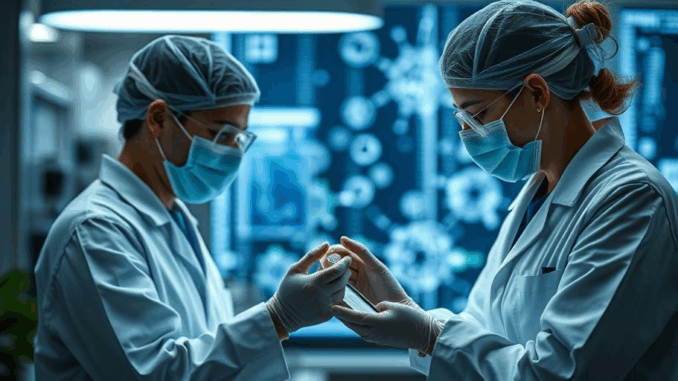
Abstract
Stem cell therapy holds immense promise for treating a wide range of diseases, from degenerative conditions to autoimmune disorders. This report provides a comprehensive overview of the current landscape of stem cell research, focusing on the different types of stem cells utilized, their mechanisms of action, clinical trial progress, associated challenges, and ethical considerations. While the potential of stem cell therapy is undeniable, significant hurdles remain in translating preclinical findings into safe and effective clinical applications. We delve into the specific applications of stem cell therapy, including, but not limited to, regenerative medicine, immune modulation, and cancer treatment. We further scrutinize the obstacles related to stem cell differentiation, targeted delivery, immune rejection, and long-term safety. Finally, this report examines the ethical frameworks surrounding stem cell research and provides a perspective on the future directions and innovations required to fully realize the therapeutic potential of stem cell therapy.
Many thanks to our sponsor Esdebe who helped us prepare this research report.
1. Introduction
Stem cell therapy represents a paradigm shift in medicine, offering the possibility of repairing or replacing damaged tissues and organs, and modulating the immune system. Unlike traditional treatments that primarily address symptoms, stem cell therapy aims to target the underlying causes of diseases by harnessing the regenerative and immunomodulatory capabilities of stem cells. This nascent field has captured the attention of researchers, clinicians, and patients alike, driven by the promise of treating previously incurable conditions. However, the complexity of stem cell biology and the challenges associated with translating preclinical findings into clinical practice necessitate a cautious and rigorous approach.
The field of stem cell therapy is broad, encompassing a variety of cell types, differentiation protocols, and delivery methods. Embryonic stem cells (ESCs), derived from the inner cell mass of blastocysts, possess the remarkable ability to differentiate into any cell type in the body, making them a valuable resource for regenerative medicine. Induced pluripotent stem cells (iPSCs), generated by reprogramming somatic cells, offer a patient-specific alternative to ESCs, potentially circumventing the issue of immune rejection. Adult stem cells, found in various tissues throughout the body, have a more restricted differentiation potential but offer the advantage of being readily accessible and autologous. Furthermore, mesenchymal stem cells (MSCs) have gained attention due to their immunomodulatory properties, making them valuable for treating autoimmune and inflammatory diseases.
This report will delve into the various aspects of stem cell therapy, including the different types of stem cells, their mechanisms of action, the current status of clinical trials, the challenges associated with their use, and the ethical considerations surrounding stem cell research.
Many thanks to our sponsor Esdebe who helped us prepare this research report.
2. Types of Stem Cells
2.1 Embryonic Stem Cells (ESCs)
ESCs are derived from the inner cell mass of a blastocyst, the early-stage embryo formed a few days after fertilization. These cells are characterized by their pluripotency, meaning they can differentiate into any cell type in the body. ESCs hold immense potential for regenerative medicine due to their unlimited self-renewal capacity and differentiation potential. However, the use of ESCs raises ethical concerns related to the destruction of embryos, and their inherent pluripotency can also lead to the formation of teratomas, benign tumors containing a mixture of differentiated tissues.
To address the ethical concerns, researchers have explored alternative methods of obtaining pluripotent stem cells, such as somatic cell nuclear transfer (SCNT) and parthenogenesis. SCNT involves transferring the nucleus of a somatic cell into an enucleated oocyte, while parthenogenesis involves the activation of an oocyte without fertilization. These methods can generate ESCs without creating or destroying viable embryos, but they are technically challenging and less efficient than traditional ESC derivation.
2.2 Induced Pluripotent Stem Cells (iPSCs)
iPSCs are generated by reprogramming somatic cells, such as skin or blood cells, into a pluripotent state using a specific set of transcription factors. This groundbreaking discovery by Shinya Yamanaka revolutionized the field of stem cell research, as it provided a means of generating patient-specific pluripotent stem cells without the need for embryos. iPSCs share many characteristics with ESCs, including their pluripotency and self-renewal capacity. However, iPSCs may exhibit epigenetic differences compared to ESCs, and the reprogramming process can introduce genetic mutations.
The development of iPSC technology has significantly advanced stem cell therapy, enabling the creation of personalized cell therapies tailored to individual patients. iPSCs can be differentiated into a wide range of cell types, including cardiomyocytes, neurons, and pancreatic beta cells, offering potential treatments for heart disease, neurodegenerative disorders, and diabetes. Furthermore, iPSCs can be used to create disease models in vitro, allowing researchers to study the mechanisms of disease and develop new therapies.
2.3 Adult Stem Cells
Adult stem cells, also known as somatic stem cells, are found in various tissues throughout the body. These cells have a more restricted differentiation potential than ESCs and iPSCs, typically differentiating into cell types within the tissue of origin. Adult stem cells play a crucial role in tissue maintenance and repair, and they have been used in clinical applications for decades, particularly in bone marrow transplantation.
Examples of adult stem cells include hematopoietic stem cells (HSCs) in bone marrow, mesenchymal stem cells (MSCs) in bone marrow, adipose tissue, and other tissues, and neural stem cells in the brain. HSCs are responsible for generating all blood cell types, while MSCs have immunomodulatory and regenerative properties. Neural stem cells can differentiate into neurons, astrocytes, and oligodendrocytes. Adult stem cells offer the advantage of being readily accessible and autologous, minimizing the risk of immune rejection. However, they are often present in small numbers and have a limited differentiation potential.
2.4 Mesenchymal Stem Cells (MSCs)
MSCs are multipotent stromal cells that can be isolated from various tissues, including bone marrow, adipose tissue, and umbilical cord blood. MSCs are characterized by their ability to differentiate into mesenchymal lineage cells, such as osteoblasts, chondrocytes, and adipocytes. However, MSCs also exhibit immunomodulatory properties, secreting factors that suppress immune cell activity and promote tissue repair. These properties have made MSCs a popular choice for treating autoimmune and inflammatory diseases.
MSCs exert their immunomodulatory effects through various mechanisms, including the secretion of cytokines, such as IL-10 and TGF-β, which suppress T cell proliferation and activity. MSCs can also interact directly with immune cells, such as T cells, B cells, and dendritic cells, modulating their function. Furthermore, MSCs can recruit other immune cells to the site of injury, promoting tissue repair and regeneration. MSCs have been shown to be effective in treating a variety of autoimmune and inflammatory diseases, including graft-versus-host disease (GVHD), rheumatoid arthritis, and multiple sclerosis.
Many thanks to our sponsor Esdebe who helped us prepare this research report.
3. Mechanisms of Action
The mechanisms of action of stem cell therapy are complex and vary depending on the type of stem cells used, the target tissue, and the disease being treated. In general, stem cell therapy can exert its therapeutic effects through several mechanisms, including:
3.1 Cell Replacement
In cell replacement therapy, stem cells are differentiated into the desired cell type and transplanted into the patient to replace damaged or dysfunctional cells. This approach is particularly relevant for diseases characterized by cell loss, such as Parkinson’s disease (dopaminergic neurons) and type 1 diabetes (pancreatic beta cells). For example, in the case of type 1 diabetes, stem cells can be differentiated into insulin-producing beta cells and transplanted into the pancreas to restore insulin production. The success of cell replacement therapy depends on several factors, including the efficiency of stem cell differentiation, the survival and function of the transplanted cells, and the integration of the transplanted cells into the host tissue.
3.2 Immunomodulation
Stem cells, particularly MSCs, can modulate the immune system, suppressing inflammation and promoting immune tolerance. This mechanism is crucial for treating autoimmune diseases, such as rheumatoid arthritis and multiple sclerosis, where the immune system attacks the body’s own tissues. MSCs exert their immunomodulatory effects through various mechanisms, including the secretion of cytokines that suppress immune cell activity and the direct interaction with immune cells. By modulating the immune system, MSCs can reduce inflammation, prevent tissue damage, and promote tissue repair.
3.3 Trophic Effects
Stem cells can secrete factors, such as growth factors and cytokines, that promote tissue repair and regeneration. These trophic factors can stimulate cell proliferation, angiogenesis, and extracellular matrix remodeling, leading to improved tissue function. The trophic effects of stem cells can be beneficial in treating a variety of diseases, including heart disease, stroke, and spinal cord injury. For example, in the case of heart disease, stem cells can secrete factors that promote angiogenesis, improving blood flow to the damaged heart tissue and reducing ischemia.
3.4 Exosome-Mediated Effects
Stem cells release extracellular vesicles called exosomes, which contain various bioactive molecules, including proteins, mRNA, and microRNA. These exosomes can be taken up by recipient cells, delivering their cargo and altering cell function. Exosomes can mediate many of the therapeutic effects of stem cells, including immunomodulation, tissue repair, and angiogenesis. Exosome-based therapy is a promising alternative to cell-based therapy, as it eliminates the need for cell transplantation and reduces the risk of immune rejection. However, the optimization of exosome production, purification, and delivery is essential for the successful translation of exosome-based therapy into clinical practice.
Many thanks to our sponsor Esdebe who helped us prepare this research report.
4. Current Status of Clinical Trials
Stem cell therapy is currently being investigated in numerous clinical trials for a wide range of diseases. While some stem cell therapies have been approved for clinical use, such as hematopoietic stem cell transplantation for blood cancers, many others are still in the experimental phase. The progress of clinical trials has been varied, with some showing promising results and others failing to meet expectations.
4.1 Hematopoietic Stem Cell Transplantation (HSCT)
HSCT is a well-established stem cell therapy for treating blood cancers, such as leukemia and lymphoma, as well as other blood disorders, such as aplastic anemia and sickle cell disease. In HSCT, the patient’s own bone marrow or blood cells are destroyed by chemotherapy or radiation, and then replaced with healthy stem cells from a donor or the patient themselves (autologous transplant). HSCT can be life-saving for patients with these diseases, but it is also associated with significant risks, including graft-versus-host disease (GVHD), infection, and organ damage.
4.2 Stem Cell Therapy for Cardiovascular Diseases
Stem cell therapy is being investigated for treating various cardiovascular diseases, including heart failure, myocardial infarction, and peripheral artery disease. Several clinical trials have shown that stem cell therapy can improve cardiac function, reduce infarct size, and increase blood flow to the affected tissue. However, the results of these trials have been inconsistent, and the optimal cell type, dose, and delivery method are still being investigated. The mechanisms of action of stem cell therapy in cardiovascular diseases are thought to involve angiogenesis, cardioprotection, and immunomodulation.
4.3 Stem Cell Therapy for Neurological Disorders
Stem cell therapy is being explored for treating a variety of neurological disorders, including Parkinson’s disease, Alzheimer’s disease, multiple sclerosis, and spinal cord injury. Clinical trials have shown some promise for improving motor function, cognitive function, and quality of life in patients with these disorders. However, the results have been mixed, and the challenges associated with delivering stem cells to the brain and spinal cord, as well as the complexity of these disorders, pose significant hurdles. The mechanisms of action of stem cell therapy in neurological disorders are thought to involve neuroprotection, neurotrophic support, and remyelination.
4.4 Stem Cell Therapy for Autoimmune Diseases
Stem cell therapy, particularly using MSCs, is being investigated for treating various autoimmune diseases, including rheumatoid arthritis, multiple sclerosis, and Crohn’s disease. Clinical trials have shown that MSCs can reduce inflammation, suppress immune cell activity, and improve symptoms in patients with these diseases. The immunomodulatory properties of MSCs are thought to be the primary mechanism of action in autoimmune diseases. However, the long-term efficacy and safety of MSC therapy for autoimmune diseases are still being investigated.
Many thanks to our sponsor Esdebe who helped us prepare this research report.
5. Challenges
While stem cell therapy holds immense promise, significant challenges remain in translating preclinical findings into safe and effective clinical applications.
5.1 Immunosuppression
A major challenge in stem cell therapy is immune rejection of the transplanted cells. To prevent immune rejection, patients often require immunosuppressive drugs, which can have significant side effects, including increased risk of infection and cancer. The development of strategies to minimize or eliminate the need for immunosuppression is a major goal in stem cell research. These strategies include the use of autologous stem cells (derived from the patient’s own body), genetic modification of stem cells to make them less immunogenic, and the use of immunomodulatory agents to promote immune tolerance.
5.2 Long-Term Efficacy
The long-term efficacy of stem cell therapy is another major concern. While some clinical trials have shown promising short-term results, the long-term benefits of stem cell therapy are often unclear. It is essential to conduct long-term follow-up studies to assess the durability of the therapeutic effects and to identify any potential long-term side effects. Furthermore, the development of strategies to enhance the survival and function of the transplanted cells is crucial for improving the long-term efficacy of stem cell therapy.
5.3 Stem Cell Differentiation and Targeted Delivery
Efficient and targeted differentiation of stem cells into the desired cell type is essential for successful cell replacement therapy. However, achieving complete and uniform differentiation can be challenging, and the presence of undifferentiated stem cells can lead to the formation of teratomas. The development of robust and reproducible differentiation protocols is crucial for ensuring the safety and efficacy of stem cell therapy. Furthermore, targeted delivery of stem cells to the site of injury or disease is essential for maximizing their therapeutic effects. This can be achieved using various methods, including direct injection, catheter-based delivery, and targeted delivery using nanoparticles.
5.4 Tumorigenicity
A major safety concern associated with stem cell therapy is the risk of tumorigenicity. ESCs and iPSCs, in particular, have the potential to form teratomas due to their inherent pluripotency. While the risk of teratoma formation can be reduced by careful differentiation and purification of stem cells, it is essential to monitor patients for signs of tumor formation after stem cell transplantation. The use of adult stem cells, which have a more restricted differentiation potential, may reduce the risk of tumorigenicity. However, adult stem cells may also have a limited capacity for proliferation and differentiation.
5.5 Standardized Protocols and Regulations
The lack of standardized protocols and regulations for stem cell therapy poses a significant challenge. The variability in cell sources, differentiation protocols, and delivery methods makes it difficult to compare results across different studies and to ensure the safety and efficacy of stem cell therapies. The development of standardized protocols and regulations is essential for promoting the responsible development and clinical translation of stem cell therapy. Regulatory agencies, such as the FDA, play a crucial role in overseeing the development and approval of stem cell therapies.
Many thanks to our sponsor Esdebe who helped us prepare this research report.
6. Ethical Considerations
Stem cell research and therapy raise several ethical considerations, particularly regarding the use of ESCs and the potential for reproductive cloning. The destruction of embryos to derive ESCs raises concerns about the moral status of the embryo and the sanctity of human life. The development of iPSC technology has alleviated some of these concerns, but ethical considerations remain regarding the sourcing and use of human cells for reprogramming. Reproductive cloning, which involves creating a genetically identical copy of an individual, raises concerns about human dignity, autonomy, and the potential for misuse of the technology.
Furthermore, the commercialization of stem cell therapy raises ethical concerns about accessibility, affordability, and the potential for unproven and potentially harmful treatments being offered to vulnerable patients. It is essential to ensure that stem cell therapies are developed and implemented in a responsible and ethical manner, with appropriate oversight and regulation. The ethical frameworks surrounding stem cell research should be based on principles of respect for persons, beneficence, non-maleficence, and justice.
Many thanks to our sponsor Esdebe who helped us prepare this research report.
7. Future Directions
The future of stem cell therapy is bright, with ongoing research aimed at overcoming the current challenges and expanding the therapeutic applications of stem cells. Some promising future directions include:
7.1 Development of Advanced Differentiation Protocols
The development of more efficient and targeted differentiation protocols is crucial for generating specific cell types for cell replacement therapy. This includes the use of small molecules, growth factors, and three-dimensional culture systems to mimic the natural microenvironment of cells and promote differentiation. Furthermore, the development of methods to purify and characterize differentiated stem cells is essential for ensuring the quality and safety of stem cell therapies.
7.2 Gene Editing Technologies
Gene editing technologies, such as CRISPR-Cas9, offer the potential to correct genetic defects in stem cells and to enhance their therapeutic properties. Gene editing can be used to knock out genes that promote immune rejection, to insert genes that enhance cell survival and function, and to correct disease-causing mutations. However, the use of gene editing in stem cell therapy raises ethical concerns about the potential for off-target effects and the unintended consequences of altering the human genome.
7.3 Development of Biomaterials and Scaffolds
Biomaterials and scaffolds can be used to create a supportive microenvironment for transplanted stem cells, promoting their survival, differentiation, and integration into the host tissue. These materials can be designed to mimic the natural extracellular matrix and to release growth factors and other bioactive molecules. Furthermore, biomaterials can be used to deliver stem cells to specific locations in the body and to prevent their migration to unwanted sites.
7.4 Exosome-Based Therapy
Exosome-based therapy is a promising alternative to cell-based therapy, as it eliminates the need for cell transplantation and reduces the risk of immune rejection. The optimization of exosome production, purification, and delivery is essential for the successful translation of exosome-based therapy into clinical practice. Furthermore, the development of methods to enhance the therapeutic properties of exosomes, such as loading them with drugs or gene editing tools, is an active area of research.
7.5 Combination Therapies
Combining stem cell therapy with other therapeutic approaches, such as drug therapy, gene therapy, and immunotherapy, may enhance the therapeutic efficacy and overcome some of the limitations of stem cell therapy alone. For example, combining stem cell therapy with immunosuppressive drugs may reduce the risk of immune rejection, while combining stem cell therapy with growth factors may promote tissue regeneration. The optimal combination of therapies will depend on the specific disease being treated and the characteristics of the stem cells being used.
Many thanks to our sponsor Esdebe who helped us prepare this research report.
8. Conclusion
Stem cell therapy holds immense promise for treating a wide range of diseases, offering the potential to repair or replace damaged tissues and organs, and to modulate the immune system. While significant progress has been made in the field of stem cell research, several challenges remain in translating preclinical findings into safe and effective clinical applications. These challenges include the risk of immune rejection, the lack of long-term efficacy, the difficulty in achieving targeted differentiation, the risk of tumorigenicity, and the lack of standardized protocols and regulations. Ongoing research is focused on overcoming these challenges and expanding the therapeutic applications of stem cells. The ethical considerations surrounding stem cell research must be carefully addressed to ensure that stem cell therapies are developed and implemented in a responsible and ethical manner. With continued research and development, stem cell therapy has the potential to revolutionize medicine and improve the lives of millions of patients worldwide.
Many thanks to our sponsor Esdebe who helped us prepare this research report.
References
- Zakrzewski, W., Dobrzyński, M., Szymonowicz, M., Rybak, Z. (2019). Stem cells: definition, classification, and sources. World Journal of Stem Cells, 11(12), 883–905.
- Thomson, J. A., Itskovitz-Eldor, J., Shapiro, S. S., Waknitz, M. A., Swiergiel, J. J., Marshall, V. S., & Jones, J. M. (1998). Embryonic stem cell lines derived from human blastocysts. Science, 282(5391), 1145–1147.
- Takahashi, K., Tanabe, K., Ohnuki, M., Narita, M., Ichisaka, T., Tomoda, K., & Yamanaka, S. (2007). Induction of pluripotent stem cells from adult human fibroblasts by defined factors. Cell, 131(5), 861–872.
- Dominici, M., Le Blanc, K., Mueller, I., Slaper-Cortenbach, I., Marini, F., Krause, D., … & Horwitz, E. (2006). Minimal criteria for defining multipotent mesenchymal stromal cells. The International Society for Cellular Therapy position statement. Cytotherapy, 8(4), 315–317.
- Pittenger, M. F., Mackay, A. M., Beck, S. C., Jaiswal, R. K., Douglas, R., Mosca, J. D., … & Marshak, D. R. (1999). Multilineage potential of adult human mesenchymal stem cells. Science, 284(5411), 143–147.
- Caplan, A. I. (2017). Mesenchymal stem cells: time to change the name!. Stem Cells Translational Medicine, 6(6), 1445–1451.
- Luz-Crawford, P., Djouad, F., Noël, D., Jorgensen, C. (2015). Mesenchymal stem cells: cell therapy and immunomodulation. Frontiers in Immunology, 6, 68.
- Trounson, A., McDonald, C. (2015). Stem cell therapies in clinical trials: progress and challenges. Cell Stem Cell, 17(1), 11–22.
- Volkman, B. F., Offen, D. (2017). Mesenchymal stem cells in neurodegenerative diseases. Cellular and Molecular Neurobiology, 37(6), 899–912.
- Gnecchi, M., Melo, L. G. (2009). Mesenchymal stem cells: a tool for cardiac repair. Stem Cells, 27(5), 898–906.
- El Andaloussi, S., Mäger, I., Breakefield, X. O., Wood, M. J. A. (2013). Exosomes: biology and emerging therapeutic opportunities. Nature Reviews Drug Discovery, 12(5), 347–357.
- Cyranoski, D. (2016). Stem-cell trials surge, but efficacy lags. Nature, 537(7622), 462–463.
- Fuchs, E., Segre, J. A. (2000). Stem cells: a new lease on life. Cell, 100(1), 143–155.
- Daley, G. Q., Lensch, M. W., Jaenisch, R., Hanna, J. H. (2007). The promise of induced pluripotent stem cells in research and therapy. Cell Stem Cell, 1(1), 1–6.
- Lo, B., Parham, L. (2009). Ethical issues in stem cell research. Endocrine Reviews, 30(3), 204–213.
The report highlights the promise of MSCs in modulating the immune system. Could you elaborate on the specific mechanisms by which MSC-derived exosomes mediate immunomodulation and whether these mechanisms differ based on the source tissue of the MSCs?
Great question! While the report touches on the general role of MSC-derived exosomes in immunomodulation, the specifics of their mechanisms are indeed complex and an area of intense investigation. The cargo (miRNAs, proteins) within exosomes can vary based on MSC source tissue, potentially leading to nuanced immunomodulatory effects. More research is needed to fully understand these differences!
Editor: MedTechNews.Uk
Thank you to our Sponsor Esdebe
Ethical considerations, eh? So, if we could bypass all ethical speedbumps, what’s the wildest, most sci-fi application of stem cell therapy you’d greenlight immediately? Asking for a friend…who may or may not be a mad scientist.