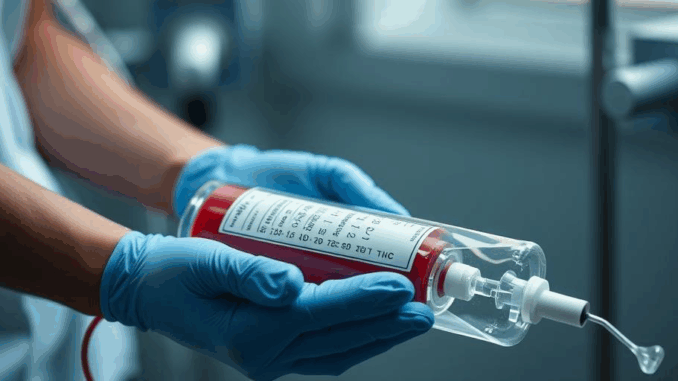
Abstract
The quest for a safe and effective red blood cell (RBC) substitute has been a long-standing goal in transfusion medicine. While allogeneic blood transfusion remains the standard of care, it is associated with inherent risks, including infectious disease transmission, transfusion reactions, and alloimmunization. Moreover, logistical challenges such as storage limitations and blood shortages, particularly during emergencies, underscore the urgent need for alternative oxygen carriers. This review provides a comprehensive overview of the diverse landscape of RBC substitutes, encompassing cell-free hemoglobin-based oxygen carriers (HBOCs), perfluorocarbons (PFCs), and cell-based approaches such as synthetic RBCs (e.g., Nano-RBCs) and stem cell-derived RBCs. We critically evaluate their mechanisms of action, advantages, disadvantages, and the challenges encountered during preclinical and clinical development. Specifically, we examine the progress in addressing issues such as toxicity, short half-life, vasoactivity, and immune response. Furthermore, we explore the regulatory hurdles, ethical considerations, and the potential market for RBC substitutes in various medical fields, including trauma, surgery, anemia management, and organ preservation. This review concludes with a perspective on the future directions of RBC substitute research, emphasizing the need for innovative strategies to overcome existing limitations and realize the promise of these life-saving technologies.
Many thanks to our sponsor Esdebe who helped us prepare this research report.
1. Introduction
Allogeneic blood transfusion has been a cornerstone of modern medicine for over a century, saving countless lives in cases of trauma, surgery, and various hematological disorders. However, reliance on donated blood is not without its limitations. The risks associated with transfusion, albeit minimized by stringent screening protocols, include the transmission of infectious diseases (e.g., HIV, hepatitis B and C), transfusion reactions (e.g., allergic, febrile non-hemolytic), and alloimmunization (e.g., the development of antibodies against RBC antigens). Furthermore, logistical challenges such as blood shortages, compatibility issues (especially in patients with rare blood types), and storage constraints necessitate the development of alternative oxygen-carrying solutions. These limitations have fueled the ongoing research and development of red blood cell substitutes (RBCs), also known as oxygen therapeutics, aiming to provide a safe, readily available, and universally compatible alternative to allogeneic blood. The ideal RBC substitute should possess several key characteristics, including: (1) efficient oxygen delivery and release to tissues; (2) long intravascular half-life; (3) minimal toxicity and immunogenicity; (4) ease of production and storage; and (5) compatibility with existing transfusion infrastructure.
The quest for an effective RBC substitute has been a long and arduous journey, spanning decades of research and development. Early attempts focused on modified hemoglobin solutions and perfluorocarbons, but these approaches faced significant challenges, including toxicity, short half-life, and adverse hemodynamic effects. More recently, advances in nanotechnology, biomaterials, and stem cell biology have opened new avenues for RBC substitute development, leading to the emergence of novel approaches such as synthetic RBCs (e.g., Nano-RBCs) and stem cell-derived RBCs. This review provides a comprehensive overview of the current landscape of RBC substitute research, examining the different types of RBC substitutes, their mechanisms of action, advantages, disadvantages, and the challenges encountered during preclinical and clinical development. We also explore the regulatory and ethical considerations surrounding the use of RBC substitutes and discuss their potential market in various medical fields.
Many thanks to our sponsor Esdebe who helped us prepare this research report.
2. Types of Red Blood Cell Substitutes
RBC substitutes can be broadly classified into two main categories: cell-free hemoglobin-based oxygen carriers (HBOCs) and perfluorocarbons (PFCs), alongside more recent developments in cell-based approaches. Each category possesses unique properties and has faced different challenges in its development.
2.1 Hemoglobin-Based Oxygen Carriers (HBOCs)
HBOCs are acellular solutions derived from purified hemoglobin. The basic principle behind HBOCs is to extract hemoglobin from red blood cells (either human or bovine) and modify it to improve its oxygen-carrying capacity, stability, and reduce its toxicity. Unmodified free hemoglobin is unsuitable for use as a blood substitute due to several reasons: rapid extravasation from the circulation, leading to nephrotoxicity; scavenging of nitric oxide (NO), resulting in vasoconstriction and increased blood pressure; and rapid degradation into toxic byproducts such as iron and bilirubin. To overcome these limitations, various modifications are employed to stabilize hemoglobin, increase its molecular weight, and reduce its NO scavenging activity. These modifications include polymerization, cross-linking, encapsulation, and conjugation with polyethylene glycol (PEG).
2.1.1 Polymerized HBOCs: Polymerization involves linking multiple hemoglobin molecules together to increase the molecular weight and reduce extravasation. Examples include Hemopure (bovine hemoglobin polymerized with glutaraldehyde) and PolyHeme (human hemoglobin polymerized with glutaraldehyde). While polymerization increases the intravascular half-life of hemoglobin, it can also lead to increased viscosity and potential for vasoactivity.
2.1.2 Cross-Linked HBOCs: Cross-linking involves chemically linking hemoglobin subunits to stabilize the tetrameric structure and prevent dissociation into dimers, which are rapidly cleared from the circulation. Examples include Hemolink (human hemoglobin cross-linked with raffinose) and DCLHb (diaspirin cross-linked hemoglobin). Cross-linking can improve the oxygen-binding affinity of hemoglobin and reduce its NO scavenging activity, but it can also alter its conformational stability and increase its susceptibility to oxidation.
2.1.3 Encapsulated HBOCs: Encapsulation involves enclosing hemoglobin within a liposome or other biocompatible carrier to protect it from degradation and reduce its toxicity. Examples include NeoHb (hemoglobin encapsulated in liposomes) and ErythroMer (hemoglobin encapsulated in a biodegradable polymer). Encapsulation can provide a protective barrier against NO scavenging and prevent direct contact between hemoglobin and plasma proteins, but it can also affect the oxygen-binding properties of hemoglobin and increase the complexity of the manufacturing process.
2.1.4 PEGylated HBOCs: PEGylation involves attaching polyethylene glycol (PEG) molecules to hemoglobin to increase its molecular weight, reduce its immunogenicity, and prolong its intravascular half-life. PEGylation can also improve the solubility and stability of hemoglobin, but it can also alter its oxygen-binding affinity and increase its viscosity.
Advantages of HBOCs: HBOCs offer several potential advantages over allogeneic blood, including universal compatibility (no need for blood typing), long shelf life, ease of storage and transportation, and reduced risk of infectious disease transmission. They also possess high oxygen-carrying capacity and can deliver oxygen to tissues even in situations where blood flow is compromised.
Disadvantages of HBOCs: Despite their potential benefits, HBOCs have faced several challenges in clinical development. The most significant challenges include: vasoactivity (due to NO scavenging), which can lead to increased blood pressure and vasoconstriction; toxicity (due to oxidative stress and iron release); short half-life (compared to natural RBCs); and potential for immune response (especially with bovine-derived HBOCs). Clinical trials with some HBOCs have shown increased rates of adverse events, including myocardial infarction, stroke, and renal failure, leading to their withdrawal from the market or restriction of their use to specific indications.
2.2 Perfluorocarbons (PFCs)
PFCs are synthetic organic compounds composed of carbon and fluorine atoms. They are inert, non-toxic, and capable of dissolving large amounts of oxygen. PFCs do not bind oxygen chemically like hemoglobin; instead, they dissolve oxygen proportionally to the partial pressure of oxygen in the surrounding environment. This property allows PFCs to deliver oxygen to tissues with low oxygen tension, but it also means that they are less efficient than hemoglobin at carrying oxygen in normoxic conditions. PFCs are typically administered as emulsions, as they are immiscible with water.
Advantages of PFCs: PFCs offer several advantages over allogeneic blood and HBOCs, including chemical inertness, resistance to degradation, long shelf life, and small particle size, which allows them to penetrate areas of microcirculatory obstruction. They also have no blood group antigens, eliminating the risk of transfusion reactions. Furthermore, some PFCs have been shown to enhance the immune response and promote wound healing.
Disadvantages of PFCs: The major disadvantages of PFCs include: low oxygen-carrying capacity (compared to hemoglobin), requiring high concentrations for effective oxygen delivery; short half-life (due to rapid clearance from the circulation); potential for pulmonary hypertension; and accumulation in the reticuloendothelial system (RES), leading to transient flu-like symptoms. Furthermore, the manufacturing of PFC emulsions can be complex and expensive.
2.3 Cell-Based Approaches: Synthetic RBCs (Nano-RBCs) and Stem Cell-Derived RBCs
Cell-based approaches represent a promising new direction in RBC substitute research. These approaches aim to create artificial RBCs that mimic the structure and function of natural RBCs or to generate functional RBCs from stem cells.
2.3.1 Synthetic RBCs (Nano-RBCs): Synthetic RBCs, often referred to as Nano-RBCs, are artificially engineered nanoparticles designed to mimic the oxygen-carrying capacity and biocompatibility of natural RBCs. These nanoparticles typically consist of a biodegradable polymer shell encapsulating hemoglobin. The polymer shell protects the hemoglobin from degradation and prevents it from scavenging NO. Nano-RBCs can be engineered to have specific size, shape, and surface properties to optimize their circulation time, oxygen delivery, and biocompatibility. Surface modifications, such as PEGylation or the addition of targeting ligands, can further enhance their performance.
Nano-RBCs hold significant promise as RBC substitutes due to their potential for high oxygen-carrying capacity, long circulation time, and reduced toxicity. However, challenges remain in scaling up their production, ensuring their long-term stability, and evaluating their potential for accumulation in the RES. Furthermore, the biocompatibility and immunogenicity of the polymer shell need to be carefully evaluated.
2.3.2 Stem Cell-Derived RBCs: Stem cell-derived RBCs offer the potential to generate a limitless supply of RBCs from pluripotent stem cells, such as embryonic stem cells (ESCs) or induced pluripotent stem cells (iPSCs). These stem cells can be differentiated into erythroid progenitors, which can then be further matured into functional RBCs. Stem cell-derived RBCs can be engineered to express specific blood group antigens, allowing for the production of RBCs that are compatible with different blood types. Furthermore, gene editing technologies can be used to correct genetic defects in stem cells, enabling the production of RBCs that are free from inherited hematological disorders.
Stem cell-derived RBCs offer a potentially ideal solution to the blood supply shortage, but significant challenges remain in scaling up their production, ensuring their complete differentiation into mature RBCs, and eliminating the risk of teratoma formation (in the case of ESC-derived RBCs). Furthermore, the cost of producing stem cell-derived RBCs is currently very high, making them less competitive with allogeneic blood transfusion.
Many thanks to our sponsor Esdebe who helped us prepare this research report.
3. Challenges in Red Blood Cell Substitute Development
The development of a safe and effective RBC substitute has been fraught with challenges. Several factors have contributed to the slow progress in this field, including:
3.1 Toxicity
Toxicity has been a major concern in the development of RBC substitutes, particularly HBOCs. Free hemoglobin can cause nephrotoxicity, oxidative stress, and inflammation. Modifications to hemoglobin, such as polymerization, cross-linking, and encapsulation, can reduce its toxicity, but they can also introduce new potential toxicities. For example, some polymers used to modify hemoglobin can be toxic themselves or can degrade into toxic byproducts. Furthermore, HBOCs can scavenge NO, leading to vasoconstriction and increased blood pressure. PFCs can accumulate in the RES, leading to transient flu-like symptoms. Careful selection of materials and rigorous preclinical and clinical testing are essential to minimize the toxicity of RBC substitutes.
3.2 Short Half-Life
The short intravascular half-life of RBC substitutes is another major limitation. Free hemoglobin is rapidly cleared from the circulation by the kidneys and the RES. Modifications to hemoglobin, such as polymerization and PEGylation, can prolong its half-life, but even these modifications may not be sufficient to achieve a clinically relevant duration of oxygen delivery. PFCs are also rapidly cleared from the circulation. Synthetic RBCs and stem cell-derived RBCs offer the potential for longer circulation times, but further research is needed to optimize their half-life.
3.3 Vasoactivity
Vasoactivity, or the ability to cause vasoconstriction, is a significant concern with HBOCs. This effect is primarily attributed to the scavenging of NO by free hemoglobin. NO is a potent vasodilator, and its depletion can lead to increased blood pressure and reduced blood flow to vital organs. Modifications to hemoglobin, such as cross-linking and encapsulation, can reduce its NO scavenging activity, but they may not completely eliminate it. The development of HBOCs that do not scavenge NO is a major goal of current research.
3.4 Immune Response
RBC substitutes can elicit an immune response, leading to allergic reactions or the formation of antibodies against the substitute. Bovine-derived HBOCs are particularly prone to causing immune reactions, as they contain non-human proteins. Even human-derived HBOCs can elicit an immune response in some individuals. PFCs are generally considered to be non-immunogenic, but some formulations can activate the complement system. Synthetic RBCs and stem cell-derived RBCs need to be carefully engineered to minimize their immunogenicity.
3.5 Regulatory Hurdles
The regulatory approval of RBC substitutes has been a complex and challenging process. Regulatory agencies, such as the FDA, require extensive preclinical and clinical data to demonstrate the safety and efficacy of RBC substitutes. The high bar set for safety and efficacy, combined with the high cost of clinical trials, has discouraged some companies from pursuing the development of RBC substitutes.
Many thanks to our sponsor Esdebe who helped us prepare this research report.
4. Ethical Considerations
The development and use of RBC substitutes raise several ethical considerations:
4.1 Informed Consent
Patients participating in clinical trials of RBC substitutes must be fully informed about the potential risks and benefits of the treatment. They must also be aware that RBC substitutes are not yet approved for general use and that they may not be as effective as allogeneic blood transfusion.
4.2 Access and Equity
If RBC substitutes are eventually approved for general use, it is important to ensure that they are accessible to all patients who need them, regardless of their socioeconomic status or geographic location. This may require government subsidies or other measures to reduce the cost of RBC substitutes.
4.3 Resource Allocation
The development of RBC substitutes requires significant investment of resources. It is important to consider whether these resources could be better allocated to other healthcare priorities, such as improving the blood supply or developing new treatments for other diseases.
4.4 Animal Welfare
Some RBC substitutes are derived from animal sources, such as bovine hemoglobin. The use of animals in research raises ethical concerns about animal welfare. It is important to ensure that animals are treated humanely and that the number of animals used is minimized.
Many thanks to our sponsor Esdebe who helped us prepare this research report.
5. Potential Market for Red Blood Cell Substitutes
The potential market for RBC substitutes is substantial, driven by the limitations of allogeneic blood transfusion and the increasing demand for blood in various medical fields.
5.1 Trauma
Trauma is a major cause of blood loss and the need for transfusion. RBC substitutes could be particularly valuable in trauma settings, where there is often a delay in obtaining compatible blood. The ability to rapidly administer an oxygen-carrying solution could save lives in these situations.
5.2 Surgery
Surgery is another major cause of blood loss and the need for transfusion. RBC substitutes could be used to reduce the need for allogeneic blood transfusion in elective surgeries, particularly in patients who are at risk of transfusion reactions or alloimmunization.
5.3 Anemia Management
RBC substitutes could be used to treat anemia in patients who are unable to receive allogeneic blood transfusion due to religious beliefs, autoimmune disorders, or other contraindications. They could also be used to treat anemia in patients with chronic kidney disease or cancer.
5.4 Organ Preservation
RBC substitutes could be used to improve the preservation of organs for transplantation. By delivering oxygen to organs during storage, RBC substitutes could prolong their viability and increase the success rate of transplantation.
5.5 Veterinary Medicine
The use of RBC substitutes extends to the veterinary field. Blood transfusions in animals can be complicated by species-specific blood types and the risk of transfusion reactions. RBC substitutes offer a potentially universal and readily available solution for treating anemia and blood loss in animals.
The market for RBC substitutes is estimated to be worth billions of dollars, and it is expected to grow significantly in the coming years as new and improved RBC substitutes are developed and approved.
Many thanks to our sponsor Esdebe who helped us prepare this research report.
6. Future Directions
The future of RBC substitute research is promising, with several new technologies and approaches showing potential for overcoming the limitations of existing RBC substitutes. Some of the key areas of future research include:
6.1 Novel Materials
The development of new biocompatible materials for encapsulating hemoglobin or constructing synthetic RBCs is crucial for improving the safety and efficacy of RBC substitutes. Researchers are exploring the use of biodegradable polymers, liposomes, and other nanomaterials to create RBC substitutes with improved properties.
6.2 Improved Hemoglobin Modifications
New and improved methods for modifying hemoglobin are needed to reduce its toxicity, prolong its half-life, and eliminate its vasoactivity. Researchers are exploring the use of site-specific PEGylation, genetic engineering, and other advanced techniques to create hemoglobin molecules with optimal properties.
6.3 Targeted Delivery
The development of RBC substitutes that can be targeted to specific tissues or organs could improve their efficacy and reduce their side effects. Researchers are exploring the use of targeting ligands, such as antibodies or peptides, to direct RBC substitutes to areas of hypoxia or inflammation.
6.4 Scalable Production
The development of scalable and cost-effective methods for producing RBC substitutes is essential for making them accessible to all patients who need them. Researchers are exploring the use of bioreactors, microfluidic devices, and other advanced manufacturing technologies to produce RBC substitutes on a large scale.
6.5 Clinical Trials
More clinical trials are needed to evaluate the safety and efficacy of RBC substitutes in different patient populations and clinical settings. These trials should be designed to address the limitations of previous trials and to provide definitive evidence of the benefits of RBC substitutes.
Specifically, advancements in protein engineering techniques allow for the creation of hemoglobin variants with reduced oxygen affinity and increased NO dioxygenase activity. This reduces both vasoactivity and the potential for tissue hypoxia. Furthermore, novel encapsulation strategies using biocompatible and biodegradable polymers with precisely controlled porosity can enhance the oxygen release kinetics and prolong the circulation time of nano-RBCs.
Moreover, the integration of microfluidic technology and bioreactor systems holds immense potential for the large-scale, cost-effective production of stem cell-derived RBCs. These systems can provide precisely controlled environments for cell differentiation and maturation, ensuring the consistent production of high-quality RBCs. Gene editing technologies, such as CRISPR-Cas9, can also be employed to enhance the functionality of stem cell-derived RBCs by modifying their oxygen-binding affinity or removing unwanted antigens.
Many thanks to our sponsor Esdebe who helped us prepare this research report.
7. Conclusion
The development of a safe and effective RBC substitute remains a significant challenge, but advances in nanotechnology, biomaterials, and stem cell biology are opening new avenues for research. While allogeneic blood transfusion will likely remain the standard of care for the foreseeable future, RBC substitutes have the potential to play an important role in specific clinical situations, such as trauma, surgery, and anemia management. Further research and development are needed to overcome the limitations of existing RBC substitutes and to realize their full potential. The ideal RBC substitute will possess a long circulation time, minimal toxicity, efficient oxygen delivery, and the ability to be produced on a large scale at a reasonable cost. While the road ahead is long, the potential benefits of a successful RBC substitute are enormous, and the quest for this life-saving technology is well worth pursuing.
Many thanks to our sponsor Esdebe who helped us prepare this research report.
References
- Chang, T. M. S. (2014). Artificial red blood cells: past, present, and future. International Journal of Artificial Organs, 37(3), 177-190.
- Jahr, J. S., & Lurie, F. (2003). Perflourocarbon Emulsions. Transfusion Alternatives in Transfusion Medicine, 5(1), 82-89.
- Winslow, R. M. (2006). Hemoglobin-based oxygen carriers. Critical Care Clinics, 22(4), 629-646.
- Palmer, A. F., & Intaglietta, M. (2000). Hemoglobin-based oxygen carriers: biochemical and physiological effects. Trends in Biotechnology, 18(12), 523-531.
- D’Agnillo, F., Hoffmann, T., & Kumar, A. (2017). Red blood cell substitutes: current status and future perspectives. Journal of Translational Medicine, 15(1), 1-14.
- Nemkov, T., D’Alessandro, A., Hansen, K. C., & Zimring, J. C. (2018). Red blood cell storage and transfusion: from bench to bedside. Blood, 131(25), 2807-2816.
- Kwak, Y. L., Kim, S. H., Lee, C. N., Koh, Y. S., Hong, K. J., & Kim, D. (2005). Vasoactivity of hemoglobin-based oxygen carriers. Anesthesiology, 102(5), 1038-1046.
- Hodgson, C. G., Cabrales, P., & Erni-Cassola, G. (2019). Stem cell-derived red blood cells: recent advances and challenges. Bioengineering & Translational Medicine, 4(4), e10139.
- Tarantal, A. F., & Diaz, P. M. (2019). Red blood cell substitutes: a review of current products and future directions. Journal of Biomedical Materials Research Part A, 107(1), 1-20.
- Sakai, H., & Takeoka, S. (2013). Artificial oxygen carriers: hemoglobin vesicles and albumin-hemes. Advanced Drug Delivery Reviews, 65(7), 933-946.
- Hammerstedt, R. H., Bessler, R., Day, R., & Winslow, R. M. (2016). Hemoglobin-based oxygen carriers: current state of the art and future prospects. Transfusion Medicine Reviews, 30(1), 47-54.
- Godet, S., Tachon, P., Barreau, O., Kellouche, S., & Mignon, A. (2012). The role of nitric oxide in the vasoconstrictive effects of cell-free hemoglobin. Anesthesia & Analgesia, 114(5), 938-947.
- Flanagan, C. (2015). Nano-RBC: A synthetic red blood cell substitute. Advanced Healthcare Materials, 4(14), 2111-2111.
- Antonucci, F., Miraglia, E., Casu, G., et al. (2020). Progress and challenges in the development of stem cell-derived red blood cells for transfusion purposes. Frontiers in Physiology, 11, 561669.
- Vandegriff, K. D. (2010). Oxygen delivery from hemoglobin-based oxygen carriers. Arteriosclerosis, Thrombosis, and Vascular Biology, 30(10), 1903-1905.
Stem cell-derived RBCs, eh? So, are we talking about designer blood types becoming a thing? Imagine ordering a specific blood profile like you’re choosing toppings for a pizza! Asking for a friend who is *tired* of blood transfusions.
That’s a great point! The potential for customizable blood profiles with stem cell-derived RBCs is fascinating. It could revolutionize how we approach transfusions, especially for those with rare blood types or who require frequent transfusions. We are working to make it a reality.
Editor: MedTechNews.Uk
Thank you to our Sponsor Esdebe
Given the challenges of toxicity and short half-life with HBOCs, how are researchers working to optimize the biocompatibility and circulation time of synthetic Nano-RBCs, specifically regarding long-term accumulation in the reticuloendothelial system?
That’s a crucial area of focus! Researchers are exploring surface modifications like PEGylation to minimize RES uptake and extend circulation time. Also, using biodegradable polymers allows for controlled degradation and excretion, further enhancing biocompatibility. These advancements are really promising!
Editor: MedTechNews.Uk
Thank you to our Sponsor Esdebe
The discussion of ethical considerations is vital. How might we balance the potential benefits of RBC substitutes, particularly in emergency situations, with the need for thorough long-term safety data, and what role should patient advocacy groups play in shaping clinical trial design and access?