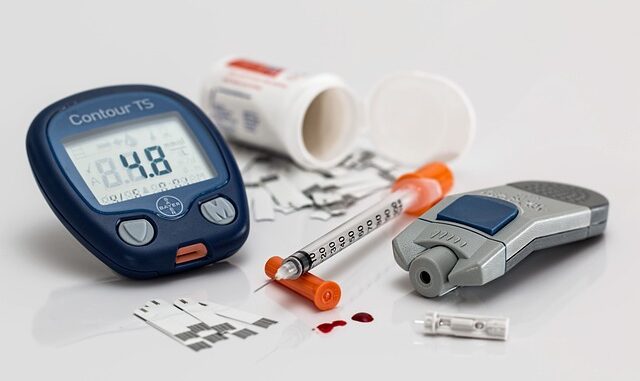
Abstract
Glucose, a monosaccharide, serves as the primary energy source for most living organisms. Its metabolism is intricately regulated to maintain cellular homeostasis and fuel essential biological processes. This review delves into the multifaceted aspects of glucose metabolism, exploring the regulatory mechanisms governing glucose uptake, utilization, and storage. We examine key metabolic pathways, including glycolysis, gluconeogenesis, glycogenesis, and glycogenolysis, highlighting the enzymes and signaling molecules involved. Furthermore, we discuss the roles of hormones such as insulin, glucagon, and epinephrine in modulating glucose homeostasis. The impact of glucose metabolism on various physiological processes, including brain function, muscle activity, and immune response, is explored. Finally, we analyze the implications of dysregulated glucose metabolism in the pathogenesis of metabolic disorders such as diabetes mellitus, metabolic syndrome, and cancer, and discuss potential therapeutic strategies targeting glucose metabolism to improve health outcomes.
Many thanks to our sponsor Esdebe who helped us prepare this research report.
1. Introduction
Glucose, a six-carbon sugar (C6H12O6), occupies a central role in cellular metabolism and energy production across diverse life forms. From unicellular organisms to complex multicellular beings, glucose serves as the primary fuel source, powering essential biological processes. Its significance stems from its ready availability, efficient energy yield upon oxidation, and versatile participation in various metabolic pathways. This review provides a comprehensive overview of glucose metabolism, encompassing regulatory mechanisms, key metabolic pathways, hormonal control, physiological roles, and pathological implications.
Maintaining stable glucose levels within a narrow physiological range is crucial for optimal cellular function and overall health. Fluctuations in blood glucose concentrations, both hyperglycemia (elevated glucose) and hypoglycemia (low glucose), can disrupt cellular processes and lead to various health complications. The human body employs a sophisticated network of regulatory mechanisms to maintain glucose homeostasis, involving hormonal signals, enzymatic control, and cellular transport processes. Understanding these mechanisms is essential for comprehending the pathogenesis of metabolic disorders associated with glucose dysregulation.
Glucose metabolism involves a complex interplay of anabolic (building) and catabolic (breaking down) pathways. Glycolysis, the breakdown of glucose into pyruvate, is a fundamental metabolic process occurring in the cytoplasm of cells. Pyruvate can then be further metabolized through oxidative phosphorylation in mitochondria to generate ATP, the cell’s energy currency. Conversely, gluconeogenesis, the synthesis of glucose from non-carbohydrate precursors, is a vital pathway for maintaining glucose levels during fasting or prolonged exercise. Glycogenesis, the storage of glucose as glycogen in the liver and muscles, and glycogenolysis, the breakdown of glycogen to release glucose, also play important roles in glucose homeostasis.
The regulation of glucose metabolism is tightly controlled by hormones, primarily insulin and glucagon. Insulin, secreted by pancreatic beta cells in response to elevated glucose levels, promotes glucose uptake by cells, stimulates glycogen synthesis, and inhibits gluconeogenesis. Glucagon, secreted by pancreatic alpha cells in response to low glucose levels, stimulates glycogenolysis and gluconeogenesis, increasing glucose release into the bloodstream. Other hormones, such as epinephrine and cortisol, also influence glucose metabolism under specific physiological conditions.
Dysregulation of glucose metabolism underlies various metabolic disorders, including diabetes mellitus, metabolic syndrome, and cancer. Diabetes mellitus, characterized by hyperglycemia due to insulin deficiency or resistance, is a major global health concern. Metabolic syndrome, a cluster of metabolic abnormalities including insulin resistance, obesity, dyslipidemia, and hypertension, increases the risk of developing type 2 diabetes and cardiovascular disease. Cancer cells often exhibit altered glucose metabolism, favoring glycolysis even in the presence of oxygen (Warburg effect), which supports rapid cell proliferation.
This review aims to provide a comprehensive and up-to-date overview of glucose metabolism, covering its intricate regulatory mechanisms, key metabolic pathways, hormonal control, physiological roles, and pathological implications. By elucidating these aspects, we aim to enhance understanding of glucose metabolism and its relevance to human health and disease.
Many thanks to our sponsor Esdebe who helped us prepare this research report.
2. Regulatory Mechanisms of Glucose Metabolism
Maintaining glucose homeostasis requires a complex and finely tuned regulatory system. This system involves hormonal signals, enzymatic control, and cellular transport processes that coordinate glucose uptake, utilization, and storage. Understanding these regulatory mechanisms is crucial for comprehending the pathogenesis of metabolic disorders associated with glucose dysregulation.
2.1 Hormonal Regulation
Insulin and glucagon are the primary hormones responsible for regulating blood glucose levels. Their opposing actions maintain glucose homeostasis in response to changes in glucose availability.
- Insulin: Secreted by pancreatic beta cells in response to elevated blood glucose, insulin promotes glucose uptake by cells, primarily in muscle and adipose tissue. It stimulates the translocation of GLUT4, a glucose transporter protein, to the cell surface, facilitating glucose entry into cells. Insulin also stimulates glycogen synthesis in the liver and muscles, converting excess glucose into glycogen for storage. Furthermore, insulin inhibits gluconeogenesis in the liver, reducing glucose production. The signaling pathway of insulin involves the activation of insulin receptor tyrosine kinase, leading to the phosphorylation and activation of downstream signaling molecules, including PI3K and Akt, which mediate the metabolic effects of insulin.
- Glucagon: Secreted by pancreatic alpha cells in response to low blood glucose, glucagon stimulates glycogenolysis and gluconeogenesis in the liver, increasing glucose release into the bloodstream. It binds to glucagon receptors on liver cells, activating adenylate cyclase and increasing intracellular cAMP levels. cAMP activates protein kinase A (PKA), which phosphorylates and activates enzymes involved in glycogen breakdown and glucose synthesis. Glucagon also inhibits glycolysis and glycogen synthesis in the liver.
Epinephrine, also known as adrenaline, is released during stress or exercise and increases glucose availability by stimulating glycogenolysis in the liver and muscles. Cortisol, a glucocorticoid hormone secreted by the adrenal cortex, also promotes gluconeogenesis in the liver and antagonizes insulin action.
2.2 Enzymatic Control
The activity of key enzymes involved in glucose metabolism is regulated through various mechanisms, including allosteric regulation, covalent modification, and changes in enzyme expression levels.
- Allosteric Regulation: Several enzymes are allosterically regulated by metabolites that bind to sites other than the active site, altering the enzyme’s conformation and activity. For example, phosphofructokinase-1 (PFK-1), a key enzyme in glycolysis, is allosterically activated by AMP and ADP and inhibited by ATP and citrate, reflecting the energy status of the cell.
- Covalent Modification: Many enzymes are regulated by phosphorylation and dephosphorylation, catalyzed by protein kinases and phosphatases, respectively. For example, glycogen synthase, the enzyme responsible for glycogen synthesis, is inhibited by phosphorylation and activated by dephosphorylation.
- Enzyme Expression: The expression levels of enzymes involved in glucose metabolism can be regulated by hormones and transcription factors. For example, insulin increases the expression of glucokinase, the enzyme that phosphorylates glucose in the liver, while glucagon increases the expression of gluconeogenic enzymes.
2.3 Cellular Transport
Glucose transport across cell membranes is mediated by glucose transporter proteins (GLUTs). Different GLUT isoforms exhibit distinct tissue distribution and kinetic properties, reflecting their specialized roles in glucose metabolism.
- GLUT1: Widely expressed in various tissues, including brain, erythrocytes, and placenta, GLUT1 exhibits high affinity for glucose and is responsible for basal glucose uptake.
- GLUT2: Expressed in the liver, pancreatic beta cells, and small intestine, GLUT2 has low affinity for glucose and plays a role in glucose sensing and transport of large amounts of glucose.
- GLUT3: Primarily expressed in neurons, GLUT3 exhibits high affinity for glucose and ensures a constant supply of glucose to the brain.
- GLUT4: Expressed in muscle and adipose tissue, GLUT4 is insulin-regulated and mediates insulin-stimulated glucose uptake. Insulin promotes the translocation of GLUT4 from intracellular vesicles to the cell surface, increasing glucose transport.
- GLUT5: Expressed in the small intestine, GLUT5 is a fructose transporter and plays a role in fructose absorption.
Many thanks to our sponsor Esdebe who helped us prepare this research report.
3. Key Metabolic Pathways of Glucose
Glucose metabolism involves a complex interplay of anabolic and catabolic pathways. These pathways are tightly regulated to maintain glucose homeostasis and provide energy for cellular functions.
3.1 Glycolysis
Glycolysis is the breakdown of glucose into pyruvate, a fundamental metabolic process occurring in the cytoplasm of cells. It involves a series of ten enzymatic reactions, each catalyzed by a specific enzyme. Glycolysis can occur in both aerobic and anaerobic conditions.
- Aerobic Glycolysis: In the presence of oxygen, pyruvate is converted to acetyl-CoA, which enters the citric acid cycle (Krebs cycle) in mitochondria, leading to the complete oxidation of glucose to carbon dioxide and water, generating a large amount of ATP through oxidative phosphorylation.
- Anaerobic Glycolysis: In the absence of oxygen, pyruvate is converted to lactate, regenerating NAD+ for glycolysis to continue. Anaerobic glycolysis produces a smaller amount of ATP compared to aerobic glycolysis but allows for rapid energy production under oxygen-limiting conditions.
Key regulatory enzymes in glycolysis include hexokinase, phosphofructokinase-1 (PFK-1), and pyruvate kinase. PFK-1 is the rate-limiting enzyme in glycolysis and is allosterically regulated by ATP, AMP, and citrate.
3.2 Gluconeogenesis
Gluconeogenesis is the synthesis of glucose from non-carbohydrate precursors, such as pyruvate, lactate, glycerol, and amino acids. It occurs primarily in the liver and, to a lesser extent, in the kidneys. Gluconeogenesis is essential for maintaining blood glucose levels during fasting, starvation, or prolonged exercise.
Gluconeogenesis involves several enzymes that bypass the irreversible steps of glycolysis, including pyruvate carboxylase, phosphoenolpyruvate carboxykinase (PEPCK), fructose-1,6-bisphosphatase, and glucose-6-phosphatase. The regulation of gluconeogenesis is complex and involves hormonal signals, such as glucagon and cortisol, as well as allosteric regulation of key enzymes.
3.3 Glycogenesis
Glycogenesis is the synthesis of glycogen from glucose. Glycogen is a branched polymer of glucose that serves as the primary storage form of glucose in the liver and muscles. Glycogenesis occurs when glucose levels are high, such as after a meal.
The key enzyme in glycogenesis is glycogen synthase, which catalyzes the addition of glucose units to the growing glycogen chain. Glycogen synthase is regulated by phosphorylation and dephosphorylation, with phosphorylation inhibiting its activity.
3.4 Glycogenolysis
Glycogenolysis is the breakdown of glycogen to release glucose. It occurs when glucose levels are low, such as during fasting or exercise. Glycogenolysis is regulated by hormones, such as glucagon and epinephrine, which stimulate glycogen breakdown.
The key enzyme in glycogenolysis is glycogen phosphorylase, which catalyzes the cleavage of glucose units from glycogen. Glycogen phosphorylase is activated by phosphorylation, which is stimulated by glucagon and epinephrine.
3.5 Pentose Phosphate Pathway
The pentose phosphate pathway (PPP) is a metabolic pathway that generates NADPH and pentose sugars, such as ribose-5-phosphate. NADPH is essential for reducing power in anabolic reactions and protecting cells from oxidative stress. Ribose-5-phosphate is a precursor for nucleotide biosynthesis.
The PPP occurs in the cytoplasm of cells and involves two phases: an oxidative phase and a non-oxidative phase. The oxidative phase generates NADPH, while the non-oxidative phase converts pentose sugars into intermediates that can enter glycolysis or gluconeogenesis.
Many thanks to our sponsor Esdebe who helped us prepare this research report.
4. Physiological Roles of Glucose Metabolism
Glucose metabolism plays crucial roles in various physiological processes, providing energy for cellular functions and supporting essential biological activities.
4.1 Brain Function
The brain relies heavily on glucose as its primary energy source. Neurons have a high metabolic rate and require a constant supply of glucose to maintain membrane potential, transmit nerve impulses, and synthesize neurotransmitters. Glucose is transported across the blood-brain barrier by GLUT1 and GLUT3 transporters. Hypoglycemia can impair brain function, leading to confusion, seizures, and coma.
4.2 Muscle Activity
Muscles utilize glucose as a fuel source for both aerobic and anaerobic exercise. During aerobic exercise, glucose is oxidized to carbon dioxide and water, generating ATP to power muscle contraction. During anaerobic exercise, glucose is converted to lactate, providing a rapid source of energy but leading to muscle fatigue.
Muscle glucose uptake is regulated by insulin and muscle contraction. Insulin stimulates GLUT4 translocation to the cell surface, increasing glucose uptake. Muscle contraction also stimulates GLUT4 translocation through a signaling pathway independent of insulin.
4.3 Immune Response
Immune cells, such as lymphocytes and macrophages, rely on glucose metabolism to support their activation, proliferation, and effector functions. Glucose uptake and glycolysis are upregulated in activated immune cells, providing energy and biosynthetic precursors for immune responses.
Altered glucose metabolism can impair immune function, making individuals more susceptible to infections and inflammatory diseases. For example, hyperglycemia in diabetes can impair neutrophil function and increase the risk of infections.
4.4 Liver Function
The liver plays a central role in glucose homeostasis, regulating glucose uptake, storage, and release. It takes up glucose from the portal vein after a meal, converting it to glycogen for storage. During fasting, the liver releases glucose into the bloodstream through glycogenolysis and gluconeogenesis, maintaining blood glucose levels.
Liver dysfunction can impair glucose homeostasis, leading to hyperglycemia or hypoglycemia. For example, liver cirrhosis can impair gluconeogenesis, increasing the risk of hypoglycemia.
Many thanks to our sponsor Esdebe who helped us prepare this research report.
5. Pathological Implications of Dysregulated Glucose Metabolism
Dysregulation of glucose metabolism underlies various metabolic disorders, including diabetes mellitus, metabolic syndrome, and cancer. Understanding the pathological implications of dysregulated glucose metabolism is crucial for developing effective therapeutic strategies.
5.1 Diabetes Mellitus
Diabetes mellitus is a group of metabolic disorders characterized by hyperglycemia due to insulin deficiency or resistance. Type 1 diabetes is an autoimmune disease in which the immune system destroys pancreatic beta cells, leading to insulin deficiency. Type 2 diabetes is characterized by insulin resistance, in which cells fail to respond properly to insulin, and impaired insulin secretion.
Chronic hyperglycemia in diabetes can lead to various complications, including cardiovascular disease, neuropathy, nephropathy, and retinopathy. These complications are caused by the damaging effects of high glucose levels on blood vessels and other tissues.
5.2 Metabolic Syndrome
Metabolic syndrome is a cluster of metabolic abnormalities including insulin resistance, obesity, dyslipidemia, and hypertension. It increases the risk of developing type 2 diabetes, cardiovascular disease, and non-alcoholic fatty liver disease (NAFLD).
Insulin resistance is a central feature of metabolic syndrome, contributing to hyperglycemia, dyslipidemia, and hypertension. Obesity, particularly visceral obesity, is strongly associated with insulin resistance and metabolic syndrome. NAFLD is a common complication of metabolic syndrome, characterized by excessive fat accumulation in the liver.
5.3 Cancer
Cancer cells often exhibit altered glucose metabolism, favoring glycolysis even in the presence of oxygen (Warburg effect). This metabolic shift provides cancer cells with a growth advantage, supporting rapid cell proliferation and survival.
Cancer cells increase glucose uptake and glycolysis to generate ATP and biosynthetic precursors for cell growth and division. They also increase the expression of enzymes involved in glycolysis and suppress mitochondrial oxidative phosphorylation. Targeting glucose metabolism is an emerging strategy for cancer therapy.
Many thanks to our sponsor Esdebe who helped us prepare this research report.
6. Therapeutic Strategies Targeting Glucose Metabolism
Therapeutic strategies targeting glucose metabolism are aimed at restoring glucose homeostasis and preventing or treating metabolic disorders. These strategies include lifestyle modifications, pharmacological interventions, and emerging therapies.
6.1 Lifestyle Modifications
Lifestyle modifications, including diet and exercise, are essential for managing glucose levels and preventing or delaying the onset of type 2 diabetes and metabolic syndrome. A healthy diet, rich in fruits, vegetables, and whole grains, and low in saturated and trans fats, can improve insulin sensitivity and reduce blood glucose levels. Regular physical activity increases glucose uptake by muscles and improves insulin sensitivity. Weight loss, even modest, can significantly improve metabolic health.
6.2 Pharmacological Interventions
Various pharmacological agents are available to treat diabetes and improve glucose control. These include:
- Insulin: Insulin therapy is essential for type 1 diabetes and is often used in type 2 diabetes to control blood glucose levels. Different types of insulin are available, including rapid-acting, short-acting, intermediate-acting, and long-acting insulins.
- Metformin: Metformin is a first-line drug for type 2 diabetes. It reduces glucose production in the liver and improves insulin sensitivity in peripheral tissues.
- Sulfonylureas: Sulfonylureas stimulate insulin secretion from pancreatic beta cells. They are effective in lowering blood glucose levels but can cause hypoglycemia.
- Thiazolidinediones (TZDs): TZDs improve insulin sensitivity in peripheral tissues by activating PPARgamma receptors. They can cause weight gain and fluid retention.
- DPP-4 Inhibitors: DPP-4 inhibitors prevent the breakdown of incretin hormones, which stimulate insulin secretion and suppress glucagon secretion. They have a low risk of hypoglycemia.
- GLP-1 Receptor Agonists: GLP-1 receptor agonists mimic the effects of incretin hormones, stimulating insulin secretion, suppressing glucagon secretion, and slowing gastric emptying. They can promote weight loss.
- SGLT2 Inhibitors: SGLT2 inhibitors block glucose reabsorption in the kidneys, increasing glucose excretion in the urine. They can promote weight loss and lower blood pressure.
6.3 Emerging Therapies
Emerging therapies targeting glucose metabolism include:
- Glucose-Lowering Devices: Continuous glucose monitoring (CGM) systems provide real-time glucose readings and can alert individuals to high or low glucose levels. Insulin pumps deliver insulin continuously, providing precise control of blood glucose levels. Artificial pancreas systems combine CGM and insulin pump technology to automatically regulate blood glucose levels.
- Gene Therapy: Gene therapy aims to correct genetic defects that cause diabetes or metabolic disorders. For example, gene therapy can be used to deliver the insulin gene to pancreatic beta cells, restoring insulin production.
- Stem Cell Therapy: Stem cell therapy involves replacing damaged or destroyed pancreatic beta cells with new beta cells derived from stem cells. This approach holds promise for treating type 1 diabetes.
- Metabolic Surgery: Bariatric surgery, also known as metabolic surgery, can improve glucose control and induce remission of type 2 diabetes in obese individuals. The mechanisms by which bariatric surgery improves glucose metabolism are complex and involve hormonal changes, alterations in gut microbiota, and improved insulin sensitivity.
Many thanks to our sponsor Esdebe who helped us prepare this research report.
7. Conclusion
Glucose metabolism is a complex and tightly regulated process that plays a central role in cellular energy production and overall health. Understanding the regulatory mechanisms, key metabolic pathways, hormonal control, physiological roles, and pathological implications of glucose metabolism is crucial for comprehending the pathogenesis of metabolic disorders and developing effective therapeutic strategies. Dysregulation of glucose metabolism underlies various diseases, including diabetes mellitus, metabolic syndrome, and cancer. Therapeutic strategies targeting glucose metabolism, including lifestyle modifications, pharmacological interventions, and emerging therapies, are aimed at restoring glucose homeostasis and improving health outcomes. Continued research in this area is essential for advancing our understanding of glucose metabolism and developing novel therapies for metabolic disorders.
Many thanks to our sponsor Esdebe who helped us prepare this research report.
References
- Berg, J. M., Tymoczko, J. L., & Stryer, L. (2002). Biochemistry. 5th edition. New York: W H Freeman.
- DeFronzo, R. A. (2009). From the triumvirate to the ominous octet: a new paradigm for the treatment of type 2 diabetes mellitus. Diabetes, 58(4), 773-795.
- Han, H. S., Kang, Y. M., Kim, D., & Choi, B. H. (2016). Regulation of glucose metabolism in liver from diabetes. World journal of gastroenterology, 22(27), 6249.
- Haynes, A. L., Boucher, J., & Kahn, C. R. (2021). Insulin resistance. Nature Reviews Disease Primers, 7(1), 1-21.
- Hers, H. G. (1976). The control of glycogen metabolism in the liver. Annual review of biochemistry, 45(1), 167-189.
- Libby, P., Buring, J. E., Badimon, L., Hansson, G. K., Deanfield, J., Maseri, A., … & Ridker, P. M. (2019). Atherosclerosis. Nature Reviews Disease Primers, 5(1), 1-17.
- Luo, J., Oonishi, E., Ohta, A., Ramirez, C. M., Rothenberg, M. E., & Karasuyama, H. (2016). Glucose metabolism in T lymphocytes. Journal of Clinical Investigation, 126(4), 1078-1087.
- Saltiel, A. R., & Olefsky, J. M. (2017). Inflammatory mechanisms contributing to insulin resistance. Physiological reviews, 97(1), 181-214.
- Vander Heiden, M. G., Cantley, L. C., & Thompson, C. B. (2009). Understanding the Warburg effect: metabolic requirements of cell proliferation. Science, 324(5930), 1029-1033.
- Warburg, O. (1956). On the origin of cancer cells. Science, 123(3191), 309-314.
The discussion on GLUT transporters highlights their crucial role; tissue-specific expression of different isoforms allows for fine-tuned glucose regulation based on varying metabolic demands in the body.
Thanks for highlighting the importance of GLUT transporters! It’s amazing how the tissue-specific expression allows for such precise glucose regulation. Considering the different metabolic needs of, say, muscle versus brain tissue, this fine-tuning is absolutely essential for overall health and function. What other aspects of glucose regulation do you find particularly interesting?
Editor: MedTechNews.Uk
Thank you to our Sponsor Esdebe