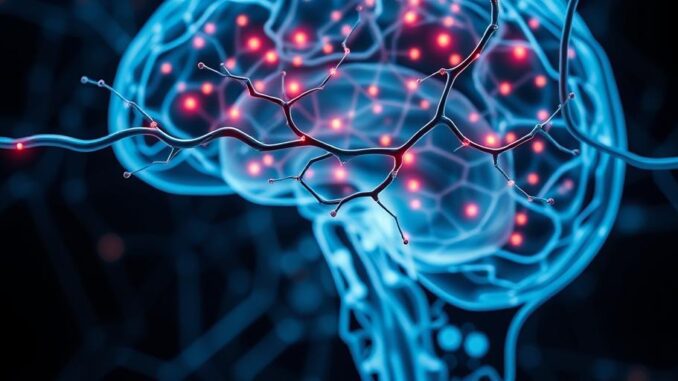
Abstract
Neuroprosthetics represents a rapidly evolving field at the intersection of neuroscience, engineering, and medicine, offering the potential to restore lost sensory and motor functions and treat neurological disorders. This report provides a comprehensive overview of neuroprosthetics, encompassing its historical development, diverse types (motor, sensory, and cognitive), underlying technologies, current challenges, ethical considerations, and promising future directions. We delve into the complexities of biocompatibility, signal processing, decoding algorithms, and closed-loop control systems. Furthermore, we explore the ethical landscape surrounding neuroprosthetic use, including issues of autonomy, identity, and accessibility. Finally, we discuss the potential of emerging technologies such as non-invasive brain-computer interfaces, personalized neuroprosthetic therapies, and advanced materials to revolutionize the field and improve the lives of individuals with neurological impairments.
Many thanks to our sponsor Esdebe who helped us prepare this research report.
1. Introduction
The field of neuroprosthetics has witnessed remarkable advancements in recent decades, fueled by progress in neuroscience, microfabrication, materials science, and computer science. Neuroprosthetics aims to restore lost neurological function by directly interfacing with the nervous system, either to bypass damaged pathways or to modulate neural activity. This is achieved through devices that record neural signals, process these signals to extract relevant information, and then use this information to control external devices or stimulate the nervous system. These devices represent a sophisticated application of brain-computer interfaces (BCIs) to improve the lives of individuals affected by a wide range of neurological disorders, including paralysis, sensory deficits, and cognitive impairments. The scope of neuroprosthetics extends beyond simply replacing lost function; increasingly, research focuses on neuromodulation and neural repair, opening possibilities for treating conditions such as Parkinson’s disease, epilepsy, and chronic pain.
The development of neuroprosthetics is driven by several factors, including an aging population with increasing rates of neurodegenerative diseases, a greater understanding of the brain’s plasticity and ability to adapt to artificial interfaces, and technological advances that make it possible to create more sophisticated and biocompatible devices. The convergence of these factors has created a fertile ground for innovation and has spurred significant investment in research and development. However, substantial challenges remain, including improving the long-term stability and biocompatibility of implanted devices, developing more robust and reliable decoding algorithms, and addressing the ethical implications of manipulating the nervous system. This report aims to provide a comprehensive overview of the current state of neuroprosthetics, highlight the major challenges and opportunities, and discuss the ethical considerations that must be addressed as the field continues to evolve.
Many thanks to our sponsor Esdebe who helped us prepare this research report.
2. Historical Development
The history of neuroprosthetics is marked by gradual but significant progress, building upon earlier discoveries in neuroscience and bioengineering. The concept of electrically stimulating the nervous system dates back to the 18th century, with Luigi Galvani’s experiments on frogs’ legs. However, it was not until the mid-20th century that the first practical neuroprosthetic devices began to emerge.
One of the earliest examples is the cochlear implant, which was initially developed in the 1950s and has since become a widely successful neuroprosthetic device for restoring hearing in individuals with severe hearing loss. The cochlear implant works by directly stimulating the auditory nerve, bypassing damaged hair cells in the inner ear. This success paved the way for further research into other types of neuroprosthetics.
In the 1960s and 1970s, researchers began to explore the possibility of using electrical stimulation to restore motor function. Giles Brindley’s pioneering work on bladder control using sacral anterior root stimulation marked a significant step forward. Around the same time, researchers were also investigating the use of BCIs to control external devices, such as computer cursors and robotic arms. These early BCIs relied on electroencephalography (EEG) signals recorded from the scalp, which provided a non-invasive but relatively low-resolution measure of brain activity.
The development of microelectrode arrays in the late 20th and early 21st centuries enabled researchers to record neural activity with much greater precision and to stimulate individual neurons or small groups of neurons. This led to significant advancements in the development of motor prosthetics, allowing individuals with paralysis to control robotic arms and hands with increasing dexterity. Sensory prosthetics also benefited from these advances, with the development of retinal prostheses aimed at restoring vision in individuals with certain types of blindness. The development of deep brain stimulation (DBS) also represented a major milestone. Originally developed for treating tremor, DBS has now become a standard therapy for a variety of neurological disorders, including Parkinson’s disease, dystonia, and essential tremor.
Many thanks to our sponsor Esdebe who helped us prepare this research report.
3. Types of Neuroprosthetics
Neuroprosthetics can be broadly classified into three main categories: motor, sensory, and cognitive. Each type targets different aspects of neurological function and utilizes distinct technological approaches.
3.1. Motor Neuroprosthetics
Motor neuroprosthetics aim to restore movement in individuals with paralysis or motor impairments. These devices typically involve recording neural signals from motor areas of the brain, such as the motor cortex, and using these signals to control external devices, such as robotic arms, exoskeletons, or computer interfaces. Different approaches exist for recording neural signals, including invasive methods such as microelectrode arrays and non-invasive methods such as EEG. Invasive methods provide higher resolution and signal quality but carry the risk of tissue damage and inflammation. Non-invasive methods are safer but offer lower resolution and are more susceptible to noise.
Decoding algorithms play a crucial role in motor neuroprosthetics. These algorithms translate neural signals into commands that can be used to control external devices. The complexity of these algorithms depends on the number of degrees of freedom that the device needs to control and the desired level of precision. Closed-loop control systems are also becoming increasingly important in motor neuroprosthetics. These systems provide feedback to the user based on the performance of the device, allowing them to make adjustments and improve their control. For example, visual feedback from a robotic arm can help the user to refine their movements and achieve greater accuracy.
3.2. Sensory Neuroprosthetics
Sensory neuroprosthetics aim to restore lost sensory function, such as vision, hearing, or touch. These devices typically involve stimulating sensory areas of the brain or peripheral nerves to evoke the desired sensation. Examples include cochlear implants, retinal prostheses, and tactile prostheses.
Cochlear implants, as mentioned previously, are a highly successful example of sensory neuroprosthetics. Retinal prostheses aim to restore vision in individuals with certain types of blindness, such as retinitis pigmentosa and age-related macular degeneration. These devices typically involve implanting a microelectrode array onto the retina to stimulate retinal ganglion cells, which transmit visual information to the brain. While retinal prostheses have shown some promise, the level of visual acuity that can be achieved is still limited.
Tactile prostheses aim to restore the sense of touch in individuals with limb amputations or spinal cord injuries. These devices typically involve stimulating sensory nerves in the skin or spinal cord to evoke tactile sensations. Research in this area is still in its early stages, but promising results have been obtained using methods such as microstimulation of the somatosensory cortex and peripheral nerve stimulation. One of the key challenges in tactile prosthetics is creating realistic and nuanced tactile sensations that can provide useful feedback to the user.
3.3. Cognitive Neuroprosthetics
Cognitive neuroprosthetics represent a more nascent area of research, focusing on restoring or enhancing cognitive functions such as memory, attention, and decision-making. These devices aim to modulate neural activity in brain regions involved in these cognitive processes. Approaches include deep brain stimulation (DBS), transcranial magnetic stimulation (TMS), and transcranial direct current stimulation (tDCS).
DBS has shown some promise in treating cognitive disorders such as obsessive-compulsive disorder (OCD) and Tourette’s syndrome. Researchers are also exploring the use of DBS to improve memory function in individuals with Alzheimer’s disease. TMS and tDCS are non-invasive techniques that can be used to modulate neural activity in specific brain regions. These techniques are being investigated as potential treatments for a range of cognitive disorders, including depression, anxiety, and ADHD.
Cognitive neuroprosthetics face several challenges, including the complexity of cognitive processes and the difficulty of targeting specific brain regions with sufficient precision. Furthermore, the ethical implications of manipulating cognitive function are significant and require careful consideration.
Many thanks to our sponsor Esdebe who helped us prepare this research report.
4. Underlying Technologies
The development of neuroprosthetics relies on a range of underlying technologies, including:
4.1. Biocompatible Materials
Biocompatibility is a critical factor in the design of neuroprosthetic devices. Implanted devices must be made from materials that do not elicit a strong immune response or cause damage to surrounding tissue. Materials commonly used in neuroprosthetics include titanium, platinum, iridium, and polymers such as polyimide and parylene. Researchers are also exploring the use of novel materials, such as carbon nanotubes and graphene, which offer unique electrical and mechanical properties.
One of the major challenges in biocompatibility is the formation of a glial scar around the implanted device. The glial scar is a dense layer of glial cells that forms in response to tissue damage. It can impede the transmission of neural signals and reduce the effectiveness of the device. Researchers are investigating various strategies to minimize glial scar formation, including surface modification of the device, drug delivery, and the use of biodegradable materials.
4.2. Microfabrication Techniques
Microfabrication techniques are essential for creating the small, complex structures required for neuroprosthetic devices. These techniques include photolithography, etching, and thin-film deposition. Microfabrication allows for the creation of microelectrode arrays with high density and precise spacing between electrodes. It also enables the integration of microfluidic channels for drug delivery and the creation of complex 3D structures.
Advances in microfabrication are enabling the development of more sophisticated neuroprosthetic devices with improved performance and functionality. For example, researchers are developing flexible microelectrode arrays that can conform to the curved surface of the brain, reducing tissue damage and improving signal quality.
4.3. Signal Processing and Decoding Algorithms
Signal processing and decoding algorithms are crucial for extracting relevant information from neural signals and translating this information into commands that can be used to control external devices or stimulate the nervous system. These algorithms typically involve a combination of techniques, including filtering, feature extraction, and machine learning.
Filtering is used to remove noise and artifacts from the neural signals. Feature extraction involves identifying relevant patterns in the neural signals that can be used to distinguish between different movements or cognitive states. Machine learning algorithms are used to train the system to recognize these patterns and to predict the user’s intent. The performance of decoding algorithms is critical for the success of neuroprosthetics. Researchers are continuously developing new and improved algorithms that can provide more accurate and reliable control.
4.4. Closed-Loop Control Systems
Closed-loop control systems provide feedback to the user based on the performance of the device, allowing them to make adjustments and improve their control. This feedback can be visual, auditory, or tactile. Closed-loop control systems are becoming increasingly important in neuroprosthetics, as they can significantly improve the user’s ability to control the device and achieve the desired outcome. These systems require sensors to detect the position, velocity, and force of the device, as well as algorithms to process this information and provide appropriate feedback to the user.
Many thanks to our sponsor Esdebe who helped us prepare this research report.
5. Current Challenges
Despite the significant advancements in neuroprosthetics, several challenges remain that need to be addressed to improve the efficacy and accessibility of these devices.
5.1. Biocompatibility and Long-Term Stability
One of the major challenges is maintaining the biocompatibility and long-term stability of implanted devices. The glial scar formation, as mentioned previously, can degrade the performance of the device over time. Furthermore, the mechanical mismatch between the device and the surrounding tissue can cause tissue damage and inflammation. Developing materials and designs that minimize these effects is crucial for improving the long-term stability of neuroprosthetic devices. This area often involves advanced materials science and surface modification techniques to promote neural integration and minimize immune response.
5.2. Signal Processing and Decoding Accuracy
Improving the accuracy and robustness of signal processing and decoding algorithms is another major challenge. Neural signals are often noisy and variable, making it difficult to extract relevant information. Furthermore, the brain’s activity can change over time, requiring the decoding algorithms to be constantly adapted. Developing more sophisticated algorithms that can handle these challenges is essential for improving the performance of neuroprosthetics. Advanced machine learning techniques, including deep learning, are being explored to enhance decoding accuracy and adaptability.
5.3. Power Consumption and Miniaturization
Reducing the power consumption and size of neuroprosthetic devices is also important. Implantable devices need to be powered by batteries or wireless power transfer systems. Reducing the power consumption can extend the battery life and reduce the size of the device. Miniaturization is important for making the device less invasive and more comfortable for the user. The development of energy-efficient circuits and microfabrication techniques is crucial for addressing these challenges. Advances in microelectronics and wireless power transfer technologies are enabling the creation of smaller and more efficient neuroprosthetic devices.
5.4. Clinical Translation and Regulatory Hurdles
Translating neuroprosthetic research into clinical practice faces several challenges. Clinical trials are expensive and time-consuming. Furthermore, regulatory agencies require rigorous testing to ensure the safety and efficacy of neuroprosthetic devices. Streamlining the regulatory approval process and reducing the cost of clinical trials is important for accelerating the translation of research into clinical practice. Collaboration between researchers, clinicians, and industry is essential for overcoming these hurdles. Developing standardized testing protocols and establishing clear regulatory guidelines can facilitate the clinical translation of neuroprosthetic technologies.
Many thanks to our sponsor Esdebe who helped us prepare this research report.
6. Ethical Considerations
The development and use of neuroprosthetics raise several ethical considerations that need to be carefully addressed.
6.1. Autonomy and Control
One of the main concerns is the potential impact of neuroprosthetics on the user’s autonomy and control. If the device malfunctions or is hacked, the user could lose control of their movements or thoughts. Furthermore, the device could be used to manipulate the user’s behavior against their will. Safeguarding the user’s autonomy and control is crucial. Security measures must be implemented to prevent unauthorized access to the device. Furthermore, the user should have the ability to turn off the device at any time.
6.2. Identity and Authenticity
Neuroprosthetics could potentially alter the user’s sense of identity and authenticity. If the device becomes an integral part of the user’s body and mind, it could be difficult to distinguish between the user’s natural abilities and the abilities provided by the device. This could raise questions about the user’s self-perception and their relationship with others. The psychological impact of neuroprosthetics on the user’s identity needs to be carefully considered.
6.3. Accessibility and Equity
Neuroprosthetics are currently expensive and not readily accessible to everyone who could benefit from them. This raises concerns about equity and social justice. Ensuring that neuroprosthetics are affordable and accessible to all who need them is crucial. Government funding and private investment can play a role in reducing the cost of these devices. Furthermore, efforts should be made to develop neuroprosthetics that are culturally sensitive and meet the needs of diverse populations.
6.4. Dual Use and Military Applications
The technologies used in neuroprosthetics could also be used for military purposes, such as enhancing soldiers’ performance or developing brain-controlled weapons. The potential for dual use raises ethical concerns about the misuse of these technologies. International agreements and ethical guidelines are needed to prevent the development and deployment of neuroprosthetics for military applications.
Many thanks to our sponsor Esdebe who helped us prepare this research report.
7. Future Trends
The field of neuroprosthetics is rapidly evolving, with several promising trends emerging.
7.1. Non-Invasive Brain-Computer Interfaces
Non-invasive BCIs, such as EEG, are becoming increasingly sophisticated. Advances in signal processing and machine learning are improving the accuracy and reliability of these devices. Non-invasive BCIs offer a safer and more accessible alternative to invasive BCIs. These technologies hold promise for a wide range of applications, including communication, rehabilitation, and gaming. However, non-invasive BCIs still face challenges in terms of signal quality and spatial resolution.
7.2. Personalized Neuroprosthetic Therapies
The development of personalized neuroprosthetic therapies is another promising trend. Each individual’s brain is unique, and the optimal neuroprosthetic device and therapy protocol will vary accordingly. Personalized therapies involve tailoring the device and therapy to the individual’s specific needs and brain characteristics. This approach has the potential to significantly improve the efficacy of neuroprosthetics. Advances in neuroimaging and computational modeling are enabling the development of more personalized neuroprosthetic therapies.
7.3. Advanced Materials and Nanotechnology
Advanced materials and nanotechnology are playing an increasingly important role in neuroprosthetics. Nanomaterials, such as carbon nanotubes and graphene, offer unique electrical and mechanical properties that make them ideal for use in neuroprosthetic devices. Nanotechnology can be used to create nanoscale sensors and actuators that can interact with individual neurons. These technologies have the potential to revolutionize the field of neuroprosthetics. Nanomaterials can be used to create more biocompatible and long-lasting implants. Furthermore, nanotechnology can be used to deliver drugs and genes directly to the brain.
7.4. Closed-Loop Neuromodulation
Closed-loop neuromodulation involves using feedback from the brain to adjust the parameters of the stimulation in real time. This approach can improve the efficacy and safety of neuromodulation therapies. Closed-loop systems can adapt to changes in the brain’s activity and optimize the stimulation parameters for each individual. These systems have the potential to treat a wide range of neurological and psychiatric disorders. Real-time feedback mechanisms are essential for optimizing the therapeutic effects of neuromodulation.
Many thanks to our sponsor Esdebe who helped us prepare this research report.
8. Conclusion
Neuroprosthetics holds immense promise for restoring lost neurological function and improving the lives of individuals with neurological disorders. The field has made significant advancements in recent decades, driven by progress in neuroscience, engineering, and medicine. However, several challenges remain, including improving the biocompatibility and long-term stability of implanted devices, developing more robust and reliable decoding algorithms, and addressing the ethical implications of manipulating the nervous system. The future of neuroprosthetics looks bright, with promising trends emerging such as non-invasive brain-computer interfaces, personalized neuroprosthetic therapies, and advanced materials and nanotechnology. Continued research and development, coupled with careful consideration of ethical implications, will be essential for realizing the full potential of neuroprosthetics.
Many thanks to our sponsor Esdebe who helped us prepare this research report.
References
- Andersen, R. A., Burdick, J. W., Cui, X., Harris, G. C., Klausner, J. F., Li, Q., … & Mahmoudi, B. (2010). BMI-supported arm movements. Neuron, 67(4), 539-543.
- Borton, D., Johnson, L. A., Kipke, D. R., & McFadden, M. L. (2013). Bridging the gap: linking preclinical animal models to human neuroprosthetics. Journal of Neuroscience Methods, 212(1), 58-68.
- Bouton, C. E., Shaikhouni, A., Hutchinson, D., Mullins, L., Hammond, T. K., Friedenberg, D. A., … & Rezai, A. R. (2016). Restoring cortical control of paralyzed upper limbs through an implantable brain–computer interface. Nature, 533(7602), 247-250.
- Lebedev, M. A., & Nicolelis, M. A. (2006). Brain-machine interfaces: past, present and future. Trends in Neurosciences, 29(9), 536-546.
- Merzenich, M. M., Van Vreeswijk, C., Mahani, A. S., Newbury, T. J., Bottge, A., Kaizu, H., … & Nahum, M. (2014). Brain plasticity-based therapeutics. Frontiers in Human Neuroscience, 8, 762.
- Nicolelis, M. A. (2003). Brain–machine interfaces to restore motor function and probe neural circuits. Nature Reviews Neuroscience, 4(5), 417-422.
- Rieth, L. W., Laeseke, C. A., Miller, L. E., & Solla, S. A. (2011). Bayesian estimation of neural network connectivity. Journal of Neurophysiology, 106(2), 911-923.
- Warwick, K. (2004). Cyborg 1.0: Theory and practice. Cybernetics and Systems, 35(3), 229-260.
- Wolpaw, J. R., Birbaumer, N., McFarland, D. J., Pfurtscheller, G., & Vaughan, T. M. (2002). Brain–computer interfaces for communication and control. Clinical Neurophysiology, 113(6), 767-791.
The discussion on ethical considerations is vital. The potential impact on autonomy and identity needs careful, ongoing dialogue as neuroprosthetics advance, ensuring user well-being remains paramount.
Absolutely! The ethical considerations are definitely at the forefront as we push the boundaries of neuroprosthetics. The points you raise about autonomy and identity are incredibly important. It’s crucial that researchers, ethicists, and users collaborate to establish guidelines that prioritize user well-being and address potential societal impacts as this tech evolves. Let’s keep the conversation going!
Editor: MedTechNews.Uk
Thank you to our Sponsor Esdebe
The discussion of biocompatible materials is critical. What advancements are being made to address the glial scar formation, and how close are we to seeing significant improvements in long-term implant stability and performance?
That’s a great point! Research into materials like bioactive glass and porous titanium is showing promise in promoting better integration and reducing glial scarring. While we’re seeing progress, long-term human trials are crucial to fully validate these advancements and their impact on implant longevity. It’s a complex puzzle!
Editor: MedTechNews.Uk
Thank you to our Sponsor Esdebe
Cognitive neuroprosthetics enhancing memory – intriguing! Imagine never forgetting a name again. Though, would you still have an excuse for forgetting birthdays? Asking for a friend… who *definitely* remembers yours.
That’s a fun thought! While remembering everything would be amazing, maybe a little selective forgetting keeps things interesting. Perhaps future cognitive neuroprosthetics will offer customizable memory filters. What do you think, would that be a desirable feature?
Editor: MedTechNews.Uk
Thank you to our Sponsor Esdebe