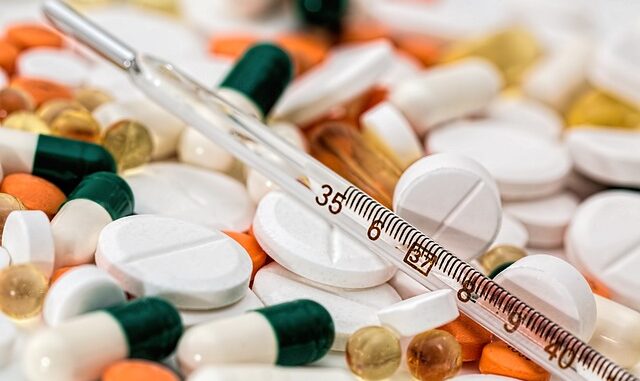
Abstract
Antibiotic resistance (AR) represents a paramount global health threat, eroding the efficacy of life-saving antimicrobial agents and jeopardizing advancements in modern medicine. This report provides a comprehensive exploration of the multifaceted nature of AR, moving beyond conventional discussions of bacterial mechanisms and clinical implications. We delve into the evolutionary dynamics driving AR, examining not only the molecular basis of resistance but also the ecological and selective pressures shaping its emergence and spread. A critical analysis of horizontal gene transfer (HGT) mechanisms, including plasmids, transposons, and bacteriophages, reveals their pivotal role in accelerating AR dissemination across diverse bacterial species and ecological niches. Furthermore, we investigate the intricate interplay of factors contributing to the AR crisis, spanning from antimicrobial usage in human and animal health to environmental contamination and the global interconnectedness facilitated by trade and travel. A significant portion of this report is dedicated to exploring innovative mitigation strategies, moving beyond traditional antibiotic development to encompass alternative therapies, improved diagnostics, enhanced surveillance systems, and comprehensive antimicrobial stewardship programs embedded within a One Health framework. Finally, we address the critical need for interdisciplinary collaboration and policy interventions to effectively combat the escalating threat of AR and safeguard the future of antimicrobial therapy.
Many thanks to our sponsor Esdebe who helped us prepare this research report.
1. Introduction
The discovery of antibiotics in the 20th century revolutionized medicine, offering unprecedented control over bacterial infections and significantly reducing morbidity and mortality. However, the widespread and often indiscriminate use of these ‘wonder drugs’ has inadvertently fueled the evolution and dissemination of antibiotic resistance (AR), transforming it into a global crisis threatening human and animal health, food security, and economic stability. AR arises from the inherent ability of bacteria to adapt and evolve under selective pressure, allowing them to develop mechanisms to evade or neutralize the effects of antibiotics. This evolutionary arms race is exacerbated by the remarkable capacity of bacteria to acquire and share resistance genes through horizontal gene transfer (HGT), enabling rapid dissemination of AR across diverse bacterial species and ecological niches. The consequences of AR are far-reaching, leading to increased treatment failures, prolonged hospital stays, higher healthcare costs, and elevated mortality rates. Moreover, AR complicates the management of common infections and undermines the efficacy of essential medical procedures such as surgery, organ transplantation, and cancer chemotherapy. The AR crisis necessitates a comprehensive and multi-faceted approach that integrates scientific advancements, public health interventions, and policy reforms. This report aims to provide an in-depth analysis of the evolutionary dynamics, dissemination mechanisms, contributing factors, consequences, and mitigation strategies associated with AR, emphasizing the need for a One Health perspective that acknowledges the interconnectedness of human, animal, and environmental health.
Many thanks to our sponsor Esdebe who helped us prepare this research report.
2. Evolutionary Mechanisms of Antibiotic Resistance
Antibiotic resistance is fundamentally an evolutionary phenomenon driven by natural selection. Bacteria, with their rapid reproduction rates and diverse genetic makeup, possess an inherent capacity to adapt to environmental stressors, including exposure to antibiotics. The evolutionary mechanisms underlying AR can be broadly categorized into two main types: intrinsic resistance and acquired resistance.
2.1. Intrinsic Resistance:
Intrinsic resistance refers to the inherent ability of certain bacterial species to resist the effects of antibiotics due to their natural structural or functional characteristics. This type of resistance is not acquired through genetic mutation or HGT, but rather is an inherent property of the organism. Examples of intrinsic resistance mechanisms include:
- Cell Wall Impermeability: The outer membrane of Gram-negative bacteria, such as Escherichia coli and Pseudomonas aeruginosa, acts as a permeability barrier, restricting the entry of many antibiotics. The presence of porins, channel-forming proteins in the outer membrane, allows for the passage of small hydrophilic molecules, but the size and charge selectivity of these porins can limit the access of larger or hydrophobic antibiotics. Mutations affecting porin expression or structure can further reduce antibiotic permeability, leading to resistance.
- Efflux Pumps: Efflux pumps are transmembrane proteins that actively transport antibiotics out of the bacterial cell, reducing their intracellular concentration and preventing them from reaching their target. Many bacteria possess multiple efflux pumps with broad substrate specificity, allowing them to extrude a wide range of antibiotics. Overexpression of efflux pumps, often due to mutations in regulatory genes, can significantly contribute to AR.
- Absence of Target: Some bacteria are intrinsically resistant to certain antibiotics because they lack the specific molecular target that the antibiotic inhibits. For example, Mycoplasma species lack a cell wall and are therefore intrinsically resistant to cell wall-targeting antibiotics such as penicillin and cephalosporins.
2.2. Acquired Resistance:
Acquired resistance arises when bacteria develop resistance mechanisms through genetic mutations or the acquisition of resistance genes via HGT. This type of resistance is typically acquired in response to antibiotic exposure and can spread rapidly among bacterial populations.
- Mutational Resistance: Mutations in bacterial genes encoding antibiotic targets can alter the structure of the target protein, reducing its affinity for the antibiotic and rendering it ineffective. For example, mutations in the genes encoding DNA gyrase and topoisomerase IV can lead to quinolone resistance, while mutations in the genes encoding ribosomal proteins can confer resistance to aminoglycosides and macrolides. The likelihood of mutational resistance depends on the mutation rate of the bacterial species, the size of the target gene, and the selective pressure exerted by antibiotic exposure.
- Horizontal Gene Transfer (HGT): HGT is a major driver of AR dissemination, allowing bacteria to acquire resistance genes from other bacteria, even those of different species or genera. The three main mechanisms of HGT are:
- Transformation: The uptake of free DNA from the environment by competent bacteria. This DNA may contain resistance genes that can be integrated into the recipient cell’s chromosome.
- Transduction: The transfer of DNA from one bacterium to another via bacteriophages (viruses that infect bacteria). Bacteriophages can package resistance genes into their viral particles and deliver them to new host cells.
- Conjugation: The transfer of DNA between bacteria through direct cell-to-cell contact via a plasmid. Plasmids are self-replicating extrachromosomal DNA molecules that can carry multiple resistance genes and spread rapidly among bacterial populations. Conjugation is considered the most efficient and widespread mechanism of HGT.
Many thanks to our sponsor Esdebe who helped us prepare this research report.
3. Horizontal Gene Transfer and the Mobilome
Horizontal gene transfer (HGT) is the primary engine driving the rapid dissemination of antibiotic resistance genes (ARGs) across diverse bacterial populations, transcending species and even genus boundaries. Understanding the mechanisms and dynamics of HGT is crucial for developing effective strategies to combat AR. The collective pool of mobile genetic elements (MGEs) – including plasmids, transposons, integrons, and bacteriophages – that facilitate HGT is often referred to as the ‘mobilome’. This section will explore the key MGEs involved in AR dissemination and their contribution to the global AR crisis.
3.1. Plasmids:
Plasmids are extrachromosomal, self-replicating DNA molecules that are commonly found in bacteria. They are arguably the most important vectors for HGT, particularly in the context of AR. Plasmids can carry multiple ARGs, often clustered together in ‘resistance islands’, which allow for the simultaneous transfer of resistance to multiple antibiotics. Plasmids replicate independently of the bacterial chromosome and can be transferred between bacteria via conjugation, a process that involves direct cell-to-cell contact facilitated by a pilus encoded by the plasmid. The efficiency of conjugation varies depending on the plasmid type, the host bacteria, and environmental conditions. Some plasmids are ‘promiscuous’, meaning they can transfer between a wide range of bacterial species, while others are more host-specific. The spread of ARGs via plasmids is a major concern, as it can lead to the emergence of multi-drug resistant (MDR) bacteria that are difficult or impossible to treat.
3.2. Transposons:
Transposons, also known as ‘jumping genes’, are mobile DNA sequences that can insert themselves into different locations within a bacterial genome or plasmid. They often carry ARGs and can facilitate the movement of these genes between different genetic elements. Transposons are flanked by inverted repeat sequences and encode a transposase enzyme that mediates their transposition. There are two main types of transposons: insertion sequences (ISs), which only encode the transposase, and composite transposons, which contain additional genes, such as ARGs, flanked by two ISs. Transposons can contribute to AR dissemination by inserting ARGs into plasmids, which can then be transferred to other bacteria via conjugation. They can also facilitate the spread of ARGs within a bacterial genome by moving them to different locations or creating new genetic arrangements.
3.3. Integrons:
Integrons are genetic elements that capture and express genes, typically ARGs, through a site-specific recombination system. They consist of an integrase gene ( intI), an attachment site (attI), and a promoter (Pc) that drives the expression of captured gene cassettes. Gene cassettes are small, circular DNA molecules that contain a single gene and an attC recombination site. Integrons capture gene cassettes by site-specific recombination between the attI site and the attC site, resulting in the insertion of the gene cassette into the integron. Integrons can accumulate multiple gene cassettes, leading to the expression of multiple ARGs. Integrons are often located on plasmids or transposons, facilitating their dissemination among bacteria. Class 1 integrons are the most prevalent and clinically relevant type of integron, and they are commonly associated with MDR in Gram-negative bacteria.
3.4. Bacteriophages:
Bacteriophages (phages) are viruses that infect bacteria. They can transfer DNA between bacteria via transduction, a process in which phage particles package bacterial DNA and deliver it to new host cells. There are two main types of transduction: generalized transduction, in which any bacterial gene can be transferred, and specialized transduction, in which only genes located near the phage integration site can be transferred. Phages can contribute to AR dissemination by transferring ARGs between bacteria via transduction. While transduction is generally considered less efficient than conjugation for the transfer of large plasmids, it can be an important mechanism for the spread of ARGs in certain environments, particularly in biofilms and other settings where bacteria are in close proximity.
3.5. The Role of the Mobilome in Shaping Bacterial Evolution:
The mobilome plays a crucial role in shaping bacterial evolution by facilitating the rapid adaptation of bacteria to new environments and selective pressures. HGT allows bacteria to acquire new genes and traits much faster than through vertical inheritance, enabling them to quickly develop resistance to antibiotics and other antimicrobial agents. The mobilome also contributes to bacterial diversity by creating new genetic combinations and facilitating the exchange of genes between different bacterial species. The dynamic interplay between different MGEs, such as plasmids, transposons, and integrons, allows for the rapid evolution and dissemination of AR, posing a significant challenge to public health.
Many thanks to our sponsor Esdebe who helped us prepare this research report.
4. Factors Contributing to the Spread of Antibiotic Resistance
The global spread of AR is a complex phenomenon driven by a confluence of factors, including antimicrobial usage, poor infection control practices, environmental contamination, and international travel and trade. Understanding these contributing factors is essential for developing effective intervention strategies.
4.1. Antimicrobial Usage:
Antimicrobial usage, particularly the overuse and misuse of antibiotics, is the primary driver of AR. When antibiotics are used, they exert selective pressure on bacterial populations, favoring the survival and proliferation of resistant bacteria. This selective pressure can lead to the enrichment of ARGs in bacterial communities and the emergence of MDR strains. Antimicrobial usage in human medicine, animal agriculture, and aquaculture all contribute to the AR crisis. In human medicine, antibiotics are often prescribed inappropriately for viral infections, such as colds and flu, where they are ineffective. In animal agriculture, antibiotics are widely used for growth promotion and prophylactic purposes, which can lead to the development of AR in animal-associated bacteria. These resistant bacteria can then be transmitted to humans through the food chain or direct contact with animals.
4.2. Poor Infection Control Practices:
Poor infection control practices in healthcare settings, such as hospitals and nursing homes, can facilitate the spread of AR bacteria between patients. Inadequate hand hygiene, improper use of personal protective equipment, and insufficient cleaning and disinfection of surfaces can all contribute to the transmission of resistant bacteria. The high density of patients in healthcare settings, combined with the frequent use of antibiotics, creates an ideal environment for the spread of AR. Outbreaks of MDR bacteria, such as methicillin-resistant Staphylococcus aureus (MRSA) and carbapenem-resistant Enterobacteriaceae (CRE), are a major concern in healthcare settings.
4.3. Environmental Contamination:
The environment plays a significant role in the dissemination of AR. Antibiotics and AR bacteria can enter the environment through various pathways, including wastewater treatment plants, agricultural runoff, and hospital effluents. These pollutants can contaminate soil, water, and sediments, creating reservoirs of ARGs that can persist for long periods of time. The presence of antibiotics in the environment can exert selective pressure on bacterial communities, promoting the development of AR. ARGs can also be transferred between bacteria in the environment via HGT, further contributing to the spread of AR. The use of manure and biosolids as fertilizers can also introduce AR bacteria and ARGs into agricultural soils, potentially leading to the contamination of crops and the food chain.
4.4. International Travel and Trade:
The increasing globalization of travel and trade has accelerated the spread of AR around the world. International travelers can acquire AR bacteria in foreign countries and introduce them to their home countries. The global trade of food and animals can also contribute to the dissemination of AR, as resistant bacteria can be transported across borders via contaminated products. The rapid spread of AR across continents highlights the need for international collaboration and coordinated efforts to combat this global threat.
4.5. Other Contributing Factors:
Other factors that contribute to the spread of AR include:
- Lack of access to clean water and sanitation: Poor sanitation can facilitate the spread of AR bacteria through contaminated water sources.
- Over-the-counter availability of antibiotics: The availability of antibiotics without a prescription in some countries contributes to their inappropriate use and the development of AR.
- Lack of awareness and education: Insufficient public awareness and education about AR can lead to inappropriate antibiotic use and poor infection control practices.
- Suboptimal diagnostic capabilities: The lack of rapid and accurate diagnostic tests for bacterial infections can lead to the overuse of broad-spectrum antibiotics.
Many thanks to our sponsor Esdebe who helped us prepare this research report.
5. Consequences of Antibiotic Resistance for Public Health
The escalating threat of AR poses significant and far-reaching consequences for public health, jeopardizing our ability to effectively treat bacterial infections and undermining advancements in modern medicine. The consequences of AR extend beyond increased morbidity and mortality to encompass economic burdens, healthcare system strains, and threats to global health security.
5.1. Increased Morbidity and Mortality:
AR leads to increased treatment failures, prolonged hospital stays, and higher mortality rates. Infections caused by AR bacteria are more difficult and costly to treat, often requiring the use of more toxic and expensive antibiotics. In some cases, infections caused by MDR bacteria are untreatable, leading to severe illness and death. The World Health Organization (WHO) estimates that AR infections cause at least 700,000 deaths globally each year, and this number is projected to rise to 10 million deaths per year by 2050 if no action is taken.
5.2. Economic Burden:
AR imposes a significant economic burden on healthcare systems and societies. The increased cost of treating AR infections includes the cost of more expensive antibiotics, longer hospital stays, and additional diagnostic tests. AR also leads to lost productivity due to illness and death, as well as increased healthcare costs for long-term care and disability. The World Bank estimates that AR could cost the global economy trillions of dollars per year by 2050.
5.3. Healthcare System Strain:
AR strains healthcare systems by increasing the demand for hospital beds, intensive care units, and specialized medical expertise. The spread of AR bacteria in hospitals and other healthcare settings can lead to outbreaks that disrupt services and require costly infection control measures. The need to isolate patients infected with AR bacteria also reduces the availability of hospital beds and increases the workload for healthcare workers. The emergence of untreatable infections poses a serious threat to the ability of healthcare systems to provide basic medical care.
5.4. Threats to Global Health Security:
AR poses a threat to global health security by undermining the effectiveness of antibiotics, which are essential for preventing and treating infectious diseases. The spread of AR across borders can lead to the emergence of untreatable epidemics and pandemics, posing a significant risk to global health. AR also threatens the ability to respond to bioterrorism events, as many biological weapons are susceptible to antibiotics. The lack of effective antibiotics could make it more difficult to contain and control outbreaks of infectious diseases, jeopardizing global health security.
5.5. Impact on Medical Procedures:
AR threatens the safety and efficacy of many medical procedures that rely on antibiotics to prevent and treat infections. These procedures include:
- Surgery: Antibiotics are routinely used to prevent infections after surgery. AR can increase the risk of surgical site infections and make them more difficult to treat.
- Organ transplantation: Immunosuppressant drugs are used to prevent organ rejection after transplantation, making transplant recipients more susceptible to infections. AR can increase the risk of infections in transplant recipients and make them more difficult to treat.
- Cancer chemotherapy: Chemotherapy weakens the immune system, making cancer patients more susceptible to infections. AR can increase the risk of infections in cancer patients and make them more difficult to treat.
- Treatment of premature infants: Premature infants have weak immune systems and are highly susceptible to infections. AR can increase the risk of infections in premature infants and make them more difficult to treat.
Many thanks to our sponsor Esdebe who helped us prepare this research report.
6. Strategies for Combating Antibiotic Resistance: A One Health Approach
Combating AR requires a comprehensive and multi-faceted approach that integrates scientific advancements, public health interventions, and policy reforms. A One Health approach, which recognizes the interconnectedness of human, animal, and environmental health, is essential for effectively addressing the AR crisis. This section will explore various strategies for combating AR, including the development of new antibiotics, improved antibiotic stewardship, alternative therapies, enhanced surveillance systems, and policy interventions.
6.1. Developing New Antibiotics:
The development of new antibiotics is crucial for replenishing the dwindling arsenal of effective antimicrobial agents. However, the discovery and development of new antibiotics has slowed down significantly in recent decades due to scientific, economic, and regulatory challenges. To stimulate antibiotic development, various initiatives have been launched, including government funding programs, public-private partnerships, and regulatory incentives. Novel approaches to antibiotic discovery include:
- Exploring unconventional sources: Screening natural products from unexplored environments, such as marine sediments and deep-sea hydrothermal vents, for novel antimicrobial compounds.
- Developing new targets: Identifying new bacterial targets that are essential for survival and are less prone to resistance development.
- Designing antibiotics that overcome resistance mechanisms: Developing antibiotics that can evade or overcome existing resistance mechanisms, such as efflux pumps and target mutations.
- Using synthetic biology: Designing and synthesizing novel antimicrobial compounds with specific properties and mechanisms of action.
6.2. Improving Antibiotic Stewardship:
Antibiotic stewardship programs aim to optimize antibiotic use in human and animal health to reduce the selective pressure for AR. These programs typically involve:
- Promoting appropriate antibiotic prescribing: Educating healthcare professionals about the appropriate use of antibiotics and providing guidelines for the treatment of common infections.
- Implementing diagnostic testing: Using rapid and accurate diagnostic tests to identify bacterial infections and guide antibiotic selection.
- Restricting the use of broad-spectrum antibiotics: Limiting the use of broad-spectrum antibiotics to situations where they are clearly indicated.
- Implementing antibiotic cycling: Rotating the use of different antibiotics to reduce the selective pressure for resistance to any one drug.
- Monitoring antibiotic use and resistance patterns: Tracking antibiotic use and resistance patterns to identify areas where stewardship efforts can be improved.
6.3. Exploring Alternative Therapies:
Alternative therapies, such as phage therapy, antimicrobial peptides, and probiotics, offer potential solutions for treating bacterial infections without relying on traditional antibiotics. These therapies can be used alone or in combination with antibiotics to enhance their efficacy and reduce the development of AR. Specifically:
- Phage Therapy: Utilizing bacteriophages (viruses that infect bacteria) to target and kill specific bacterial pathogens. Phage therapy has shown promise in treating infections caused by MDR bacteria, but it requires careful selection of phages and monitoring for the development of phage resistance.
- Antimicrobial Peptides (AMPs): Employing naturally occurring peptides with antimicrobial activity to disrupt bacterial membranes or intracellular processes. AMPs have broad-spectrum activity and are less prone to resistance development than traditional antibiotics.
- Probiotics: Administering beneficial bacteria to restore the balance of the gut microbiota and prevent the colonization of pathogenic bacteria. Probiotics can be used to prevent antibiotic-associated diarrhea and other complications of antibiotic therapy.
- Immunotherapies: Harnessing the power of the immune system to fight bacterial infections. Immunotherapies, such as monoclonal antibodies and vaccines, can enhance the immune response to bacteria and prevent or treat infections.
6.4. Enhancing Surveillance Systems:
Enhanced surveillance systems are essential for tracking the spread of AR and identifying emerging resistance threats. These systems should include:
- Monitoring antibiotic use and resistance patterns: Collecting data on antibiotic use and resistance patterns in human and animal populations.
- Identifying outbreaks of AR bacteria: Investigating and controlling outbreaks of AR bacteria in healthcare settings and the community.
- Tracking the spread of ARGs: Monitoring the prevalence and distribution of ARGs in the environment.
- Developing rapid diagnostic tests: Creating rapid and accurate diagnostic tests to identify bacterial infections and detect AR genes.
6.5. Implementing Policy Interventions:
Policy interventions are needed to promote the rational use of antibiotics and prevent the spread of AR. These interventions should include:
- Regulating the use of antibiotics in human and animal health: Implementing policies to restrict the inappropriate use of antibiotics in human medicine and animal agriculture.
- Improving infection control practices: Promoting and enforcing infection control practices in healthcare settings and the community.
- Investing in research and development: Funding research and development of new antibiotics, alternative therapies, and diagnostic tests.
- Promoting public awareness and education: Raising public awareness about AR and promoting responsible antibiotic use.
- Strengthening international collaboration: Collaborating with other countries to address the global threat of AR.
Many thanks to our sponsor Esdebe who helped us prepare this research report.
7. Conclusion
Antibiotic resistance is a complex and multifaceted problem that demands a comprehensive and coordinated global response. The evolutionary adaptability of bacteria, coupled with the selective pressures imposed by antibiotic overuse, has fueled the emergence and spread of AR at an alarming rate. The consequences of AR are dire, threatening to undermine the efficacy of modern medicine and jeopardize global health security. Combating AR requires a multi-pronged approach that includes the development of new antibiotics, improved antibiotic stewardship, the exploration of alternative therapies, enhanced surveillance systems, and robust policy interventions. A One Health perspective, which recognizes the interconnectedness of human, animal, and environmental health, is essential for effectively addressing the AR crisis. Concerted efforts at the individual, national, and international levels are crucial to mitigate the threat of AR and safeguard the future of antimicrobial therapy. Continued research, innovation, and collaboration are paramount to staying ahead in the adaptive arms race against antibiotic-resistant bacteria.
Many thanks to our sponsor Esdebe who helped us prepare this research report.
References
- Alekshun, M. N., & Levy, S. B. (2007). Antimicrobial resistance: mechanisms of resistance and overview of resistance mechanisms. Cell, 128(6), 1037-1050.
- Aminov, R. I. (2010). Horizontal gene exchange in environmental microbiota. Frontiers in Microbiology, 1, 4.
- Boucher, H. W., Talbot, G. H., Bradley, J. S., Edwards, J. E., Gilbert, D., Rice, L. B., … & Spellberg, B. (2009). Bad bugs, no drugs: no ESKAPE! An update from the Infectious Diseases Society of America. Clinical Infectious Diseases, 48(1), 1-12.
- Carlet, J., Collignon, P., Goldmann, D., Graham, I. D., Harbarth, S., Jarlier, V., … & Pittet, D. (2012). Society’s failure to protect a precious resource: antibiotics. The Lancet, 379(9814), 385-386.
- Davies, J., & Davies, D. (2010). Origins and evolution of antibiotic resistance. Microbiology and Molecular Biology Reviews, 74(3), 417-433.
- Fair, R. J., & Tor, Y. (2014). Antibiotics and bacterial resistance in the 21st century. Perspectives in Medicinal Chemistry, 6, PMC-S14459.
- Hernando-Amado, S., Martínez, J. L., & Arraiza, M. D. (2016). Antibiotic resistance: from environment to man. Environmental Microbiology, 18(12), 3771-3792.
- Levy, S. B., & Marshall, B. (2004). Antibacterial resistance worldwide: causes, challenges and responses. Nature Medicine, 10(12 Suppl), S122-S129.
- Livermore, D. M. (2000). Antibiotic resistance in staphylococci. International Journal of Antimicrobial Agents, 16 Suppl 1, S3-S10.
- Read, A. F., Day, T., & Huijben, S. (2011). The evolution of drug resistance and the curious orthodoxy of aggressive chemotherapy. Proceedings of the National Academy of Sciences, 108 Suppl 2, 10871-10878.
- World Health Organization. (2014). Antimicrobial resistance: global report on surveillance 2014. World Health Organization.
- World Health Organization. (2015). Global action plan on antimicrobial resistance. World Health Organization.
Wow, that’s a bacterial deep dive! Suddenly, my sourdough starter feels way more threatening. Anyone else looking at their yogurt in a whole new light after this? Perhaps we should all invest in hazmat suits… or just wash our hands more?
That’s a great point about everyday microbes! It’s fascinating how even beneficial bacteria can harbor resistance genes. While hazmat suits might be a *bit* extreme, understanding microbial ecosystems and practicing good hygiene is definitely key to minimizing the spread of resistance. Thanks for sparking that thought!
Editor: MedTechNews.Uk
Thank you to our Sponsor Esdebe
Wow, diving into horizontal gene transfer! Makes sharing a Netflix password seem downright archaic. Suddenly I’m side-eyeing my co-workers, wondering what plasmids they might be conjugating. Maybe mandatory microbial dating profiles are the answer?
That’s a hilarious and insightful take! The comparison to sharing a Netflix password really puts the speed and efficiency of horizontal gene transfer into perspective. Microbial dating profiles – now there’s an idea that could revolutionize how we understand bacterial interactions! Thanks for the chuckle and the thought-provoking perspective.
Editor: MedTechNews.Uk
Thank you to our Sponsor Esdebe