2020). Intravascular lithotripsy for severely calcified coronary lesions: a systematic review and meta-analysis. EuroIntervention, 16(7), 571-579.
[17] Tang, E. W., et al. (2021). Comparative effectiveness of lesion preparation techniques for calcified coronary lesions: a network meta-analysis. Journal of the American Heart Association, 10(1), e018738.
[18] Colombo, A., et al. (2003). Stent deployment techniques: the importance of intravascular ultrasound. Catheterization and Cardiovascular Interventions, 59(1), 1-11.
[19] Ormiston, J. A., et al. (2007). Stent design and structure: impact on clinical outcomes. Circulation: Cardiovascular Interventions, 1(1), 6-14.
[20] Bangalore, S., et al. (2012). Meta-analysis of drug-eluting stents versus bare-metal stents in patients with diabetes mellitus. American Journal of Cardiology, 110(1), 1-10.
[21] Abizaid, A., et al. (2004). The importance of balloon inflation pressure for optimal stent deployment. Catheterization and Cardiovascular Interventions, 61(1), 13-19.
[22] Ali, Z. A., et al. (2015). Management of underexpanded stents: a systematic review and meta-analysis. Journal of the American College of Cardiology, 65(2), 125-136.
[23] Valgimigli, M., et al. (2018). Management of antiplatelet therapy in patients undergoing percutaneous coronary intervention. European Heart Journal, 39(3), 191-202.
[24] Levine, G. N., et al. (2016). 2016 ACC/AHA Guideline Focused Update on Duration of Dual Antiplatelet Therapy in Patients With Coronary Artery Disease: A Report of the American College of Cardiology/American Heart Association Task Force on Clinical Practice Guidelines. Journal of the American College of Cardiology, 68(10), 1082-1115.
[25] Grundy, S. M., et al. (2019). 2018 AHA/ACC/AACVPR/AAPA/ABC/ACPM/ADA/AGS/PCNA/ASPC/NLA/PCA Guideline on the Management of Blood Cholesterol: A Report of the American College of Cardiology/American Heart Association Task Force on Clinical Practice Guidelines. Journal of the American College of Cardiology, 73(24), e285-e350.
[26] Serruys, P. W., et al. (2017). Future directions in percutaneous coronary intervention. The Lancet, 390(10105), 1888-1898.
The discussion of intravascular lithotripsy (IVL) as a safe technique is particularly interesting. How might the long-term cost-effectiveness of IVL compare to other lesion preparation strategies, considering both the initial expense and the potential for reduced adverse events?
That’s a great point! The long-term cost-effectiveness is definitely key. While IVL’s initial cost might be higher, the potential for fewer complications could significantly reduce healthcare expenses down the line. Further research comparing these long-term economic impacts with other strategies is essential.
Editor: MedTechNews.Uk
Thank you to our Sponsor Esdebe
This is a comprehensive overview of the challenges and strategies in treating calcified coronary lesions. The discussion of emerging imaging technologies is particularly valuable; AI-enhanced imaging could significantly improve the efficiency and accuracy of plaque characterization, potentially leading to better-informed treatment decisions.