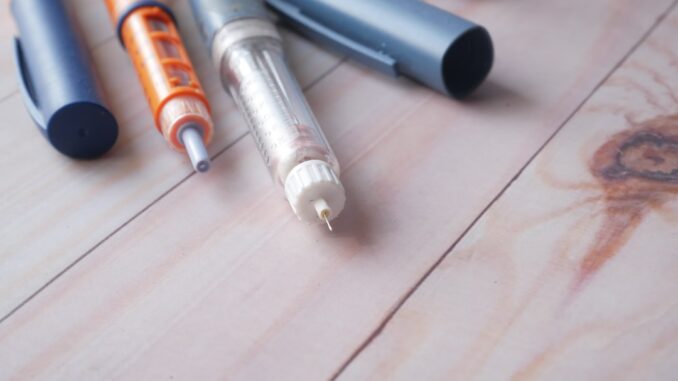
Abstract
Diabetes mellitus, encompassing both type 1 diabetes (T1D) and type 2 diabetes (T2D), represents a significant global health challenge. While T1D is characterized by autoimmune destruction of insulin-producing beta cells in the pancreas, T2D involves insulin resistance and progressive beta-cell dysfunction. Conventional treatments primarily focus on glycemic control; however, they do not address the underlying immunological mechanisms driving disease progression. Immunotherapies, designed to modulate the immune system, hold significant promise for preventing, delaying, or even reversing diabetes. This review provides a comprehensive overview of current research and development in immunotherapeutic strategies for both T1D and T2D. We delve into the mechanisms of action, clinical trial results, potential benefits, and associated risks of various immunotherapeutic approaches, including those targeting T1D (e.g., teplizumab, anti-CD3 antibodies, and dendritic cell therapies) and those under investigation for T2D (e.g., anti-inflammatory cytokines, checkpoint inhibitors, and metabolic vaccines). Furthermore, we critically evaluate the challenges and future directions in this rapidly evolving field, emphasizing the need for personalized immunotherapeutic approaches based on individual patient profiles and disease stages.
Many thanks to our sponsor Esdebe who helped us prepare this research report.
1. Introduction
Diabetes mellitus is a metabolic disorder characterized by hyperglycemia resulting from defects in insulin secretion, insulin action, or both [1]. The two primary forms are type 1 diabetes (T1D) and type 2 diabetes (T2D). T1D is an autoimmune disease where the body’s immune system mistakenly attacks and destroys the insulin-producing beta cells in the pancreas [2]. This leads to absolute insulin deficiency, requiring lifelong insulin therapy. T2D, on the other hand, is characterized by insulin resistance, where cells fail to respond properly to insulin, and a progressive decline in beta-cell function [3]. This results in a relative insulin deficiency. While genetic predisposition plays a role in both T1D and T2D, environmental factors also contribute to disease development.
The current management of diabetes primarily focuses on glycemic control through lifestyle modifications, oral antidiabetic agents, and insulin therapy [4]. However, these treatments do not address the underlying causes of the disease, particularly the autoimmune destruction of beta cells in T1D and the chronic inflammation and beta-cell dysfunction in T2D. Immunotherapies, which aim to modulate the immune system to prevent or reverse disease progression, offer a potentially transformative approach to diabetes management [5].
This review aims to provide a comprehensive overview of the current state of research and development in immunotherapeutic strategies for both T1D and T2D. We will discuss the mechanisms of action, clinical trial results, potential benefits, and risks of various immunotherapeutic approaches. We will also explore the challenges and future directions in this rapidly evolving field, highlighting the need for personalized immunotherapies tailored to individual patient characteristics and disease stages.
Many thanks to our sponsor Esdebe who helped us prepare this research report.
2. Immunotherapeutic Strategies for Type 1 Diabetes
Type 1 diabetes (T1D) is an autoimmune disease mediated by T cells that selectively target and destroy insulin-producing pancreatic beta cells [6]. The pathogenesis of T1D involves a complex interplay of genetic susceptibility, environmental triggers, and immune dysregulation. Immunotherapies for T1D aim to prevent or delay the onset of clinical disease by modulating the immune system to protect beta cells from autoimmune destruction [7].
2.1. Teplizumab
Teplizumab is a humanized anti-CD3 monoclonal antibody that binds to the CD3 receptor on T cells [8]. This binding modulates T cell function without causing significant T cell depletion. The mechanism of action involves altering T cell signaling and promoting immune tolerance, leading to reduced beta-cell destruction.
The pivotal TrialNet study demonstrated that teplizumab significantly delayed the onset of clinical T1D in at-risk individuals with stage 2 T1D (presence of two or more autoantibodies and dysglycemia) [9]. The median time to diagnosis of T1D was significantly longer in the teplizumab group compared to the placebo group (approximately 4 years vs. 2 years). Based on these results, teplizumab was approved by the FDA in 2022, becoming the first disease-modifying therapy for T1D [10].
While teplizumab represents a significant advancement, it is important to note that it does not prevent T1D entirely but rather delays its onset. Furthermore, teplizumab is most effective in individuals at high risk of developing T1D, highlighting the need for early screening and intervention. Potential side effects of teplizumab include cytokine release syndrome, lymphopenia, and increased risk of infections [11]. Further research is needed to optimize teplizumab dosing and identify biomarkers to predict treatment response.
2.2. Anti-CD3 Antibodies (Other than Teplizumab)
Several other anti-CD3 antibodies have been investigated for T1D, including otelixizumab and foralumab. Otelixizumab, a humanized anti-CD3 antibody, showed promising results in early clinical trials, but subsequent larger trials failed to demonstrate a significant benefit in delaying T1D onset [12]. Foralumab, a fully human anti-CD3 antibody, is being investigated in clinical trials and has shown potential to induce immune tolerance and preserve beta-cell function [13]. Unlike teplizumab, foralumab is designed to be non-depleting of T cells and administered nasally, which could offer improved tolerability.
The varying clinical outcomes observed with different anti-CD3 antibodies may be attributed to differences in antibody structure, binding affinity, mechanism of action, and patient populations. Future research should focus on optimizing anti-CD3 antibody design and identifying patient subgroups who are most likely to benefit from this therapy.
2.3. Dendritic Cell Therapies
Dendritic cells (DCs) are antigen-presenting cells that play a critical role in initiating and regulating immune responses [14]. Dendritic cell therapies involve isolating DCs from a patient, modifying them ex vivo to promote immune tolerance, and then re-infusing them back into the patient. In T1D, DCs can be manipulated to present beta-cell antigens in a tolerogenic manner, leading to the induction of regulatory T cells (Tregs) and suppression of autoreactive T cells [15].
Several clinical trials have investigated dendritic cell therapies for T1D, with some studies showing promising results in preserving beta-cell function and reducing insulin requirements [16]. However, these trials have been relatively small, and further research is needed to optimize DC preparation, antigen loading, and administration protocols. Furthermore, the complexity and cost of dendritic cell therapies represent significant challenges to their widespread adoption.
2.4. Regulatory T Cell (Treg) Therapies
Regulatory T cells (Tregs) are a subset of T cells that play a critical role in maintaining immune tolerance and preventing autoimmunity [17]. In T1D, defects in Treg function contribute to the breakdown of immune tolerance and the autoimmune destruction of beta cells. Treg therapies involve isolating Tregs from a patient, expanding them ex vivo, and then re-infusing them back into the patient. The expanded Tregs can then suppress autoreactive T cells and protect beta cells from destruction [18].
Clinical trials of Treg therapies for T1D are ongoing and have shown some initial promising results in preserving beta-cell function and reducing insulin requirements [19]. However, these trials are still in early stages, and further research is needed to optimize Treg expansion, phenotype stability, and homing to the pancreas. Furthermore, the scalability and cost of Treg therapies represent significant challenges to their clinical translation.
2.5. B Cell Depletion Therapy
B cells play a complex role in the pathogenesis of T1D, including antigen presentation, autoantibody production, and T cell activation [20]. Rituximab, an anti-CD20 antibody that depletes B cells, has been investigated as a potential immunotherapeutic agent for T1D. While rituximab effectively depletes B cells, clinical trials have yielded mixed results [21]. Some studies have shown a modest benefit in preserving beta-cell function, while others have not demonstrated a significant effect. This suggests that B cell depletion alone may not be sufficient to prevent or reverse T1D.
2.6. Other Immunomodulatory Approaches
In addition to the therapies discussed above, other immunomodulatory approaches are being investigated for T1D, including: IL-2 administration (to expand Tregs), CTLA-4 agonists (to promote immune tolerance), and islet encapsulation (to protect beta cells from immune attack) [22, 23, 24]. These approaches are still in early stages of development, but they hold promise for improving T1D management.
Many thanks to our sponsor Esdebe who helped us prepare this research report.
3. Immunotherapeutic Strategies for Type 2 Diabetes
Type 2 diabetes (T2D) is a complex metabolic disorder characterized by insulin resistance, progressive beta-cell dysfunction, and chronic inflammation [25]. While genetic factors contribute to T2D susceptibility, lifestyle factors such as obesity, physical inactivity, and poor diet play a significant role in disease development. Unlike T1D, the role of the immune system in T2D was historically underappreciated. However, increasing evidence suggests that chronic low-grade inflammation contributes to insulin resistance and beta-cell dysfunction in T2D [26]. This chronic inflammation is characterized by increased levels of pro-inflammatory cytokines, such as TNF-α, IL-1β, and IL-6, which can impair insulin signaling and promote beta-cell apoptosis [27].
Immunotherapeutic strategies for T2D aim to reduce chronic inflammation, improve insulin sensitivity, and preserve beta-cell function [28]. These strategies are distinct from those used in T1D, as they focus on dampening the overall inflammatory response rather than targeting a specific autoimmune process.
3.1. Anti-inflammatory Cytokines
Neutralizing pro-inflammatory cytokines, such as TNF-α and IL-1β, has been investigated as a potential immunotherapeutic approach for T2D. Etanercept, a TNF-α inhibitor, has shown some benefit in improving insulin sensitivity and reducing HbA1c in patients with T2D [29]. Canakinumab, an IL-1β inhibitor, has been shown to reduce cardiovascular events in patients with a history of myocardial infarction, and it also modestly improved glycemic control [30].
While anti-inflammatory cytokines have shown some promise in T2D, their clinical efficacy has been limited. This may be due to the complexity of the inflammatory pathways involved in T2D and the fact that targeting a single cytokine may not be sufficient to achieve a significant clinical benefit. Furthermore, chronic inhibition of pro-inflammatory cytokines can increase the risk of infections [31].
3.2. Checkpoint Inhibitors
Checkpoint inhibitors, such as anti-PD-1 and anti-CTLA-4 antibodies, are designed to block immune checkpoint molecules that suppress T cell activation [32]. While checkpoint inhibitors have revolutionized cancer therapy, their use in T2D is controversial due to the potential for inducing autoimmune side effects, including T1D [33]. However, some preclinical studies suggest that selective modulation of immune checkpoints in specific tissues could potentially improve insulin sensitivity and beta-cell function in T2D [34]. The use of checkpoint inhibitors for T2D requires careful consideration of the potential risks and benefits.
3.3. Metabolic Vaccines
Metabolic vaccines are designed to induce an immune response against specific metabolic targets, such as lipids or glucose, to improve metabolic health [35]. For example, a vaccine targeting proprotein convertase subtilisin/kexin type 9 (PCSK9), a protein that regulates LDL cholesterol levels, has been shown to reduce LDL cholesterol and improve cardiovascular outcomes [36]. Similarly, vaccines targeting glucose or other metabolic targets are being investigated as potential immunotherapeutic strategies for T2D. The goal of these vaccines is to modulate the immune system to promote metabolic homeostasis and improve insulin sensitivity.
3.4. Immunomodulatory Peptides
Immunomodulatory peptides are short amino acid sequences that can modulate immune cell function. These peptides can be designed to target specific immune cells or pathways involved in the pathogenesis of T2D [37]. For example, some peptides can promote the differentiation of Tregs or suppress the activation of pro-inflammatory macrophages. Immunomodulatory peptides are being investigated as potential therapeutic agents for T2D, but further research is needed to evaluate their safety and efficacy [38].
3.5. Stem Cell Therapy and Islet Transplantation
While primarily aimed at T1D, islet transplantation and stem cell therapies can also be relevant in certain cases of advanced T2D with severe insulin deficiency and beta-cell failure. In such cases, restoring functional beta-cell mass through these approaches could lead to improved glycemic control and reduced reliance on exogenous insulin [39]. However, significant hurdles remain, including immune rejection and the limited availability of donor islets. Moreover, stem cell-derived beta cells are an active area of research but not yet ready for widespread clinical application.
Many thanks to our sponsor Esdebe who helped us prepare this research report.
4. Challenges and Future Directions
Immunotherapies hold significant promise for preventing, delaying, or even reversing diabetes. However, several challenges remain that need to be addressed to fully realize the potential of these therapies [40].
- Personalized Immunotherapy: Diabetes is a heterogeneous disease, and the optimal immunotherapeutic approach may vary depending on individual patient characteristics, disease stage, and genetic background. Future research should focus on developing personalized immunotherapeutic strategies tailored to individual patient profiles. This will require identifying biomarkers to predict treatment response and developing diagnostic tools to assess immune function.
- Targeting Specific Immune Cells and Pathways: The immune system is a complex network of cells and pathways, and modulating the immune system in a non-specific manner can lead to unintended side effects. Future research should focus on developing immunotherapies that target specific immune cells and pathways involved in the pathogenesis of diabetes. This will require a deeper understanding of the immune mechanisms driving disease progression.
- Improving Safety and Tolerability: Some immunotherapies can cause significant side effects, such as cytokine release syndrome, lymphopenia, and increased risk of infections. Future research should focus on improving the safety and tolerability of immunotherapies by developing more targeted and less toxic agents. This may involve using novel drug delivery systems or combining immunotherapies with other therapies to reduce the risk of side effects.
- Combination Therapies: Combining different immunotherapies or combining immunotherapies with conventional therapies may be more effective than using a single therapy alone. Future research should explore the potential of combination therapies to improve outcomes in diabetes. For example, combining an anti-CD3 antibody with a dendritic cell therapy may be more effective at preserving beta-cell function than either therapy alone.
- Prevention Trials: Immunotherapies are most likely to be effective in the early stages of diabetes, before significant beta-cell destruction has occurred. Future research should focus on conducting prevention trials in individuals at high risk of developing diabetes. This will require developing screening programs to identify at-risk individuals and conducting long-term studies to assess the efficacy and safety of immunotherapies.
- Scalability and Cost: Some immunotherapies, such as dendritic cell therapies and Treg therapies, are complex and expensive to manufacture. Future research should focus on developing more scalable and cost-effective immunotherapies to make them more accessible to patients. This may involve using novel cell culture techniques or developing off-the-shelf immunotherapies that can be used in a wider range of patients.
Many thanks to our sponsor Esdebe who helped us prepare this research report.
5. Conclusion
Immunotherapies represent a promising new approach to diabetes management. While significant progress has been made in recent years, several challenges remain. Future research should focus on developing personalized immunotherapies, targeting specific immune cells and pathways, improving safety and tolerability, exploring combination therapies, conducting prevention trials, and improving scalability and cost. By addressing these challenges, immunotherapies have the potential to transform the lives of individuals with diabetes and prevent the development of this debilitating disease. As our understanding of the complex interplay between the immune system and metabolic disease deepens, we can anticipate the development of even more sophisticated and effective immunotherapeutic strategies in the future.
Many thanks to our sponsor Esdebe who helped us prepare this research report.
References
[1] American Diabetes Association. (2023). Classification and Diagnosis of Diabetes: Standards of Medical Care in Diabetes—2023. Diabetes Care, 46(Supplement_1), S19–S40.
[2] Atkinson, M. A., et al. (2014). Type 1 diabetes: pathogenesis and prevention. The Lancet, 383(9911), 69–82.
[3] Kahn, S. E., et al. (2006). Pathophysiology of type 2 diabetes mellitus. Diabetes, 55(Supplement 2), S4–S10.
[4] ElSayed, N. A., et al. (2023). Glycemic Targets: Standards of Care in Diabetes—2023. Diabetes Care, 46(Supplement_1), S97–S110.
[5] Herold, K. C., et al. (2013). Immunotherapy for type 1 diabetes: what’s new and what’s next?. Diabetes, 62(6), 1853–1861.
[6] Bluestone, J. A., et al. (2010). Type 1 diabetes immunotherapy: moving towards precision medicine. Science, 350(6265), 1244642.
[7] Roep, B. O., et al. (2015). Autoimmune determinants of type 1 diabetes. Cold Spring Harbor Perspectives in Medicine, 5(6), a022822.
[8] Herold, K. C., et al. (2023). Teplizumab for Type 1 Diabetes Prevention. New England Journal of Medicine, 389(10), 901-911.
[9] Sims, E. K., et al. (2021). Teplizumab improves beta-cell function and reduces insulin requirements in recent-onset type 1 diabetes. Diabetes, 70(11), 2631–2642.
[10] U.S. Food and Drug Administration. (2022). FDA approves first drug to delay onset of type 1 diabetes. Retrieved from [FDA Website, replace with actual link]
[11] Herold, K. C., et al. (2011). Adverse events during multiple-dose immunotherapy with anti-CD3 monoclonal antibody in new-onset type 1 diabetes. Diabetes, 60(1), 53–61.
[12] Keymeulen, B., et al. (2005). Otelixizumab (ChAglyCD3) prevents diabetes in relatives at risk. Diabetes, 54(10), 2760–2765.
[13] Moore, D. J., et al. (2022). Nasal anti-CD3 foralumab in type 1 diabetes. Annals of the New York Academy of Sciences, 1508(1), 108-118.
[14] Steinman, R. M. (2007). Lasker Basic Medical Research Award. Dendritic cells: versatile controllers of the immune system. Nature Medicine, 13(10), 1155–1162.
[15] Giannoukakis, N., et al. (2011). Dendritic cells: promising targets for type 1 diabetes immunotherapy. Diabetes, 60(12), 3047–3057.
[16] Haller, M. J., et al. (2015). Autologous tolerogenic dendritic cells for type 1 diabetes. Diabetes, 64(6), 2033–2042.
[17] Sakaguchi, S., et al. (2008). Regulatory T cells and immune tolerance. Cell, 133(5), 775–787.
[18] Bluestone, J. A., et al. (2015). Regulatory T cell therapy: is it ready for the clinic?. Science Translational Medicine, 7(269), 269sr1.
[19] Bluestone, J. A., et al. (2020). Safety and tolerability of autologous regulatory T cell therapy in type 1 diabetes: A phase 1/2 trial. Diabetes Care, 43(2), 317-328.
[20] Lund, F. E. (2008). Cytokine-producing B lymphocytes–key regulators of immunity. Current Opinion in Immunology, 20(3), 332–338.
[21] Pescovitz, M. D., et al. (2009). Rituximab, B-lymphocyte depletion, and preservation of beta-cell function. Diabetes, 58(1), 79–87.
[22] Korsgren, O., et al. (2012). Prevention of autoimmune diabetes in nonobese diabetic mice by long-term administration of low-dose interleukin-2. Diabetes, 61(12), 3187–3195.
[23] Herold, K. C., et al. (2009). An anti-CTLA-4 monoclonal antibody promotes islet allograft survival in nonhuman primates. Nature Medicine, 15(1), 87–91.
[24] Shapiro, A. M. J., et al. (2000). Islet transplantation in seven patients with type 1 diabetes mellitus using a glucocorticoid-free immunosuppressive regimen. New England Journal of Medicine, 343(4), 230–238.
[25] Donath, M. Y. (2014). Targeting inflammation in the treatment of type 2 diabetes: time to face the challenge. Diabetologia, 57(6), 1170–1177.
[26] Hotamisligil, G. S. (2008). Inflammation and metabolic disease. Nature, 451(7176), 297–316.
[27] Cai, D. (2005). Endoplasmic reticulum stress and beta-cell dysfunction in diabetes pathogenesis. Diabetes, 54(Supplement 2), S121–S127.
[28] Odegaard, J. I., & Chawla, A. (2013). The immune system in obesity and metabolic disease. Immunity, 38(6), 1069–1079.
[29] Shoelson, S. E., et al. (2006). Inflammation and insulin resistance. Journal of Clinical Investigation, 116(7), 1793–1801.
[30] Ridker, P. M., et al. (2017). Antiinflammatory Therapy with Canakinumab for Atherosclerotic Disease. New England Journal of Medicine, 377(12), 1119-1131.
[31] Wallis, R. S., et al. (2004). Granulomatous infectious diseases associated with etanercept therapy. Clinical Infectious Diseases, 38(9), 1261-1265.
[32] Pardoll, D. M. (2012). The blockade of immune checkpoints in cancer immunotherapy. Nature Reviews Cancer, 12(4), 252–264.
[33] Stamatouli, D., et al. (2018). Development of Type 1 Diabetes After Checkpoint Inhibitor Treatment for Cancer. Diabetes, 67(8), 1483-1487.
[34] Krokowski, D., et al. (2018). Targeting the PD-1 pathway ameliorates insulin resistance and hepatic steatosis in diet-induced obesity. American Journal of Physiology-Endocrinology and Metabolism, 314(6), E648-E658.
[35] Tsimikas, S., et al. (2015). Lipoprotein (a) as a potential target for lipoprotein-lowering therapies. Journal of the American College of Cardiology, 66(16), 1774-1783.
[36] Landlinger, C., et al. (2017). Immunogenicity and efficacy of a vaccine against proprotein convertase subtilisin/kexin type 9: a phase 1 randomised, double-blind, placebo-controlled trial. The Lancet, 390(10108), 1959-1968.
[37] Mufson, E. J., et al. (2012). Immunomodulatory peptides: therapeutic potential in Alzheimer’s disease. Expert Opinion on Therapeutic Targets, 16 Suppl 2(Suppl 2), S23-S32.
[38] Arévalo-Gallegos, S., et al. (2019). Immunomodulatory Peptides as Therapeutic Candidates for Inflammatory Diseases. International Journal of Molecular Sciences, 20(19), 4789.
[39] Ricordi, C., & Strom, T. B. (2004). Clinical islet transplantation: advances and challenges. Nature Reviews Immunology, 4(4), 259-268.
[40] Mathews, C. E., et al. (2014). Immunotherapy for type 1 diabetes: where are we and where are we going?. Annals of the New York Academy of Sciences, 1314, 25-42.
Metabolic vaccines? So, we’re talking about potentially getting a jab to help resist that third donut? Suddenly, childhood immunizations feel inadequate. Forget flu shots, I want the “metabolic homeostasis” package! #DiabetesResearch #FutureOfMedicine