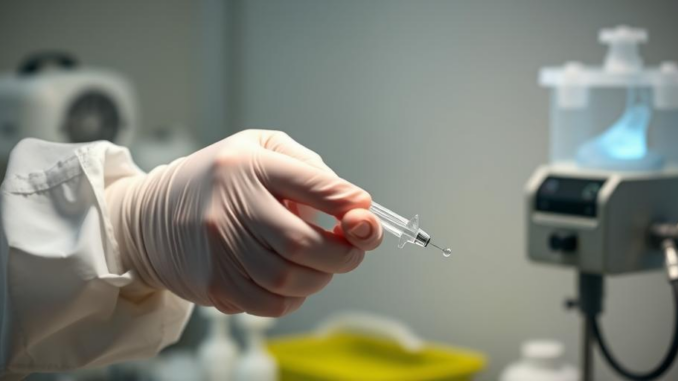
Navigating the Crossroads of Medical Device Sterilization: Innovation, Environmental Stewardship, and Regulatory Dynamics
Many thanks to our sponsor Esdebe who helped us prepare this research report.
Abstract
The sterilization of medical devices is a cornerstone of modern healthcare, preventing infections and ensuring patient safety. However, traditional sterilization methods, particularly ethylene oxide (EtO) sterilization, pose significant environmental and health risks. This research report provides a comprehensive overview of medical device sterilization technologies, evaluating their effectiveness, environmental impact, and regulatory context, with a particular focus on the role of the Environmental Protection Agency (EPA) in shaping sterilization practices. It examines established techniques such as EtO and gamma irradiation, as well as emerging alternatives like vaporized hydrogen peroxide (VHP), nitrogen dioxide sterilization (NO2), ultraviolet (UV) irradiation, and cold plasma sterilization. The report critically analyzes the challenges and opportunities associated with transitioning to more sustainable sterilization methods, encompassing technological innovation, infrastructure investment, supply chain adaptations, and the complexities of regulatory compliance. Furthermore, it explores the economic considerations, safety profiles, and limitations associated with each method. This report aims to provide a strategic overview for stakeholders, including medical device manufacturers, sterilization service providers, healthcare facilities, regulatory bodies, and researchers, to promote informed decision-making and facilitate the adoption of environmentally responsible and effective sterilization practices.
Many thanks to our sponsor Esdebe who helped us prepare this research report.
1. Introduction: The Imperative of Sterilization and its Evolving Landscape
The sterilization of medical devices is an indispensable process within healthcare, vital for mitigating the risks of healthcare-associated infections (HAIs) and ensuring patient safety. Sterilization, defined as the complete elimination of all forms of microbial life, is particularly critical for devices that come into contact with sterile body tissues or fluids. The stakes are high; inadequate sterilization can lead to severe infections, prolonged hospital stays, increased healthcare costs, and potentially fatal outcomes. Given the increasing complexity and sophistication of medical devices, alongside heightened awareness of environmental and occupational health hazards, the field of sterilization is undergoing a period of significant evolution and innovation.
Traditionally, sterilization has relied heavily on methods such as ethylene oxide (EtO) gas sterilization, gamma irradiation, and autoclaving (steam sterilization). While effective at achieving sterility, these methods present various drawbacks. EtO, for instance, is a known carcinogen and mutagen with documented environmental impacts, including ozone depletion and greenhouse gas emissions [1]. Gamma irradiation, while highly penetrative, raises concerns regarding the potential for material degradation and requires robust safety protocols to prevent radiation exposure. Autoclaving, while environmentally benign, is limited to devices that can withstand high temperatures and pressures, thus excluding a substantial portion of delicate or heat-sensitive instruments.
The growing emphasis on sustainability, coupled with increasingly stringent environmental regulations, has spurred the development and adoption of alternative sterilization technologies. These newer methods, including vaporized hydrogen peroxide (VHP) sterilization, nitrogen dioxide (NO2) sterilization, ultraviolet (UV) irradiation, electron beam (E-beam) sterilization and cold plasma sterilization, offer the potential to reduce environmental impact, improve worker safety, and sterilize a wider range of materials. However, the transition to these alternative methods is not without its challenges. It requires significant investment in new equipment, facility upgrades, and personnel training. Moreover, the regulatory approval process for new sterilization technologies can be complex and time-consuming, creating a barrier to widespread adoption. Finally, concerns regarding the efficacy and compatibility of these alternative methods with various medical device materials must be carefully addressed.
This research report aims to provide a comprehensive overview of the current state of medical device sterilization, examining both established and emerging technologies. It will delve into the environmental impact and regulatory landscape surrounding sterilization, with particular attention to the role of the EPA in shaping sterilization practices. By evaluating the challenges and opportunities associated with transitioning to more sustainable sterilization methods, this report will provide valuable insights for medical device manufacturers, sterilization service providers, healthcare facilities, regulatory bodies, and researchers seeking to navigate the complexities of this critical field.
Many thanks to our sponsor Esdebe who helped us prepare this research report.
2. Established Sterilization Technologies: Effectiveness, Limitations, and Environmental Footprint
2.1 Ethylene Oxide (EtO) Sterilization
EtO sterilization has been a mainstay of the medical device industry for decades due to its effectiveness in sterilizing a wide range of materials, including heat-sensitive polymers and complex device geometries. EtO’s mechanism of action involves alkylation of DNA and RNA, disrupting cellular function and leading to microbial death [2]. Despite its efficacy, EtO presents significant environmental and health risks. It is a known human carcinogen, and chronic exposure can cause respiratory irritation, neurological damage, and reproductive problems [3]. EtO is also a potent greenhouse gas, contributing to climate change. Furthermore, it poses an explosion hazard, necessitating strict safety protocols in sterilization facilities. The EPA has been actively working to reduce EtO emissions through regulatory actions, including revisions to the National Emission Standards for Hazardous Air Pollutants (NESHAP) [4]. These revisions mandate more stringent emission controls, such as catalytic oxidation or acid scrubbing, to reduce EtO releases from sterilization facilities. However, the high costs associated with these control technologies have raised concerns about the economic viability of EtO sterilization, particularly for smaller sterilization facilities. While advanced technologies exist to reduce EtO emissions, these can add substantially to the cost per sterilized device.
2.2 Gamma Irradiation
Gamma irradiation utilizes ionizing radiation from radioactive isotopes, typically Cobalt-60, to sterilize medical devices. Gamma radiation penetrates materials effectively, making it suitable for sterilizing large volumes of pre-packaged devices [5]. The mechanism of action involves the formation of free radicals, which damage DNA and other cellular components. While gamma irradiation is generally considered safe when properly controlled, it raises concerns about radiation exposure for workers and the potential for material degradation. Some polymers can become brittle or discolored after gamma irradiation, limiting its applicability for certain devices. Furthermore, the transportation and disposal of radioactive materials pose logistical and security challenges. Public perception of radiation also remains a significant hurdle, potentially leading to opposition to the construction or expansion of gamma irradiation facilities. The long half-life of Cobalt-60 necessitates careful management and eventual disposal as radioactive waste.
2.3 Steam Sterilization (Autoclaving)
Steam sterilization, or autoclaving, is a widely used and cost-effective sterilization method that employs saturated steam under pressure to achieve sterilization [6]. It is particularly effective for sterilizing reusable medical devices that can withstand high temperatures and pressures. The moist heat denatures proteins and other essential cellular components, leading to microbial death. Autoclaving is environmentally benign, producing no toxic emissions. However, its applicability is limited to heat- and moisture-stable materials. Many disposable medical devices, particularly those made from polymers, cannot withstand the high temperatures and pressures of autoclaving. Furthermore, complex device geometries may pose challenges for steam penetration, requiring careful validation of the sterilization process. Despite its limitations, autoclaving remains an important sterilization method for reusable medical devices and laboratory equipment. The cycle time can be relatively short compared to EtO, offering a faster turnaround.
Many thanks to our sponsor Esdebe who helped us prepare this research report.
3. Emerging Sterilization Technologies: Towards Sustainable Solutions
3.1 Vaporized Hydrogen Peroxide (VHP) Sterilization
VHP sterilization utilizes hydrogen peroxide in a vaporized form to achieve sterilization. It is a low-temperature sterilization method that is compatible with a wide range of materials, including heat-sensitive polymers [7]. The mechanism of action involves the oxidation of microbial cellular components by hydrogen peroxide. VHP is considered environmentally friendly because hydrogen peroxide decomposes into water and oxygen, leaving no toxic residues. However, VHP sterilization requires specialized equipment and careful control of humidity and temperature. The penetration of hydrogen peroxide vapor can be limited by complex device geometries or packaging materials, necessitating careful validation of the sterilization process. Furthermore, some materials, such as cellulose-based products, can absorb hydrogen peroxide, potentially affecting sterilization efficacy. The cycle times are typically longer than those of EtO or gamma irradiation. Despite these limitations, VHP sterilization is gaining increasing acceptance as a viable alternative to EtO, particularly for sterilizing medical devices in healthcare facilities and smaller sterilization facilities.
3.2 Nitrogen Dioxide (NO2) Sterilization
Nitrogen dioxide (NO2) sterilization is a relatively new low-temperature sterilization method that utilizes NO2 gas as the sterilant. NO2 sterilization offers several advantages, including rapid sterilization cycles, low residuals, and compatibility with a wide range of materials [8]. The mechanism of action involves the oxidation of microbial proteins and nucleic acids by NO2. NO2 is considered environmentally friendly, as it decomposes into nitrogen and oxygen. However, NO2 is a respiratory irritant, and strict safety precautions are necessary to prevent worker exposure. Furthermore, the penetration of NO2 gas can be limited by complex device geometries or packaging materials, requiring careful validation of the sterilization process. The technology is still relatively new, and more research is needed to fully understand its efficacy and compatibility with various medical device materials. NO2 sterilization systems are typically smaller and more self-contained than EtO systems, making them suitable for on-site sterilization in healthcare facilities or smaller sterilization facilities.
3.3 Ultraviolet (UV) Irradiation
UV irradiation utilizes ultraviolet light, typically in the UV-C range (200-280 nm), to sterilize surfaces and air. UV-C light damages DNA and RNA, preventing microbial replication [9]. UV irradiation is a rapid and environmentally friendly sterilization method that does not produce toxic residues. However, UV irradiation has limited penetration and is only effective for sterilizing surfaces directly exposed to the UV light. It is not suitable for sterilizing devices with complex geometries or packaged devices. UV irradiation is commonly used for disinfecting surfaces in healthcare facilities and for sterilizing air in operating rooms. Emerging applications include the use of UV irradiation for sterilizing personal protective equipment (PPE), such as N95 respirators, during pandemics. The effectiveness of UV sterilization is highly dependent on the intensity of the UV light, the exposure time, and the distance from the UV source. Shadows and occlusions can significantly reduce the effectiveness of UV sterilization.
3.4 Cold Plasma Sterilization
Cold plasma sterilization utilizes ionized gas at low temperatures to achieve sterilization. The plasma contains a mixture of ions, electrons, free radicals, and neutral species that interact with microbial cells, causing damage to their DNA and other cellular components [10]. Cold plasma sterilization is a low-temperature method that is compatible with a wide range of materials, including heat-sensitive polymers. It is considered environmentally friendly because it does not produce toxic residues. However, cold plasma sterilization requires specialized equipment and careful control of process parameters, such as gas composition, pressure, and power. The penetration of plasma can be limited by complex device geometries or packaging materials, necessitating careful validation of the sterilization process. Cold plasma sterilization is gaining increasing acceptance for sterilizing medical devices with complex geometries and heat-sensitive materials. Research is ongoing to optimize plasma parameters and improve the efficacy and penetration of cold plasma sterilization.
3.5 Electron Beam (E-Beam) Sterilization
E-beam sterilization is a radiation-based sterilization method that uses high-energy electrons to kill microorganisms. It is similar to gamma irradiation but uses an electron accelerator instead of a radioactive isotope. E-beam offers several advantages over gamma, including faster processing times, no radioactive materials, and the ability to turn the process on and off. However, E-beam has lower penetration depth than gamma radiation and may require special handling of certain materials. The environmental impact is lower than EtO and Gamma, with no chemical release or radioactive waste. The downside can include high up-front costs for the equipment and electricity use.
Many thanks to our sponsor Esdebe who helped us prepare this research report.
4. Regulatory Landscape and the Role of the EPA
The sterilization of medical devices is subject to stringent regulatory oversight to ensure patient safety and environmental protection. In the United States, the EPA plays a critical role in regulating emissions from sterilization facilities, particularly those using EtO [11]. The EPA’s regulations aim to reduce EtO emissions to the lowest achievable levels through the implementation of control technologies and work practice standards. The agency also evaluates the potential health risks associated with EtO exposure and develops risk management strategies to protect public health. The FDA, the primary regulatory body for medical devices, oversees the sterilization validation process and sets standards for sterility assurance. Medical device manufacturers must demonstrate that their sterilization processes are effective in achieving sterility and that the devices remain sterile throughout their shelf life. This typically involves rigorous testing and documentation to ensure compliance with FDA regulations.
The transition to more sustainable sterilization methods is also influenced by regulatory considerations. Medical device manufacturers must obtain regulatory approval for any changes to their sterilization processes, including the adoption of new sterilization technologies. This process can be complex and time-consuming, requiring extensive testing and validation data. Regulatory harmonization across different countries can also be a challenge, as sterilization standards and requirements may vary. Collaboration between regulatory bodies and industry stakeholders is essential to facilitate the adoption of innovative and sustainable sterilization practices. The FDA provides guidance on sterilization validation and offers incentives for the development of new sterilization technologies. The EPA provides technical assistance to sterilization facilities to help them comply with environmental regulations. The interplay between the EPA and FDA is critical, requiring manufacturers to consider both the sterilization effectiveness regulated by the FDA and the emissions and safety aspects regulated by the EPA.
Many thanks to our sponsor Esdebe who helped us prepare this research report.
5. Challenges and Opportunities in Transitioning to Sustainable Sterilization
The transition to more sustainable sterilization methods presents both significant challenges and promising opportunities. One of the main challenges is the high cost associated with investing in new equipment and infrastructure. Alternative sterilization technologies, such as VHP and NO2, often require specialized equipment and facilities, which can be a barrier for smaller sterilization facilities. Furthermore, the regulatory approval process for new sterilization technologies can be complex and time-consuming, adding to the overall cost. Another challenge is the need for personnel training. Operating and maintaining alternative sterilization equipment requires specialized knowledge and skills. Sterilization facilities must invest in training programs to ensure that their employees are competent in using the new technologies. Finally, the supply chain must be adapted to accommodate the use of alternative sterilization methods. Medical device manufacturers must work with their suppliers to ensure that materials and packaging are compatible with the chosen sterilization method.
Despite these challenges, the transition to more sustainable sterilization methods also presents significant opportunities. Adopting environmentally friendly sterilization technologies can improve a company’s reputation and enhance its brand image. Consumers are increasingly demanding sustainable products and services, and medical device manufacturers that prioritize sustainability can gain a competitive advantage. Furthermore, sustainable sterilization methods can reduce environmental impact and protect public health. By reducing EtO emissions, for example, sterilization facilities can help to improve air quality and reduce the risk of cancer. Finally, the transition to sustainable sterilization methods can drive innovation and create new business opportunities. Companies that develop and commercialize innovative sterilization technologies can benefit from growing demand for sustainable solutions. Opportunities can also be created by focusing on regionalized sterilization services that reduce transportation distances and improve response to supply chain disruptions.
Many thanks to our sponsor Esdebe who helped us prepare this research report.
6. Economic Considerations and Cost-Benefit Analysis
Economic considerations play a crucial role in the selection and implementation of medical device sterilization methods. A comprehensive cost-benefit analysis is essential to evaluate the economic feasibility of different sterilization options. This analysis should consider the initial investment costs, operating costs, maintenance costs, and potential environmental compliance costs. The initial investment costs include the cost of purchasing and installing the sterilization equipment. Operating costs include the cost of energy, consumables, and labor. Maintenance costs include the cost of routine maintenance and repairs. Environmental compliance costs include the cost of complying with environmental regulations, such as EtO emission limits.
In addition to these direct costs, the cost-benefit analysis should also consider the potential benefits of adopting more sustainable sterilization methods. These benefits include reduced environmental impact, improved worker safety, and enhanced brand image. The reduced environmental impact can be quantified by measuring the reduction in greenhouse gas emissions and toxic pollutants. Improved worker safety can be quantified by measuring the reduction in occupational injuries and illnesses. The enhanced brand image can be quantified by measuring the increase in sales and market share. By comparing the costs and benefits of different sterilization options, medical device manufacturers can make informed decisions about which methods to implement.
The total cost analysis must also consider the cost of failures in sterilization. Infections caused by improperly sterilized equipment can be incredibly expensive to treat, particularly in cases of antibiotic-resistant bacteria. In addition, product recalls due to sterilization failures can cause significant damage to brand reputation and create legal liabilities. Therefore, any cost-saving measures on sterilization processes should be carefully scrutinized to ensure they do not compromise sterility assurance.
Many thanks to our sponsor Esdebe who helped us prepare this research report.
7. Future Directions and Research Needs
The field of medical device sterilization is constantly evolving, with ongoing research aimed at developing more effective, safe, and sustainable sterilization methods. Future research should focus on several key areas. First, there is a need for more research on the efficacy and compatibility of emerging sterilization technologies, such as NO2 sterilization and cold plasma sterilization. More data are needed to fully understand the performance of these technologies and to identify their optimal applications. Second, there is a need for research on the development of new sterilization indicators and monitoring systems. These systems should be capable of providing real-time feedback on the sterilization process, allowing for better control and optimization. Third, there is a need for research on the development of new materials and packaging that are compatible with alternative sterilization methods. This research should focus on identifying materials that are resistant to degradation and that do not interfere with the sterilization process. Fourth, there is a need for research on the development of new methods for reducing EtO emissions from sterilization facilities. This research should focus on developing more efficient and cost-effective control technologies. Finally, there is a need for research on the development of new regulatory frameworks that promote the adoption of sustainable sterilization methods. These frameworks should provide incentives for companies to invest in new technologies and should streamline the regulatory approval process.
Specifically, research into advanced oxidation processes (AOPs) to further break down sterilant residuals holds great promise. AOPs involve the generation of highly reactive species, such as hydroxyl radicals, that can rapidly degrade organic pollutants. Research should also focus on the development of portable and point-of-use sterilization technologies, particularly for resource-limited settings. This could involve miniaturized sterilization systems or innovative approaches to disinfection and sterilization that do not require complex infrastructure. Nanomaterials with antimicrobial properties could also play a significant role in future sterilization strategies, either as coatings on medical devices or as components of sterilizing agents. However, careful consideration must be given to the potential toxicity and environmental fate of these nanomaterials. Addressing these research needs will be critical for advancing the field of medical device sterilization and ensuring the availability of safe, effective, and sustainable sterilization methods.
Many thanks to our sponsor Esdebe who helped us prepare this research report.
8. Conclusion
The sterilization of medical devices is a critical aspect of healthcare, ensuring patient safety and preventing infections. However, traditional sterilization methods, particularly EtO sterilization, pose significant environmental and health risks. The transition to more sustainable sterilization methods is essential for protecting public health and the environment. This transition presents both challenges and opportunities. Challenges include the high cost of investing in new equipment and infrastructure, the complexity of the regulatory approval process, and the need for personnel training. Opportunities include improved environmental performance, enhanced brand image, and the potential for innovation and new business opportunities.
The EPA plays a critical role in regulating emissions from sterilization facilities and in promoting the adoption of sustainable sterilization practices. Medical device manufacturers, sterilization service providers, healthcare facilities, regulatory bodies, and researchers must work together to facilitate the transition to more sustainable sterilization methods. This collaboration should focus on developing and implementing innovative sterilization technologies, streamlining the regulatory approval process, and providing incentives for companies to invest in sustainable solutions. By working together, stakeholders can ensure that medical devices are sterilized safely, effectively, and sustainably, protecting both patient health and the environment.
Many thanks to our sponsor Esdebe who helped us prepare this research report.
References
[1] U.S. Environmental Protection Agency. (2020). Integrated Risk Information System (IRIS): Ethylene Oxide. https://www.epa.gov/iris/ethylene-oxide
[2] McDonnell, G. (2017). Antisepsis, Disinfection, and Sterilization: Types, Action, and Resistance. John Wiley & Sons.
[3] National Institute for Occupational Safety and Health (NIOSH). (2016). Ethylene Oxide. https://www.cdc.gov/niosh/topics/ethyleneoxide/default.html
[4] Environmental Protection Agency. (2024). EPA Actions to Reduce Ethylene Oxide Emissions. https://www.epa.gov/hazardous-air-pollutants-ethylene-oxide/epa-actions-reduce-ethylene-oxide-emissions
[5] Tallentire, A., & Koskinen, W. J. (2003). Sterilization by Ionizing Radiation. In Disinfection, Sterilization, and Preservation (pp. 413-433). Lippincott Williams & Wilkins.
[6] Rutala, W. A., & Weber, D. J. (2016). Disinfection and Sterilization: What Clinicians Need to Know. Clinical Microbiology Reviews, 29(1), 125-154.
[7] Klapes, N. A., & Vesley, D. (1990). Vapor-Phase Hydrogen Peroxide as a Surface Decontaminant. Applied and Environmental Microbiology, 56(2), 503-506.
[8] Agalloco, J., & Akers, J. (2016). Sterile Drug Products: Process Engineering and Aseptic Manufacturing. Informa Healthcare.
[9] Kowalski, W. J. (2009). Ultraviolet Germicidal Irradiation Handbook: UVGI for Air and Surface Disinfection. Springer Science & Business Media.
[10] Laroussi, M., & Leipold, F. (2004). Nonthermal Plasma Sterilization: Overview of Principles, Mechanisms, and Applications. Applied Physics Reviews, 96(9), 3028-3041.
[11] Food and Drug Administration (FDA). (2024). Medical Device Sterilization. https://www.fda.gov/medical-devices/general-hospital-devices-and-supplies/medical-device-sterilization
[12] World Health Organization (WHO). (2018). Decontamination and reprocessing of medical devices for health-care facilities. https://www.who.int/publications/i/item/9789241514463
[13] European Medicines Agency (EMA). (2019). Guideline on sterilisation of medicinal products, active substances, excipients and primary packaging material. https://www.ema.europa.eu/en/documents/scientific-guideline/guideline-sterilisation-medicinal-products-active-substances-excipients-primary-packaging_en.pdf
[14] ISO 11135:2014. Sterilization of health-care products – Ethylene oxide – Requirements for development, validation and routine control of a sterilization process for medical devices. International Organization for Standardization.
[15] ISO 11137-1:2006. Sterilization of health care products – Radiation – Part 1: Requirements for development, validation and routine control of a sterilization process for medical devices. International Organization for Standardization.
[16] ISO 14937:2009. Sterilization of health care products – General requirements for characterization of a sterilizing agent and the development, validation and routine control of a sterilization process for medical devices. International Organization for Standardization.
[17] Joshi, R., Adhikari, M., & Bohara, A. (2023). Cold plasma: an innovative method for medical device sterilization. Applied Microbiology and Biotechnology, 107(18), 5669–5685.
[18] Wozniak, A., Stachowiak, A., & Szymanowski, M. (2023). Low-Temperature Plasma Sterilization for Medical Instruments—Overview. Materials, 16(15), 5378.
[19] US EPA. (2024). Control of Air Pollution From Ethylene Oxide Commercial Sterilization and Fumigation Operations. Federal Register, 89(72), 26064-26164
[20] OECD (2023), Sustainable Materials Management: Making Resource Use More Efficient in a Circular Economy, OECD Publishing, Paris,
https://doi.org/10.1787/ca735c4b-en.
Sterilization AND environmental stewardship? Talk about a tough crowd! I’m suddenly picturing medical devices lining up for their “green” report cards. Wonder if they’ll start offering carbon-neutral syringes soon?
That’s a great image! The idea of “green report cards” for medical devices is both amusing and thought-provoking. Perhaps focusing on reusable components or innovative material choices could pave the way for those carbon-neutral syringes you mentioned. What are your thoughts on material innovation?
Editor: MedTechNews.Uk
Thank you to our Sponsor Esdebe
So, if we start irradiating devices with electron beams, are we one step closer to Star Trek’s medical tricorder? Just imagine waving a wand for instant sterilization. Beam me up, Scotty… but only if you’ve sterilized the transporter!
That’s a fun thought! The speed of E-beam sterilization definitely moves us in that direction. Imagine a future where handheld devices ensure immediate sterility in operating rooms or even remote field hospitals. The possibilities for rapid and reliable sterilization are exciting! What other sterilization tech do you think could find a place in a future medical tricorder?
Editor: MedTechNews.Uk
Thank you to our Sponsor Esdebe
Given the challenges surrounding EtO, what advancements in closed-loop systems or alternative chemistries might mitigate its environmental impact while maintaining sterilization efficacy for complex devices?