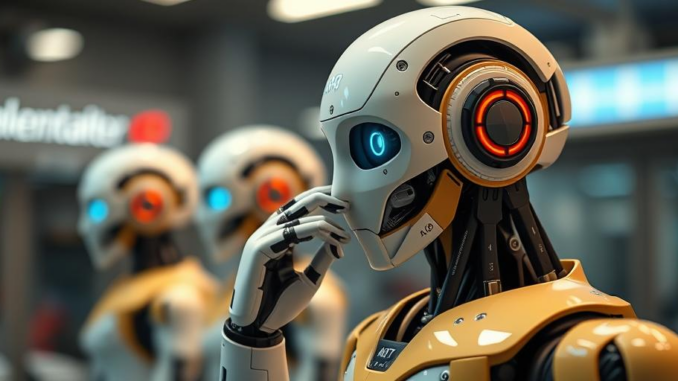
Abstract
Robotics has rapidly transformed various sectors, and medicine is no exception. This research report provides an in-depth exploration of the role of robotics in modern medicine, covering technological advancements, diverse applications beyond robotic surgery, the integration of artificial intelligence (AI), regulatory frameworks, economic considerations, ethical implications, and future directions. We delve into the progress made in surgical robotics, focusing on areas like minimally invasive procedures, including, but not limited to transcatheter mitral valve replacements (TMVR), and the ongoing development of autonomous surgical systems. Beyond surgery, the report examines robotic applications in rehabilitation, drug delivery, diagnostics, and assistive care. The integration of AI and machine learning for enhanced precision, personalized treatment planning, and real-time decision support is thoroughly discussed. We also address the challenges associated with the widespread adoption of medical robotics, including regulatory hurdles, economic constraints, ethical concerns related to autonomy and patient safety, and the evolving role of healthcare professionals in a technologically advanced environment. The report concludes by outlining potential future developments, such as nanorobotics, personalized medicine guided by AI-driven robotics, and the expanding role of robotics in addressing healthcare disparities and enhancing patient outcomes. This report aims to provide a comprehensive overview of the current state and future trajectory of robotics in medicine, targeted toward experts in the field.
Many thanks to our sponsor Esdebe who helped us prepare this research report.
1. Introduction
The convergence of engineering, computer science, and medicine has led to a paradigm shift in healthcare through the integration of robotics. From assisting surgeons with intricate procedures to providing rehabilitation for patients with disabilities, robotics has emerged as a versatile tool with the potential to revolutionize medical practice. The evolution of medical robotics is characterized by increasing precision, enhanced dexterity, improved visualization, and the ability to perform minimally invasive procedures, leading to reduced patient trauma, shorter recovery times, and improved clinical outcomes [1]. While robotic surgery, particularly with systems like the da Vinci Surgical System, has gained prominence, the applications of robotics in medicine extend far beyond the operating room. Rehabilitation robotics, automated drug delivery systems, robotic assistants for elderly care, and laboratory automation are just a few examples of the diverse ways in which robots are being utilized to improve healthcare delivery [2].
This research report aims to provide a comprehensive overview of the current state and future directions of robotics in medicine. It will explore the advancements in surgical robotics, including discussions of haptic feedback, augmented reality, and AI-driven automation. It will then delve into the applications of robotics in other areas of healthcare, such as rehabilitation, drug delivery, and diagnostics. The integration of AI and machine learning, which is enabling more sophisticated robotic systems with the ability to learn and adapt, will be examined in detail. The regulatory landscape, economic considerations, ethical implications, and challenges associated with the widespread adoption of medical robotics will also be addressed. Finally, the report will outline potential future developments and the role of robotics in addressing healthcare disparities and enhancing patient outcomes.
Many thanks to our sponsor Esdebe who helped us prepare this research report.
2. Advancements in Surgical Robotics
Surgical robotics has witnessed remarkable advancements in recent years, driven by the demand for minimally invasive procedures, enhanced precision, and improved surgical outcomes. The da Vinci Surgical System, developed by Intuitive Surgical, remains the most widely adopted surgical robot, but there are numerous other systems and emerging technologies that are pushing the boundaries of surgical capabilities [3].
2.1. Enhanced Dexterity and Precision
Robotic surgical systems offer greater dexterity and precision compared to traditional laparoscopic surgery. The EndoWrist technology, featured in the da Vinci system, allows surgeons to manipulate instruments with a range of motion that exceeds human capabilities. This is particularly beneficial in confined spaces and for intricate procedures, such as microsurgery and neurosurgery [4]. New robotic platforms are also incorporating haptic feedback, which provides surgeons with a sense of touch, improving their ability to distinguish between different tissues and apply appropriate forces during surgery. The lack of haptic feedback has been a long-standing criticism of early surgical robots, and its integration is a significant advancement [5].
2.2. Imaging and Navigation
Advanced imaging modalities, such as intraoperative MRI, CT, and ultrasound, are being integrated with surgical robots to provide real-time visualization and guidance. Augmented reality (AR) overlays, generated from preoperative imaging data, can be projected onto the surgical field, allowing surgeons to visualize underlying anatomical structures and plan their surgical approach more effectively [6]. Robotic navigation systems utilize tracking technologies to precisely guide surgical instruments to the target location, minimizing the risk of damage to surrounding tissues. These navigation systems are particularly useful in orthopedic surgery, neurosurgery, and spine surgery, where precise placement of implants is critical.
2.3. Artificial Intelligence and Automation
AI and machine learning are playing an increasingly important role in surgical robotics. AI algorithms can analyze preoperative imaging data to create personalized surgical plans, predict potential complications, and optimize surgical techniques. Machine learning models can be trained to recognize anatomical structures, identify pathological tissues, and assist surgeons in making real-time decisions [7]. The development of autonomous surgical systems is a long-term goal, but significant progress has been made in automating specific surgical tasks, such as suturing and tissue resection. These automated tasks can improve surgical efficiency, reduce surgical errors, and free up surgeons to focus on more complex aspects of the procedure. However, ethical concerns and regulatory challenges surrounding autonomous surgery need to be addressed before these systems can be widely adopted [8].
2.4. Specific Examples: TMVR and Beyond
Transcatheter mitral valve replacement (TMVR) exemplifies the benefits of robotics in minimally invasive cardiac surgery. Robotic assistance allows for precise navigation and deployment of the prosthetic valve, minimizing trauma to the heart and reducing the risk of complications compared to open-heart surgery [9]. The Corindus Vascular Robotics system, for example, enables remote control of guidewires and catheters during percutaneous coronary interventions. Beyond TMVR, robotic systems are being used in a wide range of surgical specialties, including urology, gynecology, general surgery, and cardiothoracic surgery. Specific examples include robotic prostatectomy, hysterectomy, and lung resection [10].
Many thanks to our sponsor Esdebe who helped us prepare this research report.
3. Robotics in Rehabilitation and Assistive Care
Beyond the operating room, robotics is making a significant impact in rehabilitation and assistive care, improving the quality of life for individuals with disabilities and mobility impairments.
3.1. Rehabilitation Robotics
Rehabilitation robots are used to assist patients in regaining motor function after stroke, spinal cord injury, or other neurological conditions. These robots provide repetitive, task-oriented training that can improve muscle strength, coordination, and range of motion [11]. Exoskeletons, which are wearable robotic devices, provide support and assistance to individuals with lower limb weakness, allowing them to stand, walk, and perform daily activities [12]. Virtual reality-based rehabilitation systems are also being developed, which provide engaging and motivating training environments for patients. The integration of biofeedback and neural interfaces allows rehabilitation robots to adapt to the patient’s individual needs and provide personalized therapy.
3.2. Assistive Robotics
Assistive robots are designed to help individuals with disabilities perform daily tasks, such as eating, dressing, bathing, and using the toilet. These robots can be controlled using voice commands, eye tracking, or brain-computer interfaces, allowing individuals with severe motor impairments to regain some independence [13]. Companion robots, which are social robots designed to provide companionship and emotional support, are also being developed for elderly individuals and individuals with cognitive impairments. These robots can engage in conversation, play games, and remind individuals to take their medication [14].
3.3. Challenges and Future Directions
Despite the potential benefits of rehabilitation and assistive robotics, there are several challenges that need to be addressed. The cost of these robots can be prohibitive for many individuals, and the technology needs to be more user-friendly and adaptable to different environments. Further research is needed to optimize rehabilitation protocols and personalize therapy based on individual patient needs. The development of more affordable, accessible, and user-friendly robots will be crucial for widespread adoption [15].
Many thanks to our sponsor Esdebe who helped us prepare this research report.
4. Robotics in Drug Delivery and Diagnostics
Robotics is also playing an increasingly important role in drug delivery and diagnostics, offering the potential for more targeted, precise, and efficient treatments.
4.1. Targeted Drug Delivery
Robotic drug delivery systems can deliver medication directly to the site of disease, minimizing systemic side effects and improving therapeutic efficacy. Micro- and nanorobots, which are tiny robots that can navigate through the bloodstream, are being developed to deliver drugs to tumors, infected tissues, and other specific targets [16]. These robots can be controlled using external magnetic fields, ultrasound, or light. Robotic implants, which are drug-eluting devices that can be implanted into the body, can provide sustained release of medication over extended periods [17].
4.2. Automated Diagnostics
Robotic systems are being used to automate laboratory processes, such as sample preparation, DNA sequencing, and drug screening. These automated systems can improve the speed, accuracy, and reproducibility of diagnostic tests, reducing human error and improving laboratory efficiency [18]. Robotic imaging systems, such as automated microscopy and robotic endoscopy, can provide high-resolution images of tissues and organs, aiding in the diagnosis of disease. AI algorithms can be used to analyze these images and identify subtle abnormalities that may be missed by human observers [19].
4.3. Nanorobotics: A Futuristic Vision
The field of nanorobotics, while still largely theoretical, holds immense promise for future medical applications. Nanorobots, operating at the nanoscale, could potentially perform complex tasks such as targeted drug delivery at the cellular level, repairing damaged tissues, and even destroying cancerous cells. While significant technological hurdles remain, ongoing research is exploring the feasibility of nanorobots for various medical applications [20].
Many thanks to our sponsor Esdebe who helped us prepare this research report.
5. Integration of Artificial Intelligence and Machine Learning
The integration of AI and machine learning is transforming medical robotics, enabling more sophisticated and autonomous systems that can learn, adapt, and make real-time decisions.
5.1. Image-Guided Surgery
AI algorithms can analyze medical images, such as CT scans and MRIs, to create personalized surgical plans and guide surgical robots during procedures. These algorithms can automatically segment anatomical structures, identify pathological tissues, and predict potential complications. Machine learning models can be trained to recognize patterns in medical images and assist surgeons in making real-time decisions [21].
5.2. Personalized Treatment Planning
AI can analyze patient data, including medical history, genetic information, and lifestyle factors, to create personalized treatment plans. Machine learning models can predict patient response to different treatments and optimize treatment strategies based on individual patient characteristics. This personalized approach to medicine has the potential to improve treatment outcomes and reduce side effects [22].
5.3. Autonomous Robotics
The development of autonomous surgical systems is a long-term goal, but significant progress has been made in automating specific surgical tasks. AI algorithms can be trained to perform tasks such as suturing, tissue resection, and instrument manipulation. These automated tasks can improve surgical efficiency, reduce surgical errors, and free up surgeons to focus on more complex aspects of the procedure. However, ethical concerns and regulatory challenges surrounding autonomous surgery need to be addressed before these systems can be widely adopted [23]. The level of autonomy should also be carefully considered in each application; a shared control approach where the human surgeon retains overriding control may be more appropriate than full autonomy in many situations.
Many thanks to our sponsor Esdebe who helped us prepare this research report.
6. Regulatory Landscape and Economic Considerations
The widespread adoption of medical robotics is influenced by the regulatory landscape and economic considerations, which can impact the development, approval, and reimbursement of robotic technologies.
6.1. Regulatory Approval
Medical robots are subject to stringent regulatory requirements, ensuring their safety and efficacy. The U.S. Food and Drug Administration (FDA) and other regulatory agencies around the world review and approve medical robots based on preclinical and clinical data. The approval process can be lengthy and expensive, which can delay the introduction of new robotic technologies to the market [24].
6.2. Reimbursement and Cost-Effectiveness
The cost of medical robots can be substantial, which can limit their accessibility. Hospitals and healthcare providers need to justify the investment in robotic technologies by demonstrating their cost-effectiveness. Reimbursement policies, which determine how much insurance companies will pay for robotic procedures, can also impact the adoption of medical robotics. Studies are needed to compare the cost-effectiveness of robotic procedures to traditional methods and to identify the clinical indications for which robotic surgery offers the greatest benefit [25]. A deeper understanding of long-term outcomes and cost savings associated with reduced complications and shorter hospital stays is crucial.
6.3 Liability and Safety
The safety of medical robotic systems is paramount. Clear guidelines and protocols are needed to ensure that robots are used safely and effectively. Training programs for surgeons and other healthcare professionals are essential to ensure that they are proficient in the use of robotic technologies. Liability issues related to robotic surgery need to be addressed, including the responsibility for errors made by the robot. Establishing robust safety protocols, comprehensive training programs, and clear lines of responsibility is crucial for building trust in robotic medical devices [26].
Many thanks to our sponsor Esdebe who helped us prepare this research report.
7. Ethical Implications and Challenges
The increasing use of robotics in medicine raises several ethical implications and challenges that need to be carefully considered.
7.1. Autonomy and Control
The level of autonomy in medical robots raises questions about the role of human judgment and control. How much autonomy should be granted to a robot in making decisions about patient care? Who is responsible for errors made by the robot? These questions need to be addressed through careful consideration of the ethical principles of autonomy, beneficence, non-maleficence, and justice [27].
7.2. Patient Safety and Privacy
Patient safety is a paramount concern in medical robotics. Robots need to be designed and tested to ensure that they are safe and reliable. Data privacy is another important consideration. Medical robots generate vast amounts of data about patients, which needs to be protected from unauthorized access and misuse. Robust cybersecurity measures are needed to prevent hacking and data breaches [28].
7.3. Accessibility and Equity
The cost of medical robots can limit their accessibility, potentially exacerbating healthcare disparities. Efforts need to be made to ensure that robotic technologies are available to all patients, regardless of their socioeconomic status or geographic location. Tele-robotic surgery, which allows surgeons to remotely control robots from distant locations, could potentially improve access to specialized surgical care in underserved areas [29].
Many thanks to our sponsor Esdebe who helped us prepare this research report.
8. Future Directions
The field of robotics in medicine is rapidly evolving, with numerous potential future directions that could transform healthcare delivery.
8.1. Nanorobotics and Personalized Medicine
Nanorobotics, as previously discussed, holds immense promise for targeted drug delivery and cellular-level interventions. Combining nanorobotics with personalized medicine approaches, guided by AI-driven diagnostics, could revolutionize treatment strategies [30]. This would involve tailoring treatments to individual patients based on their unique genetic makeup, lifestyle, and environmental factors.
8.2. Soft Robotics and Biocompatible Materials
Traditional robots are often made of rigid materials, which can be problematic for applications involving contact with delicate tissues. Soft robotics, which utilizes flexible and deformable materials, offers a more biocompatible and adaptable approach. These robots can conform to the shape of the body and minimize the risk of tissue damage. The development of biocompatible materials that can be integrated into robotic systems is another important area of research [31].
8.3. Telepresence and Remote Surgery
Telepresence robots, which allow surgeons to remotely control robots from distant locations, could improve access to specialized surgical care in underserved areas. These robots can also be used to provide remote consultations and training [32]. The development of reliable and secure communication networks is essential for the success of telepresence surgery.
8.4. Collaborative Robots (Cobots)
Cobots are designed to work alongside human healthcare professionals, assisting with tasks such as patient transport, medication dispensing, and equipment sterilization. These robots can improve efficiency, reduce workload, and minimize the risk of human error [33].
Many thanks to our sponsor Esdebe who helped us prepare this research report.
9. Conclusion
Robotics is transforming medicine in profound ways, offering the potential for more precise, efficient, and personalized healthcare. From surgical robotics and rehabilitation to drug delivery and diagnostics, robots are being used to improve patient outcomes and enhance the quality of life for individuals with disabilities. The integration of AI and machine learning is enabling more sophisticated and autonomous robotic systems, while ongoing research is exploring new applications and future directions. However, the widespread adoption of medical robotics requires addressing regulatory hurdles, economic constraints, ethical concerns, and the evolving role of healthcare professionals in a technologically advanced environment. By carefully considering these challenges and embracing innovation, we can harness the power of robotics to create a more equitable, accessible, and effective healthcare system for all.
Many thanks to our sponsor Esdebe who helped us prepare this research report.
References
[1] Lanfranco AR, Castellanos AE, Desai JP, Meyers WC. Robotic surgery: a current perspective. Ann Surg. 2004;239(1):14-21.
[2] Dario P, Allotta B, Guglielmelli E. Robotics for medical applications. IEEE Robot Autom Mag. 2003;10(1):4-6.
[3] Hung AJ, Patel VR. Robotic surgery: current status and future directions. Urology. 2008;71(5):761-8.
[4] Ballantyne GH. Robotic surgery: evolution, rationale, and future directions. Dis Colon Rectum. 2002;45(1):1-18.
[5] Okamura AM. Haptic feedback in robot-assisted surgery. Curr Opin Urol. 2009;19(1):102-7.
[6] Marescaux J, Rubino F, Arenas RB, Mutter D, Soler L, Diana M. Augmented reality in surgery. Br J Surg. 2015;102(6):515-22.
[7] Hashimoto DA, Witkowski E, Kamel MK, et al. Artificial intelligence in surgery. Br J Surg. 2018;105(13):1579-89.
[8] Yang GZ, Bellingham J, Dupont PE, et al. The grand challenges of Science Robotics. Sci Robot. 2016;1(1):eaaf7650.
[9] Reardon MJ, Van Mieghem NM, Popma JJ, et al. Surgical or Transcatheter Aortic-Valve Replacement in High-Risk Patients. N Engl J Med. 2017;376(14):1321-31.
[10] Smith JA Jr, Chan RC, Chang SS, et al. Robotic-assisted radical prostatectomy: early results from a prospective randomized trial. J Urol. 2012;187(5):1644-50.
[11] Hussain S, Jamwal PK, Ghai S, et al. Robot-assisted rehabilitation: a systematic review. Adv Robot. 2016;30(17):1145-61.
[12] Dollar AM, Herr H. Lower extremity exoskeletons and active orthoses: challenges and state-of-the-art. IEEE Trans Robot. 2008;24(1):144-58.
[13] Feil-Seifer D, Matarić MJ. Socially assistive robotics. IEEE Robot Autom Mag. 2011;18(1):69-78.
[14] Broadbent E, Stafford R, MacDonald B. Acceptance of healthcare robots for the elderly. Int J Soc Robot. 2009;1(4):319-30.
[15] Krebs HI, Hogan N, Aisen ML, Flash T. Robot-aided neurorehabilitation. IEEE Trans Rehabil Eng. 1998;6(1):75-87.
[16] Li J, Esteban-Fernández de Ávila B, Gao W, Zhang L, Wang J. Micro/nanomachines for drug delivery. Adv Drug Deliv Rev. 2017;114:3-20.
[17] Farra R, Alcântara AC, Khoury N, Greige-Gerges H, Fajloun Z. Biodegradable polymers for biomedical applications. Molecules. 2021;26(19):5870.
[18] Hawkins T, O’Neil J, Hoeman CM. Robotics in the clinical laboratory. Clin Lab Med. 2007;27(3):725-42.
[19] Gurcan MN, Boucheron LE, Can A, et al. Histopathological image analysis: a review. IEEE Rev Biomed Eng. 2009;2:147-71.
[20] Freitas RA Jr. Nanomedicine, Volume I: Basic Capabilities. Landes Bioscience; 1999.
[21] Esteva A, Kuprel B, Novoa RA, et al. Dermatologist-level classification of skin cancer with deep neural networks. Nature. 2017;542(7639):115-8.
[22] Ginsburg GS, Willard HF. Genomic and personalized medicine: foundations and applications. Transl Res. 2009;154(6):277-87.
[23] Shademan A, Decker RS, Opfermann JD, Leonard S, Krieger A, Kim PC. Robot-assisted laparoscopic surgery: a systematic review. World J Surg. 2009;33(12):2462-82.
[24] Bonfield TL, Sacristán J. The regulatory landscape of medical device innovation. Expert Rev Med Devices. 2010;7(2):201-13.
[25] Barbash GI, Glied SA. Technology and health care: a survey. Health Aff (Millwood). 2010;29(1):14-21.
[26] Dhillon PS, Stefanidis D, Korndorffer JR Jr, Scott DJ. Medicolegal issues in robotic surgery. Am J Surg. 2010;200(3):386-90.
[27] Beauchamp TL, Childress JF. Principles of biomedical ethics. 7th ed. Oxford University Press; 2013.
[28] Price WN II, Cohen IG. Privacy in the age of medical big data. Nat Biotechnol. 2019;37(1):59-69.
[29] Marescaux J, Leroy J, Gagner M, et al. Transatlantic robot-assisted telesurgery. Nature. 2001;413(6854):379-80.
[30] Patel G, Yelleswarapu V, McEachern D, Pamarthy S, Boktor SW. Nanorobotics for biomedical applications. Int J Nanomedicine. 2019;14:7491-505.
[31] Rus D, Tolley MT. Design, fabrication and control of soft robots. Nature. 2015;521(7553):467-75.
[32] Cavusoglu MC, Viswanathan A, Taylor RH. Telepresence and telesurgery: current status and future trends. IEEE Eng Med Biol Mag. 2006;25(5):24-32.
[33] Matthias B, Weisshardt R, Strassburger L, Kuhn W. Man-machine interaction in surgery. Minim Invasive Ther Allied Technol. 2009;18(1):3-12.
The advancements in AI-driven surgical planning are particularly exciting. As these systems become more sophisticated, how do you envision the role of the surgeon evolving – will they become more like supervisors or collaborators with the robotic system?
That’s a great point! The role of the surgeon is definitely evolving. I think the future will see surgeons as collaborators, leveraging AI for enhanced insights but maintaining critical oversight and decision-making. It’s about combining the best of human expertise with AI’s analytical power to improve patient outcomes together.
Editor: MedTechNews.Uk
Thank you to our Sponsor Esdebe
Suturing and tissue resection by robots – fascinating! But if the AI glitches, do we blame the algorithm, the surgeon, or Esdebe? Just curious how the liability shakes out when Skynet’s holding the scalpel.
That’s a brilliant question! The evolving legal and ethical landscape around AI in surgery is definitely something that needs careful consideration. As these technologies advance, we need to consider how liability is determined in different scenarios. Perhaps a collaborative framework, or some form of shared responsibility? It is an important discussion.
Editor: MedTechNews.Uk
Thank you to our Sponsor Esdebe
So, if nanorobots *can* destroy cancer cells, will they unionize and demand better working conditions, or is that just a sci-fi movie plot waiting to happen? Asking for a friend… a very small, metallic friend.
That’s a fantastic question! Thinking about nanorobot ‘working conditions’ opens up fascinating discussions about the resources required to power and maintain them. Perhaps future research will focus on sustainable energy solutions for these tiny machines, ensuring ethical and efficient operation!
Editor: MedTechNews.Uk
Thank you to our Sponsor Esdebe