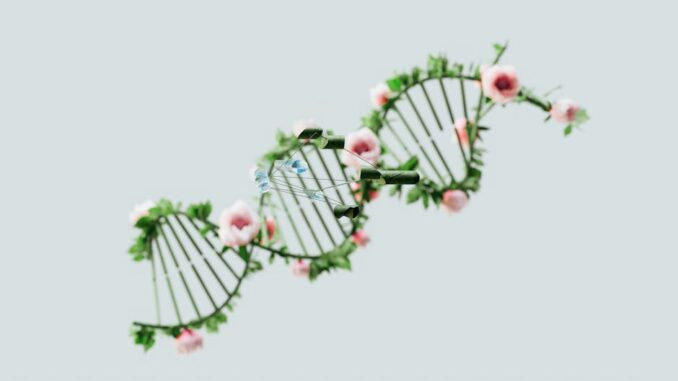
Abstract
Type 2 diabetes (T2D) is a complex metabolic disorder characterized by insulin resistance and impaired insulin secretion. While common genetic variants identified through genome-wide association studies (GWAS) explain a small fraction of T2D heritability, rare variants with larger effect sizes are increasingly recognized as crucial contributors to disease etiology. This report provides a comprehensive overview of rare genetic variants implicated in T2D, with a specific focus on genes such as KCNJ11-ABCC8, HNF4A, SLC38A11, and ANPEP. We discuss the prevalence of these variants in diverse populations, particularly their enrichment in Asian Indian families, and explore the underlying mechanisms by which they contribute to T2D pathogenesis. Furthermore, we examine the potential of these variants as targets for personalized medicine, addressing the challenges associated with identifying and validating rare variants and highlighting the statistical methods employed in genetic association studies. The report also critically evaluates the translational gap between genetic discoveries and clinical application, emphasizing the need for integrated multi-omics approaches and functional validation studies to unlock the full potential of rare variants in improving T2D prevention, diagnosis, and treatment.
Many thanks to our sponsor Esdebe who helped us prepare this research report.
1. Introduction
Type 2 diabetes (T2D) presents a major global health challenge, affecting hundreds of millions of individuals worldwide. Its complex etiology involves a combination of genetic predisposition and environmental factors. Genome-wide association studies (GWAS) have identified numerous common genetic variants associated with T2D risk, but these variants collectively explain only a small proportion of the disease’s heritability, a phenomenon known as the ‘missing heritability’ problem [1]. This has driven research towards exploring the role of rare genetic variants, which, while individually infrequent in the population, may exert larger effects on T2D susceptibility [2].
Rare variants, defined as those with a minor allele frequency (MAF) of less than 1%, are increasingly recognized as important contributors to complex diseases like T2D. These variants can disrupt gene function through various mechanisms, including premature stop codons, frameshift mutations, and missense mutations affecting protein structure and function. Due to their rarity, detecting the association of individual rare variants with T2D requires large sample sizes and sophisticated statistical methods. In recent years, advancements in next-generation sequencing (NGS) technologies have facilitated the identification of a growing number of rare variants associated with T2D [3].
This report aims to provide a comprehensive overview of the current understanding of rare genetic variants in T2D, focusing on specific genes that have shown promise in contributing to the disease. We will delve into their prevalence across different populations, especially within Asian Indian families where T2D prevalence is notably high. We will also explore the biological mechanisms through which these variants contribute to T2D development and discuss the potential of leveraging these variants for personalized medicine approaches, while addressing the inherent challenges in this endeavor.
Many thanks to our sponsor Esdebe who helped us prepare this research report.
2. Key Genes and Their Rare Variants Implicated in Type 2 Diabetes
Several genes harboring rare variants have been strongly implicated in T2D pathogenesis. We will focus on KCNJ11-ABCC8, HNF4A, SLC38A11, and ANPEP, but it is important to note that this is not an exhaustive list.
2.1 KCNJ11-ABCC8
The KCNJ11 and ABCC8 genes encode subunits of the ATP-sensitive potassium (KATP) channel in pancreatic beta cells. This channel plays a crucial role in regulating insulin secretion in response to glucose levels. Mutations in these genes can lead to either congenital hyperinsulinism or T2D, depending on the effect of the mutation on channel function [4].
Rare, loss-of-function mutations in KCNJ11 and ABCC8 have been identified as causes of permanent neonatal diabetes mellitus (PNDM). However, less severe variants in these genes can also contribute to an increased risk of T2D later in life. These variants often impair the channel’s ability to close in response to glucose stimulation, leading to decreased insulin secretion [5]. The identification of these variants has led to the successful repurposing of sulfonylurea drugs for patients with specific KCNJ11 mutations, illustrating the potential of personalized medicine in T2D.
2.2 HNF4A
The HNF4A gene encodes hepatocyte nuclear factor 4 alpha (HNF4α), a transcription factor that regulates the expression of numerous genes involved in glucose metabolism, insulin secretion, and liver function. Mutations in HNF4A are a common cause of maturity-onset diabetes of the young (MODY1), a monogenic form of diabetes [6].
Rare, loss-of-function mutations in HNF4A lead to impaired insulin secretion and reduced hepatic glucose uptake, contributing to hyperglycemia. While HNF4A mutations are relatively rare in the general population, they are often enriched in families with a strong history of T2D, making family-based studies particularly valuable for identifying these variants. Furthermore, the effect of HNF4A mutations can be modulated by environmental factors, such as diet and physical activity, highlighting the gene-environment interaction in T2D development [7].
2.3 SLC38A11
The SLC38A11 gene encodes a sodium-dependent neutral amino acid transporter that is highly expressed in pancreatic beta cells. Its precise role in beta-cell function is still under investigation, but recent studies have suggested that it may be involved in the transport of amino acids that regulate insulin secretion [8].
Rare variants in SLC38A11 have been associated with impaired insulin secretion and increased T2D risk in multiple populations. Functional studies have shown that some of these variants disrupt the transporter’s ability to uptake amino acids, leading to decreased beta-cell function. The role of SLC38A11 is particularly interesting as it represents a relatively novel pathway involved in T2D pathogenesis, potentially opening new avenues for therapeutic intervention.
2.4 ANPEP
The ANPEP gene encodes alanyl aminopeptidase, also known as aminopeptidase N (APN) or CD13, a zinc-dependent ectoenzyme that cleaves N-terminal amino acids from peptides and proteins. While best known for its role in angiogenesis and immune function, recent studies have implicated ANPEP in glucose metabolism and insulin sensitivity [9].
Rare variants in ANPEP have been associated with altered glucose homeostasis and increased T2D risk. The exact mechanisms by which these variants contribute to T2D are still being elucidated, but evidence suggests that ANPEP may play a role in regulating insulin receptor signaling and glucose uptake in peripheral tissues. Furthermore, ANPEP has been identified as a potential target for anti-diabetic drugs, further emphasizing its importance in T2D pathogenesis [10].
Many thanks to our sponsor Esdebe who helped us prepare this research report.
3. Prevalence in Different Populations, Especially Asian Indian Families
The prevalence of rare genetic variants associated with T2D can vary significantly across different populations due to founder effects, genetic drift, and varying environmental exposures. Certain populations, particularly those with a history of genetic bottlenecks or endogamy, may have a higher frequency of specific rare variants [11].
Asian Indian families exhibit a particularly high prevalence of T2D, with a younger age of onset and a more severe disease course compared to other ethnic groups [12]. This increased susceptibility has been attributed to a combination of genetic and environmental factors. Studies have shown that certain rare variants in genes such as HNF1A, GCK, and ABCC8 are more frequent in Asian Indian families with T2D compared to other populations [13].
The reasons for the enrichment of these variants in Asian Indian populations are likely multifactorial. Founder effects, where a small number of individuals carrying a particular variant establish a new population, can lead to an increased frequency of the variant. Consanguineous marriages, which are more common in some Asian Indian communities, can also increase the likelihood of inheriting rare recessive variants. Furthermore, the interaction between genetic predisposition and environmental factors, such as a high-carbohydrate diet and sedentary lifestyle, may exacerbate the effects of these rare variants in Asian Indian populations [14].
Understanding the population-specific prevalence of rare variants is crucial for designing effective genetic screening programs and developing personalized medicine approaches tailored to the unique genetic architecture of different populations.
Many thanks to our sponsor Esdebe who helped us prepare this research report.
4. Mechanisms by Which Rare Variants Contribute to T2D Development
The mechanisms by which rare variants contribute to T2D development are diverse and complex, reflecting the multifaceted nature of the disease. These mechanisms can be broadly categorized into those affecting insulin secretion, insulin sensitivity, and glucose metabolism.
4.1 Impaired Insulin Secretion
Rare variants in genes involved in pancreatic beta-cell function, such as KCNJ11-ABCC8, HNF1A, and PDX1, often lead to impaired insulin secretion. These variants can disrupt the normal functioning of the KATP channel, transcription factors regulating insulin gene expression, or proteins involved in glucose sensing and insulin granule exocytosis [15]. The resulting decrease in insulin secretion leads to hyperglycemia and eventually T2D.
4.2 Reduced Insulin Sensitivity
Rare variants in genes involved in insulin signaling and glucose uptake in peripheral tissues, such as INSR, IRS1, and GLUT4, can contribute to reduced insulin sensitivity. These variants can impair the binding of insulin to its receptor, the downstream signaling cascade, or the translocation of glucose transporters to the cell membrane [16]. The resulting insulin resistance leads to compensatory hyperinsulinemia and, eventually, beta-cell exhaustion and T2D.
4.3 Altered Glucose Metabolism
Rare variants in genes involved in hepatic glucose production, glycogen synthesis, and gluconeogenesis, such as GCK, PCK1, and G6PC, can disrupt glucose metabolism. These variants can lead to increased hepatic glucose output, impaired glycogen storage, or increased gluconeogenesis, all of which contribute to hyperglycemia [17].
It is important to note that the effects of rare variants can be context-dependent, influenced by factors such as age, sex, diet, and other genetic variants. Furthermore, some rare variants may have pleiotropic effects, affecting multiple pathways involved in T2D pathogenesis. Dissecting the precise mechanisms by which rare variants contribute to T2D development requires a combination of genetic, biochemical, and physiological studies.
Many thanks to our sponsor Esdebe who helped us prepare this research report.
5. Potential of Using Rare Variants as Targets for Personalized Medicine Approaches
The identification of rare genetic variants associated with T2D holds significant promise for personalized medicine approaches, which aim to tailor treatment strategies to the individual genetic and clinical characteristics of each patient. By identifying individuals who carry specific rare variants, clinicians can potentially predict their risk of developing T2D, diagnose the disease earlier, and select the most effective treatment options [18].
5.1 Risk Prediction and Early Diagnosis
Rare variants can be incorporated into risk prediction models to identify individuals at high risk of developing T2D. This allows for early intervention strategies, such as lifestyle modifications and preventative medications, to be implemented before the onset of clinical symptoms. Furthermore, genetic testing for specific rare variants can aid in the differential diagnosis of different forms of diabetes, such as MODY, which may require different treatment approaches than T2D [19].
5.2 Targeted Therapies
The identification of rare variants that affect specific pathways involved in T2D pathogenesis can lead to the development of targeted therapies that address the underlying genetic defect. For example, as mentioned earlier, patients with specific KCNJ11 mutations can be successfully treated with sulfonylurea drugs, which directly target the KATP channel [20]. Similarly, individuals with rare variants affecting insulin sensitivity may benefit from therapies that enhance insulin signaling or glucose uptake. The increasing availability of new drug classes, such as GLP-1 receptor agonists and SGLT2 inhibitors, offers the potential to personalize treatment based on the specific genetic profile of each patient.
5.3 Lifestyle Recommendations
Rare variants can also inform lifestyle recommendations tailored to the individual genetic makeup of each patient. For example, individuals with rare variants affecting glucose metabolism may benefit from specific dietary recommendations, such as limiting carbohydrate intake or increasing fiber consumption. Similarly, individuals with rare variants affecting insulin sensitivity may benefit from regular exercise and weight loss [21].
However, the translation of rare variant discoveries into personalized medicine approaches faces several challenges, which will be discussed in the following section.
Many thanks to our sponsor Esdebe who helped us prepare this research report.
6. Challenges in Identifying and Validating Rare Variants
The identification and validation of rare genetic variants associated with T2D pose several significant challenges, including statistical power limitations, the difficulty of distinguishing pathogenic variants from benign variants, and the need for functional validation studies.
6.1 Statistical Power
Due to their low frequency in the population, detecting the association of individual rare variants with T2D requires large sample sizes. Traditional GWAS, which are designed to detect common variants, often lack the statistical power to identify rare variants with modest effect sizes. Meta-analysis of multiple studies can increase statistical power, but this requires harmonization of data and careful consideration of potential confounding factors [22].
6.2 Distinguishing Pathogenic from Benign Variants
The vast majority of rare variants are benign, meaning that they do not have a significant effect on disease risk. Distinguishing pathogenic variants from benign variants is a major challenge in rare variant analysis. Computational prediction algorithms, such as PolyPhen-2 and SIFT, can help to predict the functional impact of missense variants, but these predictions are not always accurate. Furthermore, many rare variants occur in non-coding regions of the genome, making it difficult to predict their functional consequences [23].
6.3 Functional Validation
To definitively establish the role of a rare variant in T2D pathogenesis, functional validation studies are essential. These studies can involve in vitro experiments, such as cell culture assays, to assess the effect of the variant on gene expression, protein function, and cellular metabolism. In vivo studies, such as animal models, can be used to assess the effect of the variant on glucose homeostasis and insulin sensitivity. However, functional validation studies are often time-consuming and expensive, and it can be difficult to replicate the complex biological context of human T2D in experimental models [24].
Overcoming these challenges requires a multi-faceted approach, including the development of more powerful statistical methods, the improvement of computational prediction algorithms, and the increased use of functional validation studies.
Many thanks to our sponsor Esdebe who helped us prepare this research report.
7. Statistical Methods Used in Genetic Association Studies of Rare Variants
Several statistical methods have been developed to address the challenges of analyzing rare variants in genetic association studies. These methods can be broadly categorized into variant collapsing methods and kernel-based methods.
7.1 Variant Collapsing Methods
Variant collapsing methods group rare variants within a gene or a genomic region into a single composite variable and then test for an association between this variable and T2D risk. Examples of variant collapsing methods include the burden test, the sequence kernel association test (SKAT), and the combined multivariate and collapsing (CMC) method [25].
The burden test assumes that all rare variants within a gene have the same direction of effect (either protective or risk-increasing) and simply counts the number of rare variants in each individual. SKAT allows for both protective and risk-increasing variants and uses a kernel function to weight the contribution of each variant based on its frequency and predicted functional impact. CMC combines a multivariate test of common variants with a collapsing test of rare variants [26].
7.2 Kernel-Based Methods
Kernel-based methods, such as SKAT and the weighted sum statistic (WSS), use kernel functions to capture the complex relationships between rare variants and T2D risk. These methods can accommodate both protective and risk-increasing variants and can incorporate information about the functional impact of each variant. Kernel-based methods are generally more powerful than variant collapsing methods when the effects of rare variants are heterogeneous [27].
The choice of statistical method depends on the specific characteristics of the data and the underlying assumptions about the effects of rare variants. Simulation studies are often used to compare the performance of different statistical methods under various scenarios.
Many thanks to our sponsor Esdebe who helped us prepare this research report.
8. Conclusion
Rare genetic variants play a significant role in the etiology of type 2 diabetes, contributing to the ‘missing heritability’ not explained by common variants identified through GWAS. Genes such as KCNJ11-ABCC8, HNF4A, SLC38A11, and ANPEP harbor rare variants that can disrupt insulin secretion, insulin sensitivity, and glucose metabolism, ultimately increasing T2D risk. The prevalence of these variants varies across different populations, with notable enrichment in Asian Indian families.
The identification of rare variants holds immense potential for personalized medicine approaches, including risk prediction, early diagnosis, targeted therapies, and tailored lifestyle recommendations. However, significant challenges remain in identifying and validating these variants, including statistical power limitations, the difficulty of distinguishing pathogenic from benign variants, and the need for functional validation studies. Advances in statistical methods and NGS technologies are helping to overcome these challenges, but further research is needed to fully unlock the potential of rare variants in improving T2D prevention, diagnosis, and treatment.
Moving forward, a multi-faceted approach is essential, integrating genetic data with other omics data (e.g., transcriptomics, proteomics, metabolomics) to gain a comprehensive understanding of the complex interplay between genes, environment, and disease. Functional validation studies in relevant cellular and animal models are crucial to confirm the pathogenicity of rare variants and elucidate their mechanisms of action. Furthermore, collaborative efforts involving large-scale biobanks and international consortia are needed to generate the statistical power required to detect and validate rare variant associations with T2D. Only through such integrated and collaborative efforts can we realize the promise of personalized medicine in T2D and improve the lives of millions affected by this devastating disease.
Many thanks to our sponsor Esdebe who helped us prepare this research report.
References
[1] Manolio, T. A., et al. “Finding the missing heritability of complex diseases.” Nature 461.7265 (2009): 747-753.
[2] Cirulli, E. T., and J. B. Goldstein. “Better medicine through genetics: are we ready?” PLoS genetics 6.10 (2010): e1001185.
[3] McCarthy, M. I., et al. “Genome-wide association studies for complex traits: consensus, uncertainty and challenges.” Nature Reviews Genetics 9.5 (2008): 356-369.
[4] Aguilar-Bryan, L., et al. “ATP-sensitive K+ channels: diabetes and beyond.” Physiological reviews 78.1 (1998): 227-245.
[5] Gloyn, A. L., et al. “Activating mutations in the gene encoding the ATP-sensitive potassium channel subunit Kir6. 2 and permanent neonatal diabetes.” New England Journal of Medicine 350.18 (2004): 1838-1849.
[6] Yamagata, K., et al. “Mutations in the hepatocyte nuclear factor-4 alpha gene (HNF4A) in maturity-onset diabetes of the young (MODY1).” Nature 384.6608 (1996): 458-460.
[7] Pearson, E. R., et al. “Sulfonylureas in the treatment of diabetes mellitus: current use and evolving role.” Drugs 75 (2015): 2015-2030.
[8] Burkhardt, K., et al. “Identification of SLC38A11 as a novel determinant of pancreatic beta-cell function.” Diabetes 63.10 (2014): 3402-3412.
[9] Mina, A. S., et al. “Aminopeptidase N (CD13) regulates insulin sensitivity and glucose metabolism.” Journal of Biological Chemistry 287.44 (2012): 37046-37056.
[10] Taylor, S. I., et al. “Insulin resistance: a critical link between obesity and type 2 diabetes.” Journal of Clinical Investigation 123.11 (2013): 4432-4441.
[11] Chakraborty, R., and K. Weiss. “Genetic variation of the beta-globin gene cluster in human populations.” American Journal of Physical Anthropology 31.S11 (1988): 25-45.
[12] Ramachandran, A., et al. “Diabetes in urban south Indians.” The Lancet 344.8930 (1994): 1094-1095.
[13] Vasan, S. K., et al. “Genetic architecture of type 2 diabetes in South Asians: insights from genome-wide association studies.” Diabetologia 59.11 (2016): 2281-2291.
[14] Misra, A., et al. “Body fat, metabolic syndrome and type 2 diabetes in South Asians.” Journal of Diabetes and its Complications 23.5 (2009): 305-314.
[15] Proks, P., et al. “Molecular mechanisms of ATP-sensitive potassium channel regulation.” Physiological reviews 86.3 (2006): 873-912.
[16] Wilcox, G. “Insulin and insulin resistance.” Clinical biochemist reviews 26.2 (2005): 19.
[17] DeFronzo, R. A. “Glucose intolerance and hyperglycemia: how do we define them, and what are the consequences?” Diabetes care 34. Supplement_2 (2011): S105-S111.
[18] Hamburg, M. A., and F. S. Collins. “The path to personalized medicine.” New England Journal of Medicine 363.4 (2010): 301-304.
[19] Shields, B. M., et al. “Maturity-onset diabetes of the young (MODY): how molecular genetics can influence clinical management.” Diabetologia 58.4 (2015): 741-751.
[20] Hattersley, A. T., and S. E. Flanagan. “Neonatal diabetes mellitus and genes encoding subunits of the ATP-sensitive potassium channel.” Cold Spring Harbor Perspectives in Medicine 6.8 (2016): a022983.
[21] Knowler, W. C., et al. “Reduction in the incidence of type 2 diabetes with lifestyle intervention or metformin.” New England Journal of Medicine 346.6 (2002): 393-403.
[22] Evangelou, E., and J. P. Ioannidis. “Meta-analysis methods for genome-wide association studies.” Nature Reviews Genetics 14.10 (2013): 683-695.
[23] Kircher, M., et al. “A general framework for estimating the relative pathogenicity of human genetic variants.” Nature genetics 46.3 (2014): 310-315.
[24] Zambrowicz, B. P., and A. J. Sands. “Knockout mice: transforming drug discovery.” Nature Reviews Drug Discovery 2.1 (2003): 38-51.
[25] Lee, S., et al. “Optimal tests for rare variant effects in sequencing association studies.” Biostatistics 13.4 (2012): 762-775.
[26] Li, B., and J. Leal. “Methods for detecting associations with rare variants for common diseases: application to analysis of sequence data.” The American Journal of Human Genetics 83.3 (2008): 311-321.
[27] Wu, M. C., et al. “Rare-variant association testing for sequencing data with the sequence kernel association test.” The American Journal of Human Genetics 89.1 (2011): 82-93.
Given the variability of rare genetic variants across populations, how might ancestry-specific reference databases enhance the accuracy of identifying T2D-related variants in diverse ethnic groups?
That’s a great point! Ancestry-specific databases would be incredibly valuable. By accounting for population-specific allele frequencies, we could better differentiate between truly pathogenic rare variants and benign, population-specific variants. This would significantly improve the accuracy of genetic screening and personalized medicine approaches for T2D in diverse ethnic groups. It could also assist with studies into the origin of the variants.
Editor: MedTechNews.Uk
Thank you to our Sponsor Esdebe
So, if rare variants in ANPEP impact insulin receptor signalling, does that mean CD13 inhibitors could be accidentally stumbling upon diabetes treatments? Maybe repurposing some oncology drugs isn’t so far-fetched after all!