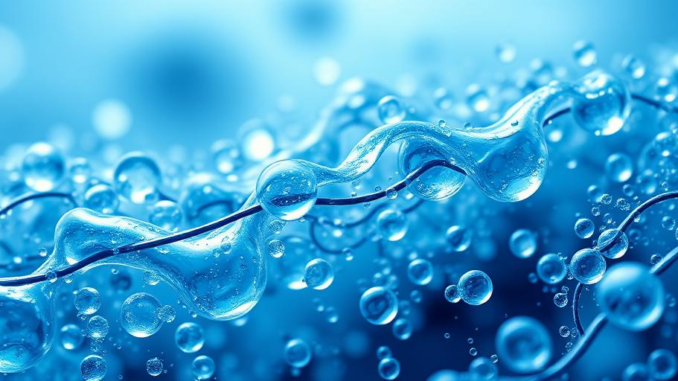
Abstract
Glucolipotoxicity, the detrimental effect of chronic exposure to elevated glucose and fatty acid levels, represents a critical pathogenic mechanism underlying cellular dysfunction in a range of diseases, far exceeding the traditional focus on pancreatic beta-cells in type 2 diabetes (T2D). This research report aims to provide a comprehensive overview of glucolipotoxicity, extending beyond the traditional T2D context to explore its implications in cardiovascular disease, neurodegenerative disorders, and cancer. We delve into the intricate biochemical pathways through which glucolipotoxicity induces cellular damage, including mechanisms like oxidative stress, endoplasmic reticulum (ER) stress, mitochondrial dysfunction, and inflammatory signaling. Furthermore, we examine the specific effects of glucolipotoxicity on diverse cell types, highlighting the common and distinct responses across different tissues. Finally, we discuss current and emerging therapeutic strategies targeting glucolipotoxicity, emphasizing the need for personalized interventions that consider the unique metabolic profile and genetic background of each patient.
Many thanks to our sponsor Esdebe who helped us prepare this research report.
1. Introduction
The term glucolipotoxicity was initially coined to describe the deleterious effects of chronic hyperglycemia and hyperlipidemia on pancreatic beta-cell function and survival, a key driver of T2D progression. However, the understanding of glucolipotoxicity has significantly expanded in recent years. It is now recognized as a broad-spectrum phenomenon impacting a wide array of cell types and contributing to the pathogenesis of multiple diseases. The underlying principle remains consistent: prolonged exposure to excessive glucose and fatty acids overwhelms cellular metabolic capacity, leading to the accumulation of toxic metabolic intermediates and the activation of cellular stress pathways.
While the initial focus was on beta-cells, it is now evident that glucolipotoxicity plays a significant role in other tissues. For example, in cardiomyocytes, it contributes to lipotoxic cardiomyopathy, characterized by impaired contractile function and increased risk of heart failure. In neurons, glucolipotoxicity is implicated in cognitive decline and neurodegenerative diseases like Alzheimer’s disease. Furthermore, accumulating evidence suggests a role for glucolipotoxicity in promoting cancer cell growth, metastasis, and resistance to therapy.
This report aims to provide a holistic view of glucolipotoxicity, moving beyond the traditional diabetes-centric perspective. We will explore the common mechanistic pathways underlying glucolipotoxicity-induced cellular damage, examine the tissue-specific effects of glucolipotoxicity, and discuss the potential of therapeutic interventions targeting these pathways.
Many thanks to our sponsor Esdebe who helped us prepare this research report.
2. Biochemical Mechanisms of Glucolipotoxicity
Glucolipotoxicity triggers a cascade of interconnected biochemical events that ultimately lead to cellular dysfunction and death. The primary mechanisms include:
2.1 Oxidative Stress:
Elevated glucose and fatty acids metabolism results in increased production of reactive oxygen species (ROS). This arises from several sources, including mitochondrial electron transport chain dysfunction, activation of NADPH oxidases, and impaired antioxidant defense mechanisms. Hyperglycemia promotes glucose auto-oxidation and glycation of proteins, further contributing to ROS generation. Lipotoxicity, induced by excess fatty acids, fuels mitochondrial beta-oxidation, leading to ROS overproduction. The resulting oxidative stress damages cellular macromolecules, including DNA, proteins, and lipids, impairing cellular function and triggering apoptosis or necrosis.
While antioxidant therapies have shown promise in preclinical models, clinical trials have yielded mixed results. This could be due to several factors, including the specificity of antioxidants, the timing of intervention, and the complexity of the oxidative stress response. Furthermore, excessive antioxidant supplementation may interfere with important physiological signaling pathways that rely on ROS as signaling molecules. Therefore, a more nuanced approach that targets the specific sources of ROS and enhances endogenous antioxidant defenses may be more effective.
2.2 Endoplasmic Reticulum (ER) Stress:
The ER is responsible for protein folding and processing. In conditions of glucolipotoxicity, the ER becomes overloaded with misfolded or unfolded proteins, leading to ER stress. This triggers the unfolded protein response (UPR), a cellular signaling pathway aimed at restoring ER homeostasis. The UPR initially attempts to resolve the stress by increasing protein folding capacity, reducing protein synthesis, and enhancing ER-associated degradation (ERAD). However, if the ER stress is prolonged or severe, the UPR can activate pro-apoptotic pathways.
Glucolipotoxicity induces ER stress through several mechanisms. Elevated glucose levels can lead to increased protein glycosylation, which can interfere with protein folding. Excess fatty acids can disrupt ER membrane structure and function, further impairing protein folding. Furthermore, oxidative stress can directly damage ER proteins, contributing to ER stress.
Targeting ER stress has emerged as a potential therapeutic strategy for mitigating glucolipotoxicity. Chemical chaperones, such as tauroursodeoxycholic acid (TUDCA), can enhance protein folding and reduce ER stress. Inhibitors of specific UPR signaling pathways, such as IRE1α and PERK, are also being investigated as potential therapeutic agents. However, careful consideration must be given to the potential off-target effects of these inhibitors, as the UPR plays important roles in normal cellular function.
2.3 Mitochondrial Dysfunction:
Mitochondria are the primary sites of energy production in cells. Glucolipotoxicity disrupts mitochondrial function through several mechanisms. Excess glucose and fatty acids overwhelm mitochondrial oxidative capacity, leading to incomplete oxidation and the accumulation of toxic lipid intermediates, such as ceramide and diacylglycerol (DAG). These lipid intermediates can disrupt mitochondrial membrane integrity, impair electron transport chain function, and increase ROS production. Furthermore, glucolipotoxicity can impair mitochondrial biogenesis and dynamics, further compromising mitochondrial function.
Mitochondrial dysfunction is a central feature of glucolipotoxicity-induced cellular damage. Strategies aimed at improving mitochondrial function, such as exercise, calorie restriction, and supplementation with mitochondrial nutrients (e.g., coenzyme Q10, L-carnitine), have shown promise in preclinical studies. Furthermore, inhibitors of mitochondrial fission and promoters of mitochondrial fusion are being investigated as potential therapeutic agents to improve mitochondrial network function.
2.4 Inflammatory Signaling:
Glucolipotoxicity activates inflammatory signaling pathways, contributing to chronic inflammation and cellular damage. Elevated glucose and fatty acid levels can trigger the activation of pattern recognition receptors (PRRs), such as Toll-like receptors (TLRs), on immune cells and parenchymal cells. Activation of TLRs leads to the production of pro-inflammatory cytokines, such as tumor necrosis factor-alpha (TNF-α), interleukin-1 beta (IL-1β), and interleukin-6 (IL-6). These cytokines activate downstream signaling pathways, such as the NF-κB pathway, further amplifying the inflammatory response. Furthermore, glucolipotoxicity can promote the formation of inflammasomes, multiprotein complexes that activate caspase-1, leading to the maturation and release of IL-1β.
Chronic inflammation contributes to glucolipotoxicity-induced cellular damage by promoting oxidative stress, ER stress, and mitochondrial dysfunction. Furthermore, inflammatory cytokines can directly impair cellular function and promote apoptosis. Targeting inflammatory signaling pathways has emerged as a potential therapeutic strategy for mitigating glucolipotoxicity. Inhibitors of TNF-α, IL-1β, and IL-6 are currently used to treat inflammatory diseases, and their potential for treating glucolipotoxicity-related diseases is being investigated. Furthermore, inhibitors of NF-κB and inflammasomes are also being developed as potential therapeutic agents. However, careful consideration must be given to the potential immunosuppressive effects of these inhibitors.
2.5 Altered Autophagy:
Autophagy is a cellular process responsible for the degradation and recycling of damaged organelles and misfolded proteins. While autophagy is generally considered a protective mechanism, glucolipotoxicity can disrupt autophagy, leading to its dysfunction. In some cases, glucolipotoxicity can initially induce autophagy as a compensatory mechanism to remove damaged organelles and proteins. However, prolonged exposure to glucolipotoxic conditions can impair autophagy flux, leading to the accumulation of autophagosomes and a failure to clear damaged cellular components. This impaired autophagy can contribute to ER stress, mitochondrial dysfunction, and cellular apoptosis.
The precise role of autophagy in glucolipotoxicity is complex and may vary depending on the cell type and the severity of the metabolic stress. Strategies aimed at modulating autophagy are being investigated as potential therapeutic agents for mitigating glucolipotoxicity. Enhancing autophagy flux may be beneficial in some contexts, while inhibiting autophagy may be beneficial in others. Therefore, a careful understanding of the role of autophagy in specific tissues and disease states is essential for developing effective autophagy-based therapies.
Many thanks to our sponsor Esdebe who helped us prepare this research report.
3. Tissue-Specific Effects of Glucolipotoxicity
While the fundamental biochemical mechanisms of glucolipotoxicity are shared across different cell types, the specific effects of glucolipotoxicity can vary significantly depending on the tissue. This is due to differences in cellular metabolism, gene expression profiles, and the presence of tissue-specific protective mechanisms.
3.1 Pancreatic Beta-Cells:
As previously mentioned, pancreatic beta-cells are particularly vulnerable to glucolipotoxicity. Chronic exposure to elevated glucose and fatty acid levels impairs insulin secretion and promotes beta-cell apoptosis, leading to T2D. Glucolipotoxicity in beta-cells is characterized by impaired glucose-stimulated insulin secretion (GSIS), increased ROS production, ER stress, mitochondrial dysfunction, and inflammation. Furthermore, glucolipotoxicity can lead to dedifferentiation of beta-cells, resulting in a loss of their specialized function. The consequences of glucolipotocity in pancreatic cells are probably the most studied of all tissues and are widely reported.
3.2 Cardiomyocytes:
In cardiomyocytes, glucolipotoxicity contributes to lipotoxic cardiomyopathy, characterized by impaired contractile function, cardiac hypertrophy, and increased risk of heart failure. Glucolipotoxicity in cardiomyocytes is associated with increased lipid accumulation, ROS production, ER stress, mitochondrial dysfunction, and inflammation. Furthermore, glucolipotoxicity can promote cardiac fibrosis, further impairing cardiac function.
3.3 Neurons:
Glucolipotoxicity is implicated in cognitive decline and neurodegenerative diseases like Alzheimer’s disease. Neurons are highly energy-demanding cells and are particularly vulnerable to metabolic stress. Glucolipotoxicity in neurons is associated with impaired glucose metabolism, increased ROS production, ER stress, mitochondrial dysfunction, and inflammation. Furthermore, glucolipotoxicity can promote the accumulation of amyloid-beta plaques and tau tangles, hallmarks of Alzheimer’s disease.
3.4 Hepatocytes:
In hepatocytes, glucolipotoxicity contributes to non-alcoholic fatty liver disease (NAFLD), which can progress to non-alcoholic steatohepatitis (NASH), cirrhosis, and hepatocellular carcinoma. Glucolipotoxicity in hepatocytes is characterized by increased lipid accumulation, ROS production, ER stress, mitochondrial dysfunction, and inflammation. Furthermore, glucolipotoxicity can promote hepatocyte apoptosis and fibrosis.
3.5 Cancer Cells:
Accumulating evidence suggests a role for glucolipotoxicity in promoting cancer cell growth, metastasis, and resistance to therapy. Cancer cells often exhibit altered metabolic profiles, characterized by increased glucose and fatty acid uptake and utilization. While cancer cells often display a preference for glycolysis (the Warburg effect), they can also utilize fatty acids as an energy source, particularly in nutrient-deprived conditions. Glucolipotoxicity can provide cancer cells with the necessary building blocks and energy to proliferate and metastasize. Furthermore, glucolipotoxicity can activate signaling pathways that promote cancer cell survival and resistance to chemotherapy and radiation therapy.
Many thanks to our sponsor Esdebe who helped us prepare this research report.
4. Therapeutic Strategies Targeting Glucolipotoxicity
Given the widespread implications of glucolipotoxicity in various diseases, targeting this pathogenic mechanism represents a promising therapeutic strategy. Several approaches are being investigated, including:
4.1 Lifestyle Interventions:
Lifestyle interventions, such as diet and exercise, are the cornerstone of preventing and managing glucolipotoxicity. A healthy diet, low in saturated and trans fats and rich in fiber and antioxidants, can help to reduce glucose and lipid overload. Regular exercise can improve insulin sensitivity, enhance glucose and lipid metabolism, and reduce oxidative stress. While these are widely recognised to be advantageous in combating gluliolipotoxicity, long term patient compliance is often lacking.
4.2 Pharmacological Agents:
Several pharmacological agents are being investigated for their ability to mitigate glucolipotoxicity. These include:
- Insulin Sensitizers: Thiazolidinediones (TZDs) and metformin are insulin sensitizers that improve glucose uptake and utilization, reducing hyperglycemia and preventing glucolipotoxicity. TZDs activate PPARγ, a nuclear receptor that regulates glucose and lipid metabolism. Metformin inhibits hepatic glucose production and improves insulin sensitivity. However, TZDs can have side effects, such as weight gain and fluid retention, while metformin can cause gastrointestinal discomfort.
- GLP-1 Receptor Agonists: Glucagon-like peptide-1 (GLP-1) receptor agonists are incretin mimetics that stimulate insulin secretion and suppress glucagon secretion, improving glucose control. Furthermore, GLP-1 receptor agonists have been shown to have cardioprotective and neuroprotective effects, which may be related to their ability to mitigate glucolipotoxicity.
- DPP-4 Inhibitors: Dipeptidyl peptidase-4 (DPP-4) inhibitors prevent the degradation of GLP-1, prolonging its action and improving glucose control. Like GLP-1 receptor agonists, DPP-4 inhibitors have been shown to have some degree of cardioprotective effects.
- SGLT2 Inhibitors: Sodium-glucose cotransporter-2 (SGLT2) inhibitors block the reabsorption of glucose in the kidneys, increasing glucose excretion and lowering blood glucose levels. SGLT2 inhibitors have also been shown to have cardioprotective effects, possibly by reducing glucolipotoxicity and improving myocardial energy metabolism.
- Lipid-Lowering Agents: Statins are HMG-CoA reductase inhibitors that lower cholesterol levels. Fibrates are PPARα agonists that lower triglyceride levels. These lipid-lowering agents can reduce lipotoxicity and improve cellular function.
- Antioxidants: Antioxidants, such as vitamin E, vitamin C, and N-acetylcysteine (NAC), can reduce oxidative stress and protect cells from damage. However, as discussed earlier, the clinical efficacy of antioxidant therapies has been mixed.
- ER Stress Modulators: Chemical chaperones, such as TUDCA, can enhance protein folding and reduce ER stress. Inhibitors of specific UPR signaling pathways, such as IRE1α and PERK, are also being investigated as potential therapeutic agents.
4.3 Novel Molecular Targets:
Emerging research is identifying novel molecular targets for mitigating glucolipotoxicity. These include:
- AMPK Activators: AMP-activated protein kinase (AMPK) is a master regulator of cellular energy metabolism. Activation of AMPK can improve glucose and lipid metabolism, reduce oxidative stress, and enhance mitochondrial function. AMPK activators, such as metformin and berberine, are being investigated as potential therapeutic agents for glucolipotoxicity.
- Sirtuin Activators: Sirtuins are a family of NAD+-dependent deacetylases that regulate cellular aging and stress resistance. Activation of sirtuins can improve glucose and lipid metabolism, reduce oxidative stress, and enhance mitochondrial function. Resveratrol, a natural polyphenol found in red wine, is a sirtuin activator that has shown promise in preclinical studies.
- Mitochondrial Targeted Therapies: Novel therapies aimed at improving mitochondrial function, such as mitochondrial antioxidants and mitochondrial biogenesis enhancers, are being developed to combat glucolipotoxicity.
- Inflammation Inhibitors: Inhibitors of specific inflammatory pathways, such as NF-κB and inflammasomes, are being investigated as potential therapeutic agents for mitigating glucolipotoxicity-induced inflammation.
Many thanks to our sponsor Esdebe who helped us prepare this research report.
5. Personalized Medicine and Glucolipotoxicity
Given the complexity of glucolipotoxicity and the heterogeneity of patients, a personalized medicine approach may be necessary to optimize therapeutic outcomes. This involves tailoring treatment strategies to the individual’s unique metabolic profile, genetic background, and disease stage. Factors to consider include:
- Genetic Predisposition: Genetic variations can influence an individual’s susceptibility to glucolipotoxicity. Identifying individuals with genetic predispositions to metabolic disorders can allow for early intervention and prevention.
- Metabolic Phenotyping: Metabolic profiling can identify individuals with specific metabolic abnormalities, such as impaired glucose tolerance, insulin resistance, or dyslipidemia. This information can be used to tailor dietary and exercise recommendations and to select appropriate pharmacological agents.
- Disease Stage: The optimal therapeutic strategy may vary depending on the stage of the disease. Early intervention may focus on preventing the development of glucolipotoxicity, while later interventions may focus on mitigating the damage caused by chronic metabolic stress.
- Comorbidities: The presence of comorbidities, such as cardiovascular disease, neurodegenerative disorders, or cancer, can influence the choice of therapeutic agents and the overall treatment strategy.
Many thanks to our sponsor Esdebe who helped us prepare this research report.
6. Conclusion
Glucolipotoxicity is a complex and multifaceted phenomenon that contributes to the pathogenesis of a wide range of diseases. Understanding the intricate biochemical mechanisms underlying glucolipotoxicity and the tissue-specific effects of metabolic stress is crucial for developing effective therapeutic strategies. While lifestyle interventions and existing pharmacological agents can help to mitigate glucolipotoxicity, novel molecular targets are being identified that hold promise for more targeted and effective therapies. A personalized medicine approach, taking into account the individual’s unique metabolic profile and genetic background, may be necessary to optimize therapeutic outcomes. Further research is needed to fully elucidate the role of glucolipotoxicity in various diseases and to develop innovative therapeutic strategies that can prevent and treat these devastating conditions.
Many thanks to our sponsor Esdebe who helped us prepare this research report.
References
- Unger, R. H. (2003). Lipotoxicity and beta-cell failure in type 2 diabetes mellitus. Diabetes, 52(Suppl 3), S11-S19.
- Newsholme, P., Keane, D., Carlessi, R., & Bender, K. (2007). Glutamine metabolism and its importance in cell function. Journal of Nutritional Biochemistry, 18(10), 727-736.
- Donath, M. Y., & Böni-Schnetzler, M. (2015). From glucotoxicity to glucolipotoxicity: mechanisms and relevance for islet beta-cell dysfunction. Endocrine Reviews, 36(5), 451-476.
- Schaffer, J. E. (2003). Lipotoxicity: when tissues overeat. Current Opinion in Lipidology, 14(3), 281-287.
- Cnop, M., Pattou, F., & Maedler, K. (2008). Glucolipotoxicity in pancreatic beta cells: a double hit that matters. Diabetes, 57(6), 1455-1466.
- Evans, J. L., Goldfine, I. D., Maddux, B. A., & Grodsky, G. M. (2002). Oxidative stress and stress-activated signaling pathways: a unifying hypothesis of type 2 diabetes. Endocrine Reviews, 23(5), 599-622.
- Hotamisligil, G. S. (2010). Endoplasmic reticulum stress and the inflammatory basis of metabolic disease. Cell, 140(6), 900-917.
- Sharma, V., & Fon Tacer, K. (2017). ER stress, oxidative stress and mitochondrial dysfunction: interconnected paradigms in non-alcoholic steatohepatitis. World Journal of Gastroenterology, 23(24), 4377-4389.
- Nunnari, J., & Suomalainen, A. (2012). Mitochondria: in sickness and in health. Cell, 148(6), 1145-1159.
- Olefsky, J. M., & Glass, C. K. (2010). Macrophages, inflammation, and insulin resistance. Annual Review of Physiology, 72, 219-246.
- Mizushima, N., Yoshimori, T., & Ohsumi, Y. (2008). LC3, a marker protein for autophagy. Autophagy, 4(8), 1017-1023.
- Bugger, H., & Abel, E. D. (2008). Molecular mechanisms of diabetic cardiomyopathy. Diabetologia, 51(10), 1741-1750.
- de la Monte, S. M., & Wands, J. R. (2008). Alzheimer’s disease is type 3 diabetes-evidence reviewed. Journal of Diabetes Science and Technology, 2(6), 1101-1113.
- Schattenberg, J. M., & Loomba, R. (2009). Non-alcoholic fatty liver disease: current understanding and future impact. Gut, 58(4), 585-599.
- Luo, W., & Semenza, G. L. (2011). Emerging roles of metabolic pathways in cancer metastasis. Journal of Clinical Investigation, 121(10), 3807-3813.
- Holman, R. R., et al. (2008). Effect of intensive blood-glucose control with metformin on complications in overweight patients with type 2 diabetes (UKPDS 34). The Lancet, 352(9131), 854-865.
- Zinman, B., et al. (2015). Empagliflozin, cardiovascular outcomes, and mortality in type 2 diabetes. New England Journal of Medicine, 373(22), 2117-2128.
- Viollet, B., Guigas, B., Sanz Garcia, N., Leclerc, J., Foretz, M., & Andreelli, F. (2012). Cellular and molecular mechanisms of metformin action. Clinical Chemistry and Laboratory Medicine, 50(3), 413-419.
- Cantó, C., Auwerx, J. (2009). PGC-1α, SIRT1 and AMPK, an energy sensing network that controls energy expenditure. Current Opinion in Lipidology, 20(2), 98-105.
- Madeo, F., Tavernarakis, N., & Kroemer, G. (2010). Can autophagy promote longevity?. Nature Cell Biology, 12(9), 842-846.
The discussion of altered autophagy is particularly compelling. Given its complex and context-dependent role in glucolipotoxicity, what specific autophagy-modulating therapies show the most promise for targeted interventions in different tissues or disease stages?
That’s a great question! The context-dependent nature of autophagy is key. While some studies suggest mTOR inhibitors to enhance autophagy, others explore autophagy induction via Beclin 1 activators. Determining the optimal strategy requires careful consideration of the specific tissue and disease stage, as you point out. Hopefully, further research will bring clarity!
Editor: MedTechNews.Uk
Thank you to our Sponsor Esdebe
Given the tissue-specific effects described, what are the key challenges in developing therapies that selectively target glucolipotoxicity in one tissue without affecting others, especially considering the potential for systemic effects?
That’s a really important point. The tissue-specific nature of glucolipotoxicity presents a significant challenge. As you mentioned, systemic therapies could have unintended consequences. One promising avenue is developing targeted drug delivery systems that ensure the therapeutic agent reaches only the affected tissue, minimizing off-target effects. It is a very complex issue. #glucolipotoxicity #metabolicdisease
Editor: MedTechNews.Uk
Thank you to our Sponsor Esdebe
So, if lifestyle interventions are the cornerstone, does that mean we’re building our metabolic health castles on somewhat crumbly foundations given adherence rates? What innovative strategies might actually *stick* long-term?
That’s a really insightful point about adherence. It’s true that lifestyle changes can be difficult to maintain. Perhaps gamification or community-based support programs could make a real difference in improving long-term adherence. Focusing on small, achievable goals might also be key for sustained success.
Editor: MedTechNews.Uk
Thank you to our Sponsor Esdebe