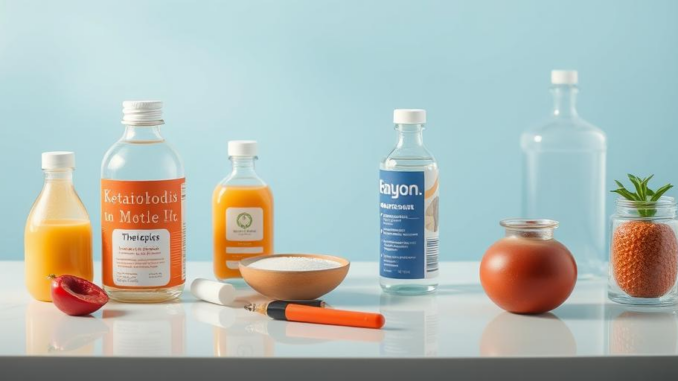
Abstract
Ketoacidosis (KA) is a metabolic state characterized by elevated levels of ketone bodies in the blood, resulting in metabolic acidosis. While Diabetic Ketoacidosis (DKA) is the most widely recognized form, KA arises from a spectrum of underlying conditions. This review offers a comprehensive exploration of ketoacidosis, expanding beyond DKA to encompass other etiologies, delving into the intricate pathophysiology, refining diagnostic approaches, evaluating current therapeutic strategies, and examining emerging therapies. This report focuses on the diverse causes of ketoacidosis, the nuances of differential diagnosis, and novel approaches to management, providing a valuable resource for clinicians and researchers involved in the care of patients with this complex metabolic derangement.
Many thanks to our sponsor Esdebe who helped us prepare this research report.
1. Introduction
Ketoacidosis represents a significant metabolic challenge characterized by the accumulation of ketone bodies (β-hydroxybutyrate, acetoacetate, and acetone) in the blood, leading to a decrease in blood pH and metabolic acidosis. While Diabetic Ketoacidosis (DKA), a severe complication of diabetes mellitus, dominates the clinical landscape, it is crucial to recognize that ketoacidosis can arise from various other conditions. These include alcoholic ketoacidosis (AKA), starvation ketoacidosis, and less commonly, ketoacidosis associated with certain medications and metabolic disorders. The underlying mechanisms, clinical presentations, and management strategies can vary substantially depending on the etiology.
The historical understanding of ketoacidosis has largely been shaped by DKA research. However, focusing solely on DKA risks overlooking the nuances and specific management considerations necessary for other forms of ketoacidosis. A comprehensive understanding of the metabolic pathways involved in ketone body production and utilization, as well as the factors that disrupt this balance, is essential for accurate diagnosis and effective treatment. This review aims to provide an in-depth exploration of ketoacidosis, moving beyond the established framework of DKA to encompass a broader perspective on its diverse etiologies, pathophysiology, diagnostic considerations, and therapeutic approaches.
Many thanks to our sponsor Esdebe who helped us prepare this research report.
2. Etiology and Classification of Ketoacidosis
Ketoacidosis can be classified based on its underlying cause. Understanding the specific etiology is paramount for guiding appropriate diagnostic and therapeutic interventions.
-
Diabetic Ketoacidosis (DKA): The most prevalent form, DKA results from an absolute or relative insulin deficiency, coupled with an excess of counter-regulatory hormones such as glucagon, catecholamines, cortisol, and growth hormone. This hormonal imbalance promotes hepatic gluconeogenesis and glycogenolysis, leading to hyperglycemia, and simultaneously stimulates lipolysis, resulting in increased free fatty acid flux to the liver. These free fatty acids are then converted into ketone bodies, exceeding the capacity of peripheral tissues to utilize them, leading to their accumulation in the blood. Precipitating factors often include infection, missed insulin doses, or undiagnosed diabetes.
-
Alcoholic Ketoacidosis (AKA): AKA typically occurs in individuals with a history of chronic alcohol abuse, often following a period of heavy drinking followed by cessation of alcohol intake and reduced food consumption. Alcohol metabolism disrupts hepatic redox balance, leading to increased NADH/NAD+ ratio, which inhibits gluconeogenesis and the citric acid cycle. Furthermore, ethanol inhibits insulin secretion, while stimulating the release of counter-regulatory hormones. The combination of these factors promotes lipolysis and ketone body production. Volume depletion and electrolyte imbalances, common in chronic alcoholics, further contribute to the pathogenesis of AKA. The frequent concurrence of other medical conditions, such as pancreatitis or liver disease, can complicate the clinical picture.
-
Starvation Ketoacidosis: Prolonged starvation or severe calorie restriction leads to depletion of liver glycogen stores. To meet energy demands, the body shifts to utilizing fatty acids as a primary fuel source. This results in increased lipolysis and subsequent ketone body production in the liver. Although blood glucose levels are typically lower in starvation ketoacidosis compared to DKA, the accumulation of ketone bodies can still lead to significant metabolic acidosis. This condition is often observed in individuals with eating disorders, malabsorption syndromes, or those undergoing prolonged fasting.
-
Medication-Induced Ketoacidosis: Certain medications can precipitate ketoacidosis. Sodium-glucose cotransporter-2 (SGLT2) inhibitors, commonly used in the treatment of type 2 diabetes, can induce euglycemic DKA. SGLT2 inhibitors promote glucosuria, which can lead to volume depletion and increased glucagon secretion. In individuals with reduced beta-cell function, this can trigger excessive lipolysis and ketone body production, despite relatively normal blood glucose levels. Other medications, such as corticosteroids and certain sympathomimetics, can also contribute to ketoacidosis by promoting insulin resistance and stimulating lipolysis. It’s important to be aware that in rare cases salicylates may also cause ketoacidosis.
-
Other Causes: Rare metabolic disorders, such as medium-chain acyl-CoA dehydrogenase (MCAD) deficiency, can impair fatty acid oxidation, leading to ketoacidosis, particularly during periods of fasting or illness. Inborn errors of metabolism that affect carbohydrate or amino acid metabolism can also contribute. Pregnant women may also experience ketoacidosis, often related to hormonal changes and increased energy demands. Conditions such as hyperemesis gravidarum may lead to ketoacidosis in pregnancy due to starvation and dehydration.
Many thanks to our sponsor Esdebe who helped us prepare this research report.
3. Pathophysiology of Ketoacidosis
The pathophysiology of ketoacidosis is a complex interplay of hormonal imbalances and metabolic alterations that ultimately lead to the accumulation of ketone bodies and a reduction in blood pH. Regardless of the specific etiology, several key mechanisms contribute to the development of ketoacidosis:
-
Insulin Deficiency/Resistance: Insulin plays a crucial role in regulating glucose metabolism and inhibiting lipolysis. In DKA, absolute or relative insulin deficiency impairs glucose uptake by peripheral tissues, leading to hyperglycemia. Simultaneously, insulin deficiency disinhibits lipolysis, resulting in increased free fatty acid release from adipose tissue. In other forms of ketoacidosis, such as AKA, while insulin levels may not be as drastically reduced as in DKA, the presence of insulin resistance or impaired insulin secretion contributes to the shift towards fatty acid metabolism.
-
Increased Counter-Regulatory Hormones: Glucagon, catecholamines, cortisol, and growth hormone antagonize the effects of insulin. These hormones promote hepatic gluconeogenesis and glycogenolysis, further elevating blood glucose levels. They also stimulate lipolysis, increasing the availability of free fatty acids for ketone body production. The imbalance between insulin and these counter-regulatory hormones is a central feature of ketoacidosis pathophysiology.
-
Lipolysis and Free Fatty Acid Metabolism: The increased flux of free fatty acids to the liver is a critical step in ketone body production. Inside the liver, free fatty acids undergo β-oxidation, generating acetyl-CoA. When the rate of acetyl-CoA production exceeds the capacity of the citric acid cycle to process it, acetyl-CoA is diverted towards ketogenesis. The liver converts acetyl-CoA into acetoacetate and β-hydroxybutyrate, the primary ketone bodies.
-
Ketone Body Metabolism and Excretion: Ketone bodies are acidic and can be used as an alternative fuel source by peripheral tissues, such as the brain and muscle. However, the rate of ketone body utilization is limited. When ketone body production exceeds the rate of utilization, they accumulate in the blood, leading to ketoacidemia. The kidneys attempt to compensate by excreting ketone bodies in the urine, resulting in ketonuria. However, this renal excretion is accompanied by the loss of sodium and potassium, contributing to electrolyte imbalances and dehydration.
-
Metabolic Acidosis: The accumulation of ketone bodies in the blood leads to a decrease in blood pH, resulting in metabolic acidosis. The body attempts to buffer the excess acid with bicarbonate, leading to a decrease in serum bicarbonate levels. Severe metabolic acidosis can impair cellular function and lead to life-threatening complications. Respiratory compensation occurs through hyperventilation (Kussmaul breathing), attempting to reduce the PaCO2 and partially correct the acid-base disturbance. However, this compensation is often insufficient to fully restore normal pH.
The relative contributions of these pathophysiological mechanisms can vary depending on the specific etiology of ketoacidosis. For example, in AKA, alcohol metabolism plays a significant role in altering the redox state of the liver and inhibiting gluconeogenesis, while in starvation ketoacidosis, glycogen depletion is the primary driver of increased lipolysis. Understanding these nuances is crucial for tailoring treatment strategies to address the specific underlying cause.
Many thanks to our sponsor Esdebe who helped us prepare this research report.
4. Diagnostic Criteria and Differential Diagnosis
The diagnosis of ketoacidosis requires a combination of clinical assessment and laboratory investigations. While the specific diagnostic criteria may vary slightly depending on the etiology, several key parameters are commonly used:
-
Blood Glucose: In DKA, blood glucose levels are typically elevated (often >250 mg/dL), reflecting impaired glucose utilization. However, euglycemic DKA, particularly associated with SGLT2 inhibitors, can occur with blood glucose levels within the normal range or only mildly elevated. In AKA and starvation ketoacidosis, blood glucose levels may be normal or even low.
-
Ketones: Elevated levels of ketone bodies in the blood or urine are a hallmark of ketoacidosis. Serum β-hydroxybutyrate is the preferred method for quantifying ketone levels due to its greater stability and abundance compared to acetoacetate. Urine ketone testing is less quantitative and can be affected by hydration status.
-
Arterial Blood Gas (ABG): An ABG analysis reveals metabolic acidosis, characterized by a low pH (typically <7.3), low bicarbonate level (typically <15 mEq/L), and a compensatory respiratory alkalosis (low PaCO2). The anion gap (calculated as [Na+] – [Cl-] – [HCO3-]) is typically elevated, reflecting the accumulation of unmeasured anions, primarily ketone bodies.
-
Electrolytes: Electrolyte imbalances are common in ketoacidosis. Serum sodium levels may be normal, elevated, or decreased, depending on the degree of dehydration and fluid shifts. Potassium levels can be initially normal or elevated due to intracellular shifting, but total body potassium is typically depleted due to urinary losses. Other electrolyte abnormalities, such as hypophosphatemia and hypomagnesemia, may also be present.
The differential diagnosis of ketoacidosis includes other causes of metabolic acidosis, such as lactic acidosis, renal failure, and ingestion of certain toxins (e.g., methanol, ethylene glycol, salicylate). Careful consideration of the patient’s history, clinical presentation, and laboratory findings is essential for accurate diagnosis. Specific clues may point to a particular etiology. For instance, a history of chronic alcohol abuse suggests AKA, while a history of SGLT2 inhibitor use raises suspicion for euglycemic DKA. Measuring lactate levels can help differentiate lactic acidosis from ketoacidosis. In cases of suspected toxin ingestion, specific toxicology testing is warranted.
Many thanks to our sponsor Esdebe who helped us prepare this research report.
5. Current Treatment Protocols
The primary goals of treatment for ketoacidosis are to correct dehydration, restore electrolyte balance, reverse metabolic acidosis, and address the underlying cause. The specific treatment approach may vary depending on the etiology and severity of the condition, but several core principles apply:
-
Fluid Resuscitation: Intravenous fluids are essential to correct dehydration and improve tissue perfusion. Isotonic saline (0.9% NaCl) is typically the initial fluid of choice. The rate of fluid administration should be guided by the patient’s hemodynamic status and degree of dehydration. Careful monitoring is necessary to avoid overhydration, particularly in patients with underlying cardiac or renal dysfunction.
-
Insulin Therapy (for DKA): In DKA, insulin therapy is crucial for suppressing lipolysis and promoting glucose utilization. A continuous intravenous infusion of regular insulin is typically initiated, with the dose adjusted based on blood glucose levels. The goal is to gradually lower blood glucose to prevent rapid shifts in osmolality, which can lead to cerebral edema. Once blood glucose reaches a target level (e.g., 200 mg/dL), dextrose is added to the intravenous fluids to prevent hypoglycemia.
-
Electrolyte Management: Potassium replacement is often necessary, as total body potassium is typically depleted. Potassium should be administered cautiously, with careful monitoring of serum potassium levels to avoid hyperkalemia. Phosphate and magnesium replacement may also be indicated, particularly in patients with severe electrolyte imbalances.
-
Bicarbonate Therapy: The use of bicarbonate therapy in ketoacidosis is controversial. While bicarbonate can temporarily raise blood pH, it may not improve clinical outcomes and can potentially worsen hypokalemia and intracellular acidosis. Bicarbonate therapy is generally reserved for patients with severe acidosis (pH < 7.0) or those with life-threatening hyperkalemia.
-
Addressing the Underlying Cause: Identifying and treating the underlying cause of ketoacidosis is crucial for preventing recurrence. This may involve treating infections, discontinuing offending medications, providing nutritional support, or managing other medical conditions. In AKA, thiamine supplementation is essential to prevent Wernicke-Korsakoff syndrome. Alcohol withdrawal symptoms should also be addressed with appropriate medications.
The management of ketoacidosis requires close monitoring of vital signs, blood glucose, electrolytes, and acid-base status. Frequent laboratory testing is necessary to guide treatment adjustments. The intensive care unit (ICU) is often the most appropriate setting for managing patients with severe ketoacidosis.
Many thanks to our sponsor Esdebe who helped us prepare this research report.
6. Emerging Therapies and Prevention Strategies
While the standard treatment protocols for ketoacidosis have been well-established, ongoing research is exploring novel therapies and prevention strategies to improve patient outcomes:
-
SGLT2 Inhibitor-Associated DKA Management: Specific protocols for managing euglycemic DKA associated with SGLT2 inhibitors are evolving. These protocols often involve higher insulin doses than typically used in DKA management, along with aggressive fluid resuscitation and carbohydrate supplementation to prevent hypoglycemia. Early recognition and prompt initiation of treatment are crucial.
-
Ketone Monitoring: Continuous glucose monitors (CGMs) have become increasingly popular for managing diabetes. Emerging technologies are also being developed to allow for continuous ketone monitoring. These devices could potentially provide earlier detection of ketoacidosis and facilitate proactive interventions to prevent its progression.
-
Prevention Strategies: Education is a key component of preventing DKA in diabetic patients. Patients should be educated about the importance of adherence to insulin therapy, blood glucose monitoring, and recognition of early warning signs of DKA. Sick-day management plans should be developed and regularly reviewed with patients. In individuals at risk for AKA, counseling on alcohol consumption and nutritional support is essential.
-
Novel Insulin Delivery Systems: Research is ongoing to develop novel insulin delivery systems, such as closed-loop insulin pumps (artificial pancreas), which can automatically adjust insulin doses based on continuous glucose monitoring data. These systems have the potential to improve glycemic control and reduce the risk of DKA.
-
Pharmacological Interventions: Some research is exploring the potential of pharmacological interventions to prevent or treat ketoacidosis. For example, certain medications may help to reduce lipolysis or improve ketone body utilization. However, these therapies are still in the early stages of development and require further investigation.
Many thanks to our sponsor Esdebe who helped us prepare this research report.
7. Conclusion
Ketoacidosis encompasses a spectrum of conditions characterized by metabolic acidosis and elevated ketone body levels. While DKA remains the most common and well-studied form, it is imperative to recognize the diverse etiologies of ketoacidosis, including AKA, starvation ketoacidosis, and medication-induced ketoacidosis. A thorough understanding of the underlying pathophysiology, diagnostic criteria, and appropriate treatment strategies is essential for optimal patient management.
Emerging therapies and prevention strategies, such as continuous ketone monitoring and novel insulin delivery systems, hold promise for improving outcomes in patients at risk for ketoacidosis. Continued research is needed to further refine our understanding of this complex metabolic derangement and develop more effective approaches to prevention and treatment. The increasing prevalence of SGLT2 inhibitor-associated euglycemic DKA necessitates ongoing education and awareness among clinicians and patients alike. A proactive and individualized approach to patient care, tailored to the specific etiology and severity of ketoacidosis, is crucial for improving patient outcomes and reducing the burden of this potentially life-threatening condition.
Many thanks to our sponsor Esdebe who helped us prepare this research report.
References
- Kitabchi AE, Umpierrez GE, Miles JM, Fisher JN. Hyperglycemic crises in adult patients with diabetes. Diabetes Care. 2009;32(7):1335-1343.
- Nyenwe EA, Kitabchi AE. Evidence-based management of hyperglycemic emergencies in diabetes mellitus. Diabetes Care. 2011;34(8):1895-1905.
- Westphal SA. Alcoholic ketoacidosis. Am J Med. 2006;119(3):e1-e2.
- Umpierrez GE, Murphy MB, Kitabchi AE. Diabetic ketoacidosis. Diabetes Metab Rev. 1997;13(1):15-41.
- Peters AL, Buschur EO, Buse JB, Cohan P, Diner JC, Hirsch IB. Euglycemic diabetic ketoacidosis: a potential complication of treatment with sodium-glucose cotransporter 2 inhibitors. Diabetes Care. 2015;38(9):1687-1693.
- Lalau JD. Ketoacidosis in starvation states: theoretical pathophysiology. Diabetes Metab. 1999;25(3):163-179.
- Gosmanov AR, Gosmanova EO, Kitabchi AE. Hyperglycemic crises: diabetic ketoacidosis (DKA), and hyperglycemic hyperosmolar state (HHS). In: De Groot LJ, Chrousos G, Dungan K, et al., editors. Endotext [Internet]. South Dartmouth (MA): MDText.com, Inc.; 2000-2023.
- Chiasson JL, Aris-Jilwan N, Bélanger R, et al. Diagnosis and treatment of diabetic ketoacidosis and the hyperglycemic hyperosmolar state. CMAJ. 2003;168(7):859-866.
- Dhatariya K, Umpierrez GE. Alcoholic ketoacidosis. Diagnosis and management. Clin Diabetes. 2006;24(4):155-163.
- Thomsen RW, Hundborg HH, Lambertsen KG, et al. Risk of diabetic ketoacidosis in patients using sodium-glucose cotransporter 2 inhibitors: a population-based case-control study. Diabetes Care. 2018;41(4):790-796.
- Munro JF, Campbell IW, McCuish AC, Duncan LJ. Euglycaemic diabetic ketoacidosis. Br Med J. 1973;2(5866):578-580.
- Kraft MD, Bota A, Cruz VT, et al. Acidemia-induced hyperkalemia revisited: a modern case series. J Intensive Care Med. 2013;28(1):51-57.
- van der Jagt EJ. Acid-base disturbances: classification, pathophysiology and treatment. Neth J Med. 2009;67(5):135-142.
- Fayfman M, Pasquel FJ, Umpierrez GE. Management of hyperglycemic crises: diabetic ketoacidosis and hyperglycemic hyperosmolar state. Med Clin North Am. 2017;101(3):587-606.
- Umpierrez GE, Jones S, Smiley D, et al. Insulin analogs versus human insulin in the treatment of diabetic ketoacidosis: a randomized controlled trial. Diabetes Care. 2009;32(7):1141-1146.
- Hillier TA, Abbott RD, Barrett EJ. Hyponatremia: evaluating the correction factor for hyperglycemia. Am J Med. 1999;106(4):399-403.
- Judah E, Isaacs D. Bicarbonate in diabetic ketoacidosis – a review. Intensive Care Med. 1992;18(4):191-195.
- Vellanki P, Umpierrez GE. Euglycemic diabetic ketoacidosis: mechanisms, challenges, and management. Am J Med Sci. 2020;359(5):259-265.
The mention of continuous ketone monitoring as an emerging technology is interesting. Could this advance, combined with telemedicine, significantly improve outcomes by enabling earlier intervention in at-risk populations, potentially reducing hospitalizations?
That’s a great point! The potential for continuous ketone monitoring alongside telemedicine is really exciting. Imagine the impact on rural or underserved communities, where access to specialists can be limited. Early detection and remote intervention could drastically change the trajectory of care and, as you mentioned, reduce hospitalizations. This is definitely an area ripe for innovation and research.
Editor: MedTechNews.Uk
Thank you to our Sponsor Esdebe