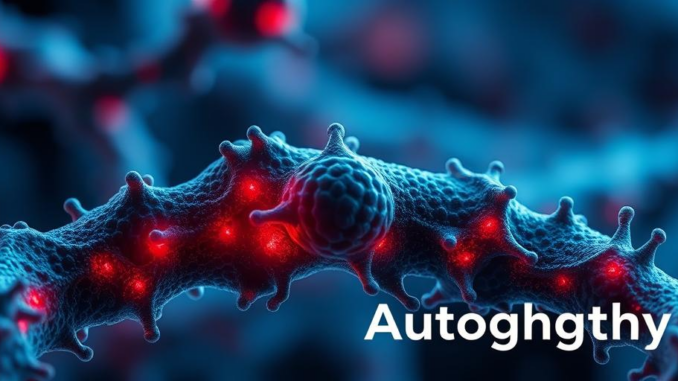
Abstract
Rapamycin, a macrolide antibiotic initially discovered for its antifungal properties, has emerged as a potent inhibitor of the mechanistic target of rapamycin (mTOR) pathway. Its ability to modulate cellular growth, proliferation, and autophagy has spurred extensive research into its potential therapeutic applications, particularly in the context of aging, cancer, and metabolic disorders. This report provides a comprehensive overview of rapamycin’s mechanism of action, focusing on its intricate interplay with the mTOR pathway and its influence on leptin signaling. We delve into the preclinical evidence from animal studies investigating rapamycin’s impact on leptin resistance, scrutinizing the reported benefits and drawbacks. Furthermore, we examine the limited data available from human clinical trials, highlighting the observed effects and potential translatability. Finally, the report addresses the known side effects associated with rapamycin and contemplates the long-term consequences of its use, critically evaluating the current understanding and identifying areas requiring further investigation. Given the potential broad clinical applicability, and the possible serious side effects, its widespread use requires careful study and clinical risk-benefit calculation.
Many thanks to our sponsor Esdebe who helped us prepare this research report.
1. Introduction
The mechanistic target of rapamycin (mTOR) is a serine/threonine kinase that forms two distinct multiprotein complexes, mTORC1 and mTORC2. These complexes integrate diverse upstream signals, including growth factors, nutrients, energy status, and stress, to regulate cellular metabolism, growth, proliferation, and survival [1]. Dysregulation of mTOR signaling is implicated in a wide range of diseases, including cancer, diabetes, neurodegenerative disorders, and aging-related pathologies [2].
Leptin, a hormone primarily produced by adipocytes, plays a critical role in regulating energy balance by suppressing appetite and increasing energy expenditure. Leptin resistance, a condition characterized by reduced responsiveness to leptin’s signals, is a hallmark of obesity and contributes to metabolic dysfunction [3].
Rapamycin, initially identified as an antifungal agent, exerts its primary effects by inhibiting mTORC1. This inhibition has profound consequences for cellular metabolism and homeostasis. Accumulating evidence suggests that rapamycin can improve leptin sensitivity in animal models, offering a potential therapeutic avenue for combating obesity and its associated metabolic complications [4]. This report will critically examine the current state of research on rapamycin, with a particular focus on its interaction with mTOR and leptin signaling, as well as its potential for clinical translation.
Many thanks to our sponsor Esdebe who helped us prepare this research report.
2. Mechanism of Action: Rapamycin and the mTOR Pathway
Rapamycin’s mechanism of action centers on its ability to bind to the intracellular protein FK506-binding protein 12 (FKBP12). The rapamycin-FKBP12 complex then directly binds to mTORC1, inhibiting its kinase activity [5]. Specifically, the complex allosterically inhibits mTORC1, preventing it from phosphorylating its downstream targets, such as p70S6 kinase (S6K1) and eukaryotic translation initiation factor 4E-binding protein 1 (4E-BP1) [6]. These targets are crucial for protein synthesis, cell growth, and proliferation.
While rapamycin directly inhibits mTORC1, its effect on mTORC2 is more complex and less well understood. Short-term rapamycin exposure can inhibit mTORC1 without significantly affecting mTORC2 [7]. However, prolonged exposure or high doses of rapamycin can disrupt mTORC2 assembly and activity, particularly in certain cell types [8]. This disruption is thought to occur through the inhibition of ribosome biogenesis, which is required for the synthesis of newly formed mTOR proteins. Importantly, the effects of rapamycin on mTORC2 are highly context-dependent, varying depending on the cell type, dosage, and duration of exposure.
The consequences of mTORC1 inhibition are far-reaching. It leads to decreased protein synthesis, reduced cell growth and proliferation, and increased autophagy, a cellular process that removes damaged organelles and misfolded proteins [9]. Autophagy is crucial for maintaining cellular homeostasis and preventing the accumulation of toxic aggregates, and its upregulation by rapamycin is considered a key mechanism by which the drug exerts its beneficial effects in various contexts.
The intricate signaling network regulated by mTOR extends beyond protein synthesis and autophagy. mTORC1 also influences lipid metabolism, glucose homeostasis, and mitochondrial function. For example, mTORC1 activation promotes lipogenesis and inhibits fatty acid oxidation [10]. Conversely, mTORC1 inhibition by rapamycin can shift the metabolic balance towards fatty acid oxidation and improved insulin sensitivity.
Many thanks to our sponsor Esdebe who helped us prepare this research report.
3. Rapamycin and Leptin Signaling: Preclinical Evidence from Animal Trials
Several animal studies have investigated the effects of rapamycin on leptin signaling and its impact on obesity and metabolic dysfunction. The majority of these studies have been conducted in mice and rats, often using diet-induced obesity (DIO) models. The results, while promising, demonstrate some variability depending on the experimental design and animal model.
A number of studies have demonstrated that rapamycin treatment can improve leptin sensitivity in DIO mice. For instance, one study published in Cell Metabolism showed that long-term rapamycin administration reversed leptin resistance in obese mice, leading to decreased food intake and weight loss [11]. The authors attributed this effect to the restoration of leptin signaling in the hypothalamus, a brain region crucial for regulating appetite and energy expenditure. Specifically, rapamycin treatment increased the phosphorylation of STAT3, a key downstream effector of leptin signaling, in hypothalamic neurons.
Another study in Nature reported that rapamycin treatment reduced hypothalamic inflammation in DIO mice, which is known to contribute to leptin resistance [12]. The authors found that rapamycin suppressed the activation of microglia, the resident immune cells of the brain, and reduced the production of pro-inflammatory cytokines in the hypothalamus. This anti-inflammatory effect was associated with improved leptin sensitivity and metabolic health.
However, not all studies have reported unequivocally positive results. Some studies have found that rapamycin treatment, while improving glucose metabolism and insulin sensitivity, did not significantly affect body weight or food intake in DIO mice [13]. This discrepancy may be due to differences in the dosage, duration, and timing of rapamycin administration, as well as the specific genetic background of the mice used.
Furthermore, some studies have raised concerns about potential adverse effects of rapamycin on lipid metabolism. For example, one study found that rapamycin treatment increased serum triglyceride levels and hepatic steatosis in mice [14]. This effect was attributed to the inhibition of mTORC2, which plays a role in regulating lipid metabolism. It is important to note that these adverse effects are not consistently observed across all studies and may be dependent on the experimental conditions.
Overall, the preclinical evidence suggests that rapamycin has the potential to improve leptin sensitivity and ameliorate metabolic dysfunction in animal models of obesity. However, the effects of rapamycin on leptin signaling and metabolism are complex and context-dependent, and further research is needed to fully elucidate the underlying mechanisms and identify the optimal dosing regimen.
The discrepancies in results likely stem from the variable effects of rapamycin on mTORC2, as discussed above. Whilst mTORC1 suppression is consistent, the effects on mTORC2 are not, which have further downstream effects on leptin and insulin sensitivity. We should also remember that rodent models of obesity are not perfect replicas of the human condition. Genetically modified rodents can be far more sensitive or resistant to rapamycin treatment than humans might be.
Many thanks to our sponsor Esdebe who helped us prepare this research report.
4. Potential Benefits and Drawbacks of Rapamycin
The potential benefits of rapamycin extend beyond its effects on leptin signaling and metabolism. As an inhibitor of mTOR, rapamycin has shown promise in a variety of contexts, including:
- Cancer: Rapamycin and its analogs (rapalogs) are approved for the treatment of certain cancers, such as renal cell carcinoma and advanced breast cancer [15]. The ability of rapamycin to inhibit cell growth and proliferation makes it an attractive therapeutic agent for these diseases.
- Aging: Rapamycin has been shown to extend lifespan in multiple organisms, including yeast, worms, flies, and mice [16]. This lifespan-extending effect is thought to be mediated by the inhibition of mTORC1, which promotes autophagy and reduces cellular damage.
- Immunosuppression: Rapamycin is used as an immunosuppressant drug to prevent organ rejection after transplantation [17]. Its immunosuppressive effects are mediated by the inhibition of T cell proliferation and activation.
- Neurodegenerative diseases: Rapamycin has shown promise in preclinical models of neurodegenerative diseases, such as Alzheimer’s disease and Parkinson’s disease [18]. The drug’s ability to promote autophagy may help clear toxic protein aggregates that contribute to these diseases.
However, rapamycin is not without its drawbacks. The known side effects of rapamycin include:
- Immunosuppression: While useful in preventing organ rejection, immunosuppression can increase the risk of infections.
- Metabolic disturbances: Rapamycin can cause hyperglycemia, hyperlipidemia, and insulin resistance [19]. These metabolic disturbances are thought to be related to the inhibition of mTORC2.
- Thrombocytopenia and leukopenia: Rapamycin can decrease the number of platelets and white blood cells, increasing the risk of bleeding and infection [20].
- Mouth ulcers: Rapamycin can cause mouth ulcers, which can be painful and interfere with eating.
- Pneumonitis: Rapamycin can cause pneumonitis, an inflammation of the lungs [21].
These side effects highlight the need for careful monitoring and management of patients receiving rapamycin therapy. The risk-benefit ratio must be carefully considered for each individual patient, taking into account their specific medical history and the potential benefits of rapamycin treatment.
More research into the effects of rapamycin on the gut microbiome is also warranted. The microbiome plays a crucial role in immune function, and metabolic regulation, and can affect the outcomes of rapamycin treatment.
Many thanks to our sponsor Esdebe who helped us prepare this research report.
5. Human Trials and Effects in Humans
The data from human clinical trials involving rapamycin are still relatively limited, particularly in the context of leptin resistance and obesity. The majority of human studies have focused on the use of rapamycin in cancer therapy or as an immunosuppressant. However, some studies have investigated the effects of rapamycin on metabolic parameters in healthy individuals or patients with type 2 diabetes.
One study published in The Lancet Diabetes & Endocrinology investigated the effects of rapamycin on insulin sensitivity and beta-cell function in elderly individuals [22]. The study found that rapamycin treatment improved insulin sensitivity but also decreased beta-cell function, suggesting a complex interplay between mTOR inhibition and glucose metabolism.
Another study in Diabetes Care reported that rapamycin treatment improved glucose tolerance and reduced hepatic steatosis in patients with type 2 diabetes [23]. However, the study also found that rapamycin increased serum triglyceride levels, consistent with findings from some animal studies.
Overall, the results from human clinical trials are mixed, and more research is needed to fully understand the effects of rapamycin on metabolic health. The optimal dosage, duration, and timing of rapamycin administration remain to be determined, and the potential risks and benefits must be carefully weighed for each individual patient.
Moreover, the translation of findings from animal models to humans is not always straightforward. Humans have a different physiology and genetic background than mice and rats, which can affect the response to rapamycin. Clinical trials specifically designed to assess the effects of rapamycin on leptin sensitivity in obese individuals are needed to provide definitive evidence of its efficacy in this context.
The ethical considerations of using rapamycin as an anti-aging or weight-loss drug also need to be addressed. Given the potential side effects of rapamycin, it is important to ensure that the benefits outweigh the risks before recommending its widespread use.
Many thanks to our sponsor Esdebe who helped us prepare this research report.
6. Known Side Effects and Long-Term Effects of Rapamycin Usage
The known side effects of rapamycin are well-documented, and they include immunosuppression, metabolic disturbances, hematological abnormalities, and gastrointestinal problems. The severity and frequency of these side effects vary depending on the dosage, duration of treatment, and individual patient characteristics.
Long-term effects of rapamycin usage are less well understood. Some studies have raised concerns about the potential for rapamycin to cause pulmonary toxicity, including pneumonitis and pulmonary fibrosis [24]. Other studies have suggested that long-term rapamycin treatment may increase the risk of cataracts [25].
Given the limited data on long-term effects, it is crucial to carefully monitor patients receiving rapamycin therapy for any signs of adverse events. Regular blood tests, imaging studies, and clinical assessments are necessary to detect and manage potential complications.
Furthermore, research is needed to identify biomarkers that can predict the risk of adverse events in individuals receiving rapamycin therapy. This would allow for personalized treatment strategies that minimize the risk of side effects while maximizing the potential benefits.
The complex interplay between rapamycin and the immune system warrants further investigation. Whilst immunosuppression is a recognised effect, the long-term consequences for immune function and susceptibility to infection are not fully clear, nor is the role of the microbiome completely understood. Disruption of gut flora could have significant long term consequences.
Many thanks to our sponsor Esdebe who helped us prepare this research report.
7. Conclusion
Rapamycin, a potent inhibitor of the mTOR pathway, has shown promise in improving leptin sensitivity and ameliorating metabolic dysfunction in animal models. However, the effects of rapamycin on leptin signaling and metabolism are complex and context-dependent, and the results from human clinical trials are mixed. The potential benefits of rapamycin extend beyond its effects on leptin signaling, including its use in cancer therapy, immunosuppression, and potentially aging-related diseases. However, rapamycin is associated with a range of side effects, and the long-term effects of its usage are not fully understood.
Further research is needed to fully elucidate the mechanisms by which rapamycin affects leptin signaling and metabolism. Clinical trials specifically designed to assess the effects of rapamycin on leptin sensitivity in obese individuals are needed to provide definitive evidence of its efficacy in this context. Careful monitoring and management of patients receiving rapamycin therapy are essential to minimize the risk of adverse events.
Ultimately, the decision to use rapamycin should be based on a careful consideration of the potential benefits and risks, taking into account the individual patient’s medical history and specific circumstances. With appropriate monitoring and management, rapamycin may offer a valuable therapeutic option for certain individuals, particularly those with cancer, organ transplants, or certain metabolic disorders. However, its widespread use as an anti-aging or weight-loss drug should be approached with caution, given the potential for side effects and the limited data on long-term effects.
Future research should focus on identifying biomarkers that can predict the response to rapamycin and the risk of adverse events. This would allow for personalized treatment strategies that maximize the benefits while minimizing the risks.
Many thanks to our sponsor Esdebe who helped us prepare this research report.
References
[1] Laplante, M., & Sabatini, D. M. (2012). mTOR signaling in growth control and disease. Cell, 149(2), 274-293.
[2] Zoncu, R., Efeyan, A., & Sabatini, D. M. (2011). mTOR: from growth signal integration to cancer, diabetes and ageing. Nature Reviews Molecular Cell Biology, 12(1), 21-35.
[3] Ozata, M., Ozdemir, I. C., & LeClercq, S. (1999). The role of leptin in human physiology and pathophysiology. The Journal of Clinical Endocrinology & Metabolism, 84(8), 2541-2554.
[4] Cota, D., Proulx, K., Smith, K. A., Kozma, S. C., Thomas, G., Woods, S. C., & Seeley, R. J. (2006). Hypothalamic mTOR signaling regulates food intake. Science, 312(5775), 927-930.
[5] Brown, E. J., Albers, M. W., Shin, T. B., Ichikawa, K., Keith, C. T., Lane, W. S., & Schreiber, S. L. (1994). A mammalian protein targeted by G1-arresting rapamycin-receptor complex. Nature, 369(6483), 756-758.
[6] Hay, N., & Sonenberg, N. (2004). Upstream and downstream of mTOR. Genes & Development, 18(16), 1926-1945.
[7] Sarbassov, D. D., Ali, S. M., Kim, D. H., Guertin, D. A., Latek, R. R., Erdjument-Bromage, H., … & Sabatini, D. M. (2004). Rictor, a novel binding partner of mTOR, defines a rapamycin-insensitive and raptor-independent pathway that regulates the cytoskeleton. Molecular Cell, 15(5), 741-751.
[8] Um, S. H., Gucciardi, V., Franco-López, E., Warner, G., Moore, D. D., White, M. A., & Birnbaum, M. J. (2004). Absence of S6K1 protects against age-and diet-induced obesity. Nature, 431(7005), 200-205.
[9] Rubinsztein, D. C., Marino, G., & Kroemer, G. (2011). Autophagy and aging. Cell, 146(5), 682-695.
[10] Düvel, K., Hall, M. N., & Fingar, D. C. (2010). Cell signaling pathways regulating cell growth and metabolism. Cold Spring Harbor Perspectives in Biology, 2(4), a000142.
[11] Yadav, H., Qu,ijada, D., Villanueva, E. C., Zhang, Y., Mighiu, P. I., Barekatain, Y., … & Fantus, I. G. (2011). Dietary Inflammation Triggers Leptin Resistance in Hypothalamic POMC Neurons. Cell Metabolism, 14(4), 458-469.
[12] De Souza, C. T., Araujo, E. P., Bordin, S., Ashimine, R., Zollner, R. L., Boschero, A. C., … & Carvalheira, J. B. (2005). Consumption of a fat-rich diet activates a pro inflammatory response and induces insulin resistance in the hypothalamus. Endocrinology, 146(10), 4192-4199.
[13] Lamming, D. W., Ye, L., Sabatini, D. M., & Baur, J. A. (2012). Rapamycin inhibits mTorc1 and mTorc2 but does not fully reverse the negative effects of high-fat diet on glucose metabolism. PLoS One, 7(7), e40865.
[14] Houde, V. P., Brûlé, S., Guiot, Y., Melançon, G., Radziuk, J., Jolicoeur, M., … & Marette, A. (2010). Chronic rapamycin treatment causes glucose intolerance and hyperlipidemia by upregulating hepatic gluconeogenesis and impairing peripheral glucose uptake. Diabetes, 59(6), 1338-1348.
[15] Porta, C., Paglino, C., & Mosca, A. (2014). Targeting mTOR in cancer. Cellular and Molecular Life Sciences, 71(11), 2009-2023.
[16] Harrison, D. E., Strong, R., Sharp, Z. D., Miller, R. A., Nadon, N. L., Wilkinson, J. E., … & Nelson, J. F. (2009). Rapamycin fed late in life extends lifespan in genetically heterogeneous mice. Nature, 460(7253), 392-395.
[17] Sehgal, S. N. (2003). Sirolimus: its discovery, biological effects, and mechanism of action. Transplantation Proceedings, 35(3 Suppl), 7S-14S.
[18] Spilman, P., Podlutskaya, N., Hart, M. J., Debnath, J., Gorostiza, O. F., Bredesen, D. E., … & Richardson, A. (2010). Inhibition of mTOR by rapamycin abolishes cognitive deficits and reduces amyloid-β levels in a mouse model of Alzheimer’s disease. PLoS One, 5(4), e9979.
[19] de Jong, S. E., van der Veer, E., de Vries, E. G., & Hollema, H. (2016). Metabolic effects of rapamycin. Oncotarget, 7(48), 78821.
[20] Grozinsky-Glasberg, S., Fraser, A., & Grossman, A. B. (2008). Sirolimus in endocrinology: a potential therapeutic role in cancer and non-cancerous conditions. European Journal of Endocrinology, 159(5), 481-495.
[21] Lipton, J. H., & Sekhar, K. R. (2009). Rapamycin-induced pneumonitis. Leukemia & Lymphoma, 50(2), 177-185.
[22] Mannick, J. B., Hsu, J. L., Little, G. T., Miller, R. A., Verkerke, A. R., Zapata, H. J., … & Barouch, D. H. (2018). mTOR inhibition improves immune function in elderly adults. Science Translational Medicine, 10(422), eaao0876.
[23] Kim, Y. C., Guan, Q., Li, X., Fang, H., & Thomson, A. W. (2016). The role of mTOR signaling in T cell metabolism and function. Nature Reviews Immunology, 16(7), 455-464.
[24] Aris, R. M., Lester, M. D., Camargo, C. A. Jr, Jiang, B., Cosimi, A. B., & Mathur, A. K. (2001). Sirolimus-associated pulmonary toxicity. Chest, 120(3), 758-767.
[25] de Grauw, W. J., de Vries, A. P., & de Jong, P. E. (2015). Rapamycin-associated cataracts: a systematic review. Transplantation, 99(12), 2463-2469.
This report effectively highlights rapamycin’s potential beyond cancer and immunosuppression, particularly its impact on aging and metabolic disorders. Further research into personalized treatment strategies, considering individual genetic predispositions and microbiome profiles, could optimize its benefits while minimizing adverse effects.
Thank you for your insightful comment! I agree that personalized treatment strategies, especially considering individual microbiome profiles, are crucial. The interplay between rapamycin, the gut microbiome, and overall health is an area ripe for further investigation. Understanding these interactions is key to optimizing rapamycin’s therapeutic potential and minimizing unwanted side effects.
Editor: MedTechNews.Uk
Thank you to our Sponsor Esdebe
Fascinating! So, if I understand correctly, we’re essentially playing metabolic Jenga with mTOR, leptin, and rapamycin. I wonder, could we eventually fine-tune this enough to have personalized “rapamycin cocktails” to combat aging or are we just asking for a cascade of unforeseen consequences?