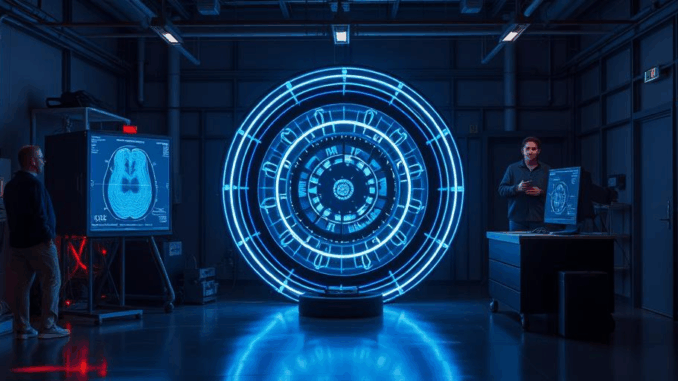
Neutron Activation Analysis: Principles, Applications, and Advancements in Biomedical Imaging and Beyond
Abstract
Neutron Activation Analysis (NAA) is a highly sensitive and versatile nuclear technique with applications spanning diverse fields, including materials science, environmental monitoring, archaeology, and biomedicine. This report provides a comprehensive overview of NAA, delving into its fundamental principles, instrumentation, and analytical capabilities. It explores the underlying nuclear physics, the types of isotopes produced during neutron irradiation, and the factors influencing the technique’s sensitivity and accuracy. Moreover, the report investigates the current landscape of NAA applications, focusing on its role in advanced imaging, particularly in tracking gold nanoparticles (AuNPs) within biological systems. A critical comparison of NAA with other established imaging modalities such as MRI, CT, and PET is presented, evaluating their respective strengths and weaknesses in terms of sensitivity, spatial resolution, invasiveness, cost, and suitability for specific applications. The report also addresses the potential benefits and risks associated with NAA, as well as the regulatory considerations concerning the use of radioactive isotopes in medical and research settings. Finally, the report highlights the latest advancements and future directions in NAA, exploring potential improvements in instrumentation, data analysis techniques, and novel applications that could further enhance its impact across various disciplines.
1. Introduction
Neutron Activation Analysis (NAA) is a powerful analytical technique rooted in nuclear physics, enabling the quantitative determination of elemental composition in a wide range of materials. Unlike many other analytical methods that rely on chemical digestion or dissolution of the sample, NAA is essentially non-destructive, requiring minimal sample preparation and precluding the risk of contamination introduced during chemical processing. The method is based on the principle of inducing radioactivity in the sample by bombarding it with neutrons, followed by the identification and quantification of the resulting radioactive isotopes through their characteristic gamma-ray emissions. This process allows for the detection of trace elements at extremely low concentrations, often in the parts-per-billion (ppb) or even parts-per-trillion (ppt) range.
The versatility and sensitivity of NAA have led to its widespread use in diverse fields. In materials science, NAA is employed to characterize the elemental composition of alloys, semiconductors, and ceramics, providing crucial insights into their properties and performance. Environmental scientists utilize NAA to monitor pollutants in air, water, and soil, contributing to environmental protection and risk assessment. Archaeologists leverage NAA to determine the provenance of artifacts, tracing their origins and shedding light on ancient trade routes and cultural interactions. In the biomedical field, NAA has emerged as a valuable tool for elemental analysis of biological tissues and fluids, offering insights into nutrient metabolism, toxicological effects, and disease mechanisms. More recently, NAA has gained prominence as an advanced imaging technique for tracking nanomaterials, such as gold nanoparticles (AuNPs), within the body, providing valuable information for drug delivery research and nanomedicine.
This report aims to provide a comprehensive overview of NAA, covering its fundamental principles, instrumentation, applications, and recent advancements. We will delve into the underlying nuclear physics, the types of isotopes produced during neutron irradiation, and the factors influencing the technique’s sensitivity and accuracy. Furthermore, we will investigate the current landscape of NAA applications, focusing on its role in advanced imaging, particularly in tracking AuNPs within biological systems. A critical comparison of NAA with other established imaging modalities such as MRI, CT, and PET will be presented, evaluating their respective strengths and weaknesses in terms of sensitivity, spatial resolution, invasiveness, cost, and suitability for specific applications. The report will also address the potential benefits and risks associated with NAA, as well as the regulatory considerations concerning the use of radioactive isotopes in medical and research settings. Finally, we will highlight the latest advancements and future directions in NAA, exploring potential improvements in instrumentation, data analysis techniques, and novel applications that could further enhance its impact across various disciplines.
2. Principles of Neutron Activation Analysis
NAA is based on the nuclear reaction that occurs when a stable nucleus captures a neutron. This process results in the formation of an unstable, radioactive isotope of the same element, which subsequently decays, emitting characteristic gamma rays. The energy and intensity of these gamma rays are unique to each radioisotope, allowing for the identification and quantification of the original element present in the sample.
2.1. Neutron Sources
The neutron flux, defined as the number of neutrons passing through a unit area per unit time, is a critical parameter in NAA. Higher neutron fluxes lead to greater activation and, consequently, increased sensitivity. Common neutron sources include:
- Nuclear Reactors: Research reactors provide the highest neutron fluxes, typically in the range of 1012 to 1014 neutrons/cm2/s. Reactors offer both thermal and epithermal neutron irradiation capabilities, enabling selective activation of specific elements based on their neutron absorption cross-sections.
- Accelerator-Based Neutron Sources: These sources utilize charged particle beams (e.g., protons or deuterons) to bombard a target material (e.g., beryllium or lithium), producing neutrons through nuclear reactions such as (p,n) or (d,n). Accelerator-based sources offer flexibility in terms of neutron energy and flux, but typically provide lower fluxes compared to nuclear reactors.
- Radioisotope Neutron Sources: These sources utilize the spontaneous emission of neutrons from radioactive isotopes such as Californium-252 (252Cf). Radioisotope sources are compact and portable, making them suitable for field applications, but they offer the lowest neutron fluxes among the three types.
The choice of neutron source depends on the specific application, considering factors such as sensitivity requirements, sample size, availability of facilities, and cost.
2.2. Nuclear Reactions and Isotope Production
The most common nuclear reaction in NAA is the (n,γ) reaction, where a nucleus captures a neutron and emits one or more gamma rays. This process results in the formation of a radioactive isotope with a mass number one unit higher than the original nucleus. The probability of a neutron being captured by a nucleus is described by the neutron absorption cross-section, which is energy-dependent and varies significantly among different elements and isotopes. Isotopes with high neutron absorption cross-sections are more readily activated and can be detected at lower concentrations.
Other nuclear reactions, such as (n,p), (n,α), and (n,2n), can also occur during neutron irradiation, leading to the formation of different isotopes or elements. These reactions are typically less probable than the (n,γ) reaction but can be significant for certain elements and neutron energies. The selection of appropriate irradiation conditions (e.g., neutron energy spectrum, irradiation time) is crucial to optimize the activation of target elements while minimizing interference from other reactions.
2.3. Radioactive Decay and Gamma-Ray Spectrometry
The radioactive isotopes produced during neutron irradiation decay through various processes, such as beta decay, positron emission, or electron capture, accompanied by the emission of characteristic gamma rays. The energy of the gamma rays is unique to each radioisotope and provides a fingerprint for its identification. The intensity of the gamma rays is proportional to the concentration of the original element in the sample.
Gamma-ray spectrometry is used to measure the energy and intensity of the emitted gamma rays. Semiconductor detectors, such as high-purity germanium (HPGe) detectors, are commonly employed due to their high energy resolution and detection efficiency. The gamma-ray spectrum, which is a plot of gamma-ray intensity versus energy, contains a series of peaks corresponding to the characteristic gamma rays of the various radioisotopes present in the sample. By analyzing the energy and area of these peaks, the identity and concentration of the elements in the sample can be determined.
2.4. Quantification and Sensitivity
The concentration of an element in the sample is determined by comparing the measured gamma-ray intensity to that of a known standard irradiated under identical conditions. The activity, A, of a radioactive isotope is given by:
A = Nσφ(1 – exp(-λtirr))exp(-λ*tdecay)
where:
- N is the number of target nuclei.
- σ is the neutron absorption cross-section.
- φ is the neutron flux.
- λ is the decay constant.
- tirr is the irradiation time.
- tdecay is the decay time.
The sensitivity of NAA depends on several factors, including the neutron flux, the neutron absorption cross-section of the target element, the decay half-life of the resulting radioisotope, the detection efficiency of the gamma-ray spectrometer, and the background radiation levels. Elements with high neutron absorption cross-sections, relatively long half-lives, and strong gamma-ray emissions are typically detected with higher sensitivity.
3. Applications of Neutron Activation Analysis
NAA finds application in diverse fields, its non-destructive nature, high sensitivity, and capability for multi-elemental analysis making it suitable for a wide range of materials and sample types.
3.1. Environmental Monitoring
NAA is a valuable tool for environmental monitoring, enabling the determination of trace elements in air, water, soil, and biological samples. It can be used to assess the levels of pollutants such as heavy metals (e.g., mercury, cadmium, lead) and organic contaminants, providing crucial information for environmental risk assessment and regulatory compliance. NAA has been used to study the sources and transport pathways of pollutants, as well as to monitor the effectiveness of remediation efforts.
3.2. Materials Science
In materials science, NAA is employed to characterize the elemental composition of a wide range of materials, including metals, alloys, semiconductors, ceramics, and polymers. It can be used to determine the concentration of dopants and impurities in semiconductors, which can significantly affect their electrical properties. NAA is also used to study the composition of thin films and coatings, as well as to analyze the distribution of elements within materials.
3.3. Archaeology and Cultural Heritage
NAA is a powerful tool for archaeological research, enabling the determination of the provenance of artifacts and the reconstruction of ancient trade routes. By analyzing the elemental composition of ceramics, metals, and other materials, NAA can identify the source of raw materials and track the movement of goods across geographical regions. NAA has been used to study the origins of ancient pottery, the composition of metallic artifacts, and the authenticity of art objects. The non-destructive nature of NAA is particularly important in this field, as it allows for the analysis of valuable and fragile artifacts without causing damage.
3.4. Biomedical Applications
NAA has found increasing applications in the biomedical field, offering a unique approach for elemental analysis of biological tissues, fluids, and pharmaceuticals. It can be used to study the role of trace elements in human health and disease, as well as to assess the toxicity of drugs and environmental pollutants. NAA has been used to measure the concentration of essential elements such as iron, zinc, and selenium in blood, urine, and tissues, providing insights into nutrient metabolism and deficiency disorders. It has also been used to study the accumulation of toxic elements such as mercury, lead, and arsenic in the body, contributing to the understanding of their health effects.
3.4.1. AuNP Tracking
One particularly promising application of NAA in biomedicine is its use in tracking gold nanoparticles (AuNPs) within the body. AuNPs are increasingly being used as drug delivery vehicles, imaging agents, and therapeutic agents due to their unique physicochemical properties and biocompatibility. However, tracking the biodistribution and clearance of AuNPs in vivo is challenging. Conventional imaging techniques such as MRI and CT have limited sensitivity for detecting AuNPs at low concentrations. NAA offers a highly sensitive method for quantifying AuNPs in biological samples, allowing for detailed studies of their pharmacokinetics and biodistribution. The high neutron capture cross-section of gold makes it particularly well-suited for NAA. Although NAA is not itself an imaging modality, by taking samples of tissue in vivo and analysing them using NAA, it can produce a profile of AuNP density in organs of the body.
4. Comparison with Other Imaging Modalities
While NAA offers high sensitivity for elemental analysis, it is not a direct imaging technique like MRI, CT, PET, or SPECT. Each modality has its strengths and weaknesses, making it suitable for different applications. Here’s a comparative overview:
- Magnetic Resonance Imaging (MRI): MRI provides excellent soft tissue contrast and spatial resolution, making it suitable for anatomical and functional imaging. However, MRI has limited sensitivity for detecting trace elements and requires contrast agents for enhanced visualization. MRI is non-ionizing, making it a safe option for repeated imaging studies. The cost of MRI is relatively high, and the technique is not readily available in all settings.
- Computed Tomography (CT): CT provides high-resolution anatomical images, particularly of bone and dense tissues. CT can be used to detect AuNPs, but the sensitivity is limited, and high concentrations of AuNPs are required for visualization. CT involves ionizing radiation, which can pose a health risk with repeated exposures. The cost of CT is moderate, and the technique is widely available.
- Positron Emission Tomography (PET): PET provides highly sensitive functional and molecular imaging, allowing for the visualization of biological processes at the cellular and molecular level. PET requires the use of radioactive tracers, which can pose a health risk. PET has limited spatial resolution compared to MRI and CT. The cost of PET is high, and the technique is not readily available in all settings.
-
Single-Photon Emission Computed Tomography (SPECT): SPECT is similar to PET but uses different radioactive tracers that emit single photons instead of positrons. SPECT has lower sensitivity and spatial resolution than PET but is more readily available and less expensive. SPECT also involves ionizing radiation. Similar to NAA, it’s used in conjunction with other imaging techniques or to validate findings due to its unique strengths.
-
NAA: NAA excels in providing quantitative elemental analysis with exceptional sensitivity, especially for elements with high neutron capture cross-sections like gold. While not a direct imaging technique, NAA provides valuable data on the elemental composition of biological samples and can be used to validate and complement findings from other imaging modalities. NAA requires access to a nuclear reactor or accelerator-based neutron source, which can be a limiting factor. The use of radioactive isotopes necessitates strict safety protocols and regulatory compliance. The technique can be destructive to samples if high fluxes are used over extended periods.
| Feature | NAA | MRI | CT | PET | SPECT |
|——————-|————————————|————————————|————————————–|————————————–|————————————-|
| Sensitivity | High | Low (without contrast) | Moderate | High | Moderate |
| Spatial Resolution| N/A (Elemental Analysis) | High | High | Moderate | Low |
| Invasiveness | Moderate (Sample Collection) | Non-invasive | Minimally Invasive (Radiation Dose) | Minimally Invasive (Radiation Dose) | Minimally Invasive (Radiation Dose)|
| Cost | High (Equipment and Operation) | High | Moderate | High | Moderate |
| Radiation | Yes (Sample Becomes Radioactive) | No | Yes | Yes | Yes |
| Applicability | Elemental Quantification, AuNP Tracking | Soft Tissue Imaging, Functional Imaging | Bone Imaging, Dense Tissue Imaging | Molecular Imaging, Functional Imaging | Organ Imaging, Functional Imaging |
5. Risks, Benefits, and Regulatory Aspects
5.1. Risks
- Radiation Exposure: The use of NAA involves the generation of radioactive isotopes, which can pose a risk of radiation exposure to personnel and the environment. Strict safety protocols must be implemented to minimize radiation exposure, including the use of shielding, remote handling equipment, and radiation monitoring systems. Proper training of personnel is essential to ensure safe operation of the NAA facility.
- Sample Handling: The handling of radioactive samples requires careful attention to avoid contamination and exposure. Appropriate personal protective equipment (PPE) should be worn, and procedures for handling and disposing of radioactive waste must be followed.
- Sample Matrix Effects: The accuracy of NAA can be affected by sample matrix effects, which can alter the neutron flux distribution and gamma-ray attenuation. Careful consideration of sample preparation and standardization is necessary to minimize these effects.
5.2. Benefits
- High Sensitivity: NAA offers exceptional sensitivity for elemental analysis, allowing for the detection of trace elements at very low concentrations.
- Multi-elemental Analysis: NAA can simultaneously determine the concentration of multiple elements in a single sample.
- Non-destructive or Minimally Destructive: NAA is essentially non-destructive, preserving the integrity of the sample for further analysis or other purposes.
- Minimal Sample Preparation: NAA requires minimal sample preparation, reducing the risk of contamination and simplifying the analytical process.
5.3. Regulatory Aspects
The use of radioactive isotopes in NAA is subject to strict regulatory oversight by national and international agencies. These regulations govern the licensing of NAA facilities, the handling and disposal of radioactive materials, and the protection of workers and the public from radiation exposure. Compliance with these regulations is essential to ensure the safe and responsible use of NAA.
In the United States, the Nuclear Regulatory Commission (NRC) regulates the possession and use of radioactive materials. In Europe, the European Atomic Energy Community (EURATOM) sets standards for radiation protection and nuclear safety. Other countries have their own regulatory bodies responsible for overseeing the use of radioactive isotopes. The regulations vary depending on the type and quantity of radioactive material used, as well as the specific application.
6. Advancements and Future Directions
NAA continues to evolve with advancements in instrumentation, data analysis techniques, and new applications. Some key areas of development include:
- Improved Neutron Sources: The development of more efficient and compact neutron sources, such as accelerator-based sources and compact fusion neutron sources, could expand the accessibility of NAA and enable its deployment in a wider range of settings.
- Advanced Detector Systems: The development of new detector materials and detector geometries could improve the energy resolution and detection efficiency of gamma-ray spectrometers, enhancing the sensitivity and accuracy of NAA.
- Data Analysis and Modeling: The use of advanced data analysis techniques, such as machine learning and multivariate analysis, can improve the accuracy of elemental quantification and enable the identification of complex patterns in NAA data. Computational modeling can be used to simulate neutron transport and gamma-ray interactions, providing a better understanding of sample matrix effects and optimizing experimental parameters.
- Micro-NAA: The development of micro-NAA techniques, using focused neutron beams and micro-detectors, could enable the analysis of small sample volumes and the spatial mapping of elemental distributions within materials. This would open up new possibilities for studying the composition of individual cells, microstructures, and archaeological artifacts.
- In vivo NAA: The development of in vivo NAA techniques, using portable neutron sources and detectors, could enable the non-invasive measurement of elemental concentrations in living subjects. This would have significant implications for medical diagnosis, nutritional assessment, and environmental monitoring. This poses considerable safety challenges however it would circumvent the need for biopsies when tracking AuNPs in vivo for example.
7. Conclusion
Neutron Activation Analysis remains a powerful and versatile analytical technique with a wide range of applications in diverse fields. Its high sensitivity, multi-elemental capability, and non-destructive nature make it an invaluable tool for elemental analysis of materials, environmental samples, archaeological artifacts, and biological tissues. While NAA is not a direct imaging technique, it provides complementary information to other imaging modalities and can be used to validate and enhance their findings. The development of new neutron sources, detector systems, and data analysis techniques continues to improve the performance and expand the applications of NAA. As research in materials science, environmental science, archaeology, and biomedicine continues to advance, NAA will undoubtedly play an increasingly important role in addressing complex analytical challenges and driving scientific discovery.
References
- Alfassi, Z. B. (Ed.). (1994). Chemical analysis by nuclear methods. John Wiley & Sons.
- De Soete, D., Gijbels, R., & Hoste, J. (1972). Neutron activation analysis. Wiley-Interscience.
- Ehmann, W. D., & Vance, D. E. (1991). Radiochemistry and nuclear methods of analysis. John Wiley & Sons.
- IAEA. (2003). Neutron activation analysis: A reference manual for marine pollution studies. IAEA.
- Knoll, G. F. (2010). Radiation detection and measurement. John Wiley & Sons.
- Glascock, M. D. (2013). Neutron activation analysis. In Handbook of archaeological sciences (pp. 277-295). John Wiley & Sons.
- Biegalski, S. R., Riley, D. A., & Gardner, R. P. (2008). A review of the development and application of neutron activation analysis. Journal of Radioanalytical and Nuclear Chemistry, 278(1), 25-30.
- Landsberger, S., Simsons, A., & Dyer, K. (2012). Application of neutron activation analysis to environmental forensics. Journal of Radioanalytical and Nuclear Chemistry, 293(1), 15-22.
- Sansone, U., Bode, P., & Ridikas, D. (2015). Neutron activation analysis: Status, potential and perspectives. Analytica Chimica Acta, 898, 1-22.
- Glausius, R., Veres, D., & Tanner, K. (2020). Neutron activation analysis in cultural heritage research: A review. Journal of Archaeological Science: Reports, 34, 102648.
- Zucker, M. S. (1989). Source book for NAA neutron activation analysis. Springer Science & Business Media.
The high sensitivity of Neutron Activation Analysis for tracking gold nanoparticles opens intriguing possibilities. How might this technique contribute to the development of targeted drug delivery systems that can cross the blood-brain barrier, and what challenges need to be overcome?
That’s a great question! The high sensitivity of NAA could allow us to precisely quantify AuNP accumulation within specific brain regions, informing the design of drug carriers optimized for BBB penetration. Overcoming the challenge of sample radioactivity post-NAA is crucial for further biological assays. Perhaps combining NAA with microdialysis could provide real-time pharmacokinetic data!
Editor: MedTechNews.Uk
Thank you to our Sponsor Esdebe