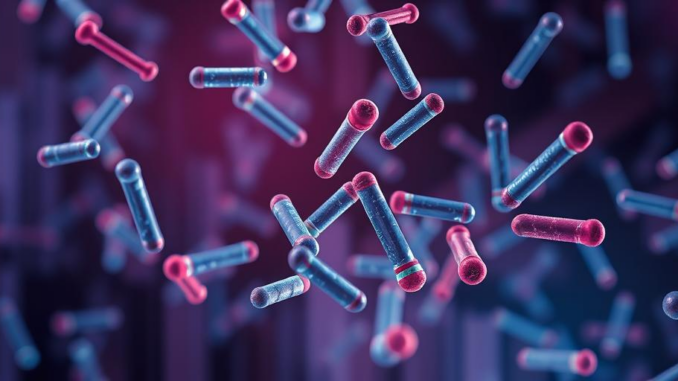
Abstract
Antibodies, also known as immunoglobulins, are central players in the adaptive immune response, providing humoral immunity against a vast array of pathogens and aberrant self-antigens. This report provides a comprehensive overview of antibody biology, encompassing their structure, function, diversity generation, and regulatory mechanisms. Furthermore, it explores the burgeoning field of antibody profiling, discussing both established and emerging technologies for high-throughput antibody repertoire analysis. We delve into the applications of antibody profiling across diverse domains, including infectious disease, autoimmunity, cancer, and allergy, emphasizing the potential of antibodies as biomarkers for disease prediction, diagnosis, and therapeutic monitoring. Crucially, we address the challenges associated with interpreting complex antibody profiles and highlight the advancements in bioinformatics and machine learning that are facilitating the translation of these data into clinically relevant insights. Finally, we discuss the rapidly evolving landscape of antibody-based therapeutics, focusing on novel strategies for antibody engineering and delivery to enhance efficacy and overcome resistance.
Many thanks to our sponsor Esdebe who helped us prepare this research report.
1. Introduction
The antibody, a cornerstone of the adaptive immune system, represents a remarkable example of biological specificity and adaptability. From its initial discovery as a mediator of humoral immunity to its current role as a versatile tool in diagnostics and therapeutics, the study of antibodies has profoundly shaped our understanding of biology and medicine. Antibodies are glycoproteins produced by B lymphocytes in response to antigenic stimulation. Their primary function is to recognize and bind to specific antigens, thereby neutralizing pathogens, opsonizing targets for phagocytosis, or activating the complement cascade. The immense diversity of the antibody repertoire, estimated to exceed 10^18 different specificities, enables the immune system to respond to virtually any foreign substance.
Beyond their protective role in immunity, antibodies have emerged as invaluable biomarkers for a wide range of diseases. Aberrant antibody profiles, characterized by the presence of autoantibodies or altered levels of antigen-specific antibodies, can provide early indications of disease onset, predict disease progression, and monitor treatment response. Furthermore, the ability to engineer antibodies with exquisite specificity and affinity has revolutionized therapeutic intervention, leading to the development of numerous antibody-based drugs for cancer, autoimmune diseases, and infectious diseases.
This report aims to provide a comprehensive overview of the field of antibody research, from the fundamental principles of antibody biology to the cutting-edge technologies for antibody profiling and the clinical applications of antibody-based therapies. We will explore the intricacies of antibody structure, function, and diversity generation, and discuss the methods used to analyze and interpret antibody repertoires. We will also address the challenges and opportunities associated with translating antibody profiling data into clinically meaningful insights and highlight the emerging trends in antibody engineering and therapeutics.
Many thanks to our sponsor Esdebe who helped us prepare this research report.
2. Antibody Structure and Function
An antibody molecule typically consists of two identical heavy chains and two identical light chains, arranged in a Y-shaped structure. Each chain contains a variable region (V region) and a constant region (C region). The V regions, located at the tips of the Y, are responsible for antigen binding, while the C regions mediate effector functions such as complement activation and Fc receptor binding.
The V regions are highly diverse due to somatic recombination of gene segments, the addition of non-templated nucleotides at the junctions of these segments (junctional diversity), and somatic hypermutation. Somatic recombination occurs during B cell development in the bone marrow, where V, D (diversity), and J (joining) gene segments are rearranged to form a unique V region sequence in the heavy chain, and V and J gene segments are rearranged to form a unique V region sequence in the light chain. Junctional diversity, mediated by the enzyme terminal deoxynucleotidyl transferase (TdT), introduces additional variability at the junctions of the V, D, and J segments. Somatic hypermutation, which occurs in germinal center B cells after antigen exposure, introduces point mutations into the V regions, further diversifying the antibody repertoire. B cells with higher affinity for antigen are positively selected and differentiate into plasma cells, which secrete large amounts of antibodies, or memory B cells, which provide long-lasting immunity.
Antibodies exert their functions through various mechanisms. Neutralization involves blocking the ability of a pathogen or toxin to bind to its target cell. Opsonization enhances phagocytosis by coating pathogens with antibodies, making them more readily recognized by phagocytic cells expressing Fc receptors. Complement activation triggers a cascade of enzymatic reactions that lead to pathogen lysis and inflammation. Antibody-dependent cell-mediated cytotoxicity (ADCC) involves the recruitment of cytotoxic cells, such as natural killer (NK) cells, to antibody-coated target cells, resulting in target cell destruction. The specific effector function elicited by an antibody depends on its isotype (IgG, IgM, IgA, IgE, IgD), which is determined by the C region of the heavy chain. Each isotype has unique properties and distribution in the body, allowing the immune system to tailor its response to different types of threats.
Many thanks to our sponsor Esdebe who helped us prepare this research report.
3. Antibody Repertoire Profiling Technologies
Antibody repertoire profiling aims to comprehensively characterize the diversity and abundance of antibodies present in a biological sample. Several technologies have been developed for this purpose, each with its own advantages and limitations.
3.1. Hybridoma Technology
Hybridoma technology, developed by Köhler and Milstein in 1975, revolutionized antibody research by enabling the production of monoclonal antibodies (mAbs). This technique involves fusing antibody-producing B cells from an immunized animal with immortal myeloma cells, creating hybridomas that can continuously produce mAbs with a defined specificity. Hybridoma technology has been instrumental in the development of numerous diagnostic and therapeutic antibodies. However, it is limited by the need for animal immunization and the laborious process of hybridoma screening and selection.
3.2. Phage Display
Phage display is a powerful technique for isolating antibodies with desired specificities from a large library of antibody fragments displayed on the surface of bacteriophages. Antibody libraries can be generated from immunized or unimmunized animals or from synthetic sequences. Phages displaying antibodies that bind to a target antigen are enriched through repeated rounds of binding, washing, and elution. Phage display allows for the selection of antibodies with high affinity and specificity without the need for animal immunization. It has also been used to generate antibodies against targets that are difficult to immunize against, such as self-antigens. However, phage display can be biased towards certain antibody sequences and may not fully represent the in vivo antibody repertoire.
3.3. B Cell Cloning and Sequencing
B cell cloning and sequencing involves isolating individual B cells from a biological sample and amplifying and sequencing the antibody genes from each cell. This approach provides a comprehensive view of the antibody repertoire at the single-cell level. B cell cloning and sequencing can be used to identify antibodies that are specific for a particular antigen, to track the evolution of antibody responses over time, and to analyze the diversity of the antibody repertoire in different individuals or disease states. High-throughput sequencing technologies have significantly increased the throughput of B cell cloning and sequencing, enabling the analysis of millions of B cells in a single experiment. However, this method can be technically challenging and requires specialized equipment and expertise.
3.4. Mass Spectrometry
Mass spectrometry (MS) is an analytical technique that measures the mass-to-charge ratio of ions. MS can be used to identify and quantify antibodies based on their amino acid sequence. Peptide sequencing of antibody fragments generated by enzymatic digestion can provide information on the variable region sequences and post-translational modifications of antibodies. MS-based antibody profiling can be used to analyze complex antibody mixtures, such as serum or plasma, and to identify novel antibody biomarkers. However, MS-based antibody profiling can be challenging due to the complexity of the antibody repertoire and the need for sophisticated data analysis techniques.
3.5. PhIP-Seq (Phage Immunoprecipitation Sequencing)
PhIP-Seq is a high-throughput technology that combines phage display with next-generation sequencing to identify and quantify antibodies that bind to a library of peptides displayed on bacteriophages. The library can consist of random peptides, overlapping peptides from a protein of interest, or peptides representing known antigens. Serum or plasma samples are incubated with the phage library, and antibodies that bind to the peptides are captured by immunoprecipitation. The DNA encoding the captured peptides is then amplified and sequenced. PhIP-Seq provides a comprehensive view of the antibody specificities in a sample and can be used to identify autoantibodies and antibodies against infectious agents. This technology is particularly powerful for discovering novel biomarkers and understanding the role of antibodies in disease pathogenesis. It has been used to study various autoimmune diseases, infectious diseases, and cancers.
3.6. High-Density Peptide Arrays
High-density peptide arrays offer another approach for high-throughput antibody profiling. These arrays consist of thousands or even millions of different peptides synthesized directly onto a solid support. Serum or plasma samples are incubated with the array, and antibodies that bind to the peptides are detected using fluorescently labeled secondary antibodies. High-density peptide arrays can be used to identify antibodies that bind to specific epitopes and to map the antibody binding landscape of a complex protein. They are also useful for identifying cross-reactive antibodies and for studying the specificity of antibody-drug interactions.
Many thanks to our sponsor Esdebe who helped us prepare this research report.
4. Applications of Antibody Profiling
Antibody profiling has a wide range of applications in basic research, diagnostics, and therapeutics.
4.1. Infectious Disease
Antibody profiling can be used to track the evolution of antibody responses during infection, to identify protective antibodies, and to develop vaccines and therapeutics. For example, antibody profiling has been used to study the antibody response to HIV, influenza virus, and SARS-CoV-2. Identifying broadly neutralizing antibodies against these viruses is a major goal of vaccine development. Antibody profiling can also be used to diagnose infections by detecting antibodies against specific pathogens.
4.2. Autoimmune Disease
Autoantibodies are antibodies that target self-antigens and are a hallmark of autoimmune diseases. Antibody profiling can be used to identify novel autoantibodies, to understand the pathogenesis of autoimmune diseases, and to develop diagnostic and therapeutic tools. For example, antibody profiling has been used to identify autoantibodies in rheumatoid arthritis, systemic lupus erythematosus, and type 1 diabetes. In the context of IBD, antibody profiling is particularly useful for differentiating between ulcerative colitis (UC) and Crohn’s disease (CD), as well as for predicting disease severity and response to therapy. Specific antibodies such as ASCA (anti-Saccharomyces cerevisiae antibodies), pANCA (perinuclear anti-neutrophil cytoplasmic antibodies), and antibodies against bacterial flagellin (anti-CBir1) have been shown to correlate with different IBD subtypes and disease outcomes.
4.3. Cancer
Antibody profiling can be used to identify tumor-associated antigens, to track the antibody response to cancer, and to develop antibody-based therapies. For example, antibody profiling has been used to identify antibodies that bind to cancer-specific mutations or to proteins that are overexpressed in cancer cells. These antibodies can be used as biomarkers for cancer diagnosis and prognosis and as therapeutic agents to target cancer cells. Furthermore, the development of immune checkpoint inhibitors has highlighted the importance of understanding the antibody response to tumors and the potential for modulating the immune system to fight cancer.
4.4. Allergy
IgE antibodies play a central role in allergic reactions. Antibody profiling can be used to identify allergens and to understand the mechanisms of allergic sensitization. For example, antibody profiling has been used to identify IgE antibodies that bind to food allergens, pollen allergens, and insect venom allergens. Understanding the IgE antibody repertoire in allergic individuals can help to develop more effective diagnostic and therapeutic strategies for allergic diseases.
4.5 Biomarker Discovery
One of the most promising applications of antibody profiling is the discovery of novel biomarkers for disease prediction, diagnosis, and prognosis. By analyzing antibody profiles in large cohorts of patients, researchers can identify antibodies that are associated with specific disease states or outcomes. These antibodies can then be used to develop diagnostic tests or to stratify patients for clinical trials. The identification of antibody biomarkers requires careful validation and standardization to ensure that the results are reproducible and clinically relevant. This involves rigorous statistical analysis, consideration of confounding factors, and validation in independent patient cohorts.
Many thanks to our sponsor Esdebe who helped us prepare this research report.
5. Challenges and Future Directions
Despite the significant advances in antibody profiling technologies, several challenges remain.
5.1. Data Complexity
The antibody repertoire is incredibly complex, and analyzing and interpreting antibody profiling data can be challenging. The sheer volume of data generated by high-throughput sequencing and peptide array technologies requires sophisticated bioinformatics tools and expertise. Furthermore, the diversity of the antibody repertoire means that many antibodies are present at very low levels, making them difficult to detect and quantify. Overcoming this challenge requires improved data analysis algorithms, better methods for normalizing and comparing antibody profiles, and the development of databases and resources for sharing antibody profiling data.
5.2. Technical Variability
Technical variability in antibody profiling assays can also be a challenge. Factors such as sample preparation, assay conditions, and data analysis pipelines can all affect the results. To minimize technical variability, it is important to standardize antibody profiling assays and to use appropriate controls. Furthermore, it is important to validate antibody profiling results using orthogonal methods, such as ELISA or Western blot.
5.3. Biological Complexity
The biological complexity of the antibody response can also make it difficult to interpret antibody profiling data. The antibody response is influenced by a variety of factors, including genetics, environment, and previous exposures. These factors can affect the antibody repertoire and can make it difficult to identify antibodies that are specifically associated with a particular disease. To address this challenge, it is important to consider the clinical and demographic characteristics of the study population when analyzing antibody profiling data. Furthermore, it is important to perform longitudinal studies to track the evolution of the antibody response over time.
5.4. Translation to Clinical Applications
Translating antibody profiling data into clinical applications requires careful validation and standardization. Antibody biomarkers must be rigorously validated in independent patient cohorts and must be shown to be clinically useful. Furthermore, diagnostic tests based on antibody profiling must be affordable and accessible to patients. Overcoming these challenges requires collaboration between researchers, clinicians, and industry partners. It also requires investment in the development of new technologies and infrastructure for antibody profiling.
5.5. Advancements in Bioinformatics and Machine Learning
The interpretation of complex antibody profiles heavily relies on advancements in bioinformatics and machine learning. These tools are essential for processing large datasets, identifying patterns, and predicting disease outcomes. Machine learning algorithms can be trained to distinguish between different disease states based on antibody profiles, even when the differences are subtle or complex. Furthermore, bioinformatics tools can be used to annotate antibody sequences, predict their binding specificities, and identify potential cross-reactivities. The integration of these tools into antibody profiling workflows is essential for maximizing the clinical utility of antibody data.
5.6. Future Directions
Future directions in antibody profiling include the development of more sensitive and specific assays, the integration of antibody profiling with other omics technologies, and the use of antibody profiling to guide personalized medicine. For example, combining antibody profiling with genomics, transcriptomics, and proteomics data can provide a more comprehensive view of the immune system and can help to identify novel therapeutic targets. Furthermore, antibody profiling can be used to predict which patients are most likely to respond to a particular therapy, allowing for more personalized treatment decisions.
The integration of multi-omics data with antibody profiling holds immense promise for unraveling the complex interplay between the immune system and disease. By combining antibody profiles with genomic, transcriptomic, and proteomic data, researchers can gain a more holistic understanding of disease mechanisms and identify novel therapeutic targets. For example, in cancer, integrating antibody profiles with tumor genomic data can reveal tumor-specific antigens and predict response to immunotherapy. Similarly, in autoimmune diseases, combining antibody profiles with transcriptomic data from immune cells can identify dysregulated pathways and inform the development of targeted therapies.
Many thanks to our sponsor Esdebe who helped us prepare this research report.
6. Antibody-Based Therapies
The ability to engineer antibodies with exquisite specificity and affinity has revolutionized therapeutic intervention. Antibody-based therapies are now used to treat a wide range of diseases, including cancer, autoimmune diseases, and infectious diseases.
6.1. Monoclonal Antibodies
Monoclonal antibodies (mAbs) are antibodies that are produced by a single clone of B cells and that bind to a single epitope. mAbs can be produced using hybridoma technology or phage display. mAbs have been used to treat a variety of diseases, including cancer, autoimmune diseases, and infectious diseases. For example, the anti-HER2 antibody trastuzumab (Herceptin) is used to treat breast cancer, the anti-TNF-alpha antibody infliximab (Remicade) is used to treat rheumatoid arthritis, and the anti-CD20 antibody rituximab (Rituxan) is used to treat non-Hodgkin lymphoma.
6.2. Antibody-Drug Conjugates
Antibody-drug conjugates (ADCs) are antibodies that are conjugated to a cytotoxic drug. ADCs are designed to selectively deliver cytotoxic drugs to cancer cells, minimizing the toxicity to normal cells. ADCs have been used to treat a variety of cancers, including breast cancer, lymphoma, and leukemia. For example, the anti-HER2 ADC trastuzumab emtansine (Kadcyla) is used to treat HER2-positive breast cancer, and the anti-CD30 ADC brentuximab vedotin (Adcetris) is used to treat Hodgkin lymphoma.
6.3. Bispecific Antibodies
Bispecific antibodies (BsAbs) are antibodies that bind to two different antigens. BsAbs can be used to simultaneously target two different molecules or cells, enhancing their therapeutic efficacy. For example, a BsAb that binds to both a tumor-associated antigen and a T cell receptor can recruit T cells to the tumor, resulting in tumor cell killing. Blinatumomab is a bispecific T-cell engager (BiTE) antibody that binds to CD19 on B cells and CD3 on T cells. It is approved for the treatment of relapsed or refractory B-cell acute lymphoblastic leukemia (ALL).
6.4. Antibody Engineering
Antibody engineering involves modifying the amino acid sequence of an antibody to improve its properties, such as affinity, specificity, and stability. Antibody engineering can be used to create antibodies with novel functions or to optimize existing antibodies for therapeutic use. For example, antibody engineering has been used to humanize mouse antibodies, to increase the affinity of antibodies for their targets, and to create antibodies that are more resistant to degradation.
6.5. Novel Delivery Strategies
The delivery of antibody-based therapies can be challenging, particularly for solid tumors. Novel delivery strategies, such as nanoparticles and exosomes, are being developed to improve the delivery of antibodies to target tissues. Nanoparticles can be used to encapsulate antibodies and to protect them from degradation in the bloodstream. Exosomes, which are small vesicles secreted by cells, can be used to deliver antibodies to target cells in a highly specific manner. The optimization of antibody delivery is crucial for maximizing therapeutic efficacy and minimizing off-target effects.
Many thanks to our sponsor Esdebe who helped us prepare this research report.
7. Conclusion
Antibodies are essential components of the adaptive immune system and play a critical role in protecting against pathogens and maintaining immune homeostasis. Antibody profiling technologies have revolutionized our understanding of antibody responses in health and disease and have opened up new avenues for biomarker discovery and therapeutic development. While challenges remain in interpreting complex antibody profiles and translating these data into clinical applications, ongoing advancements in bioinformatics, machine learning, and antibody engineering hold immense promise for the future of antibody research. Antibody-based therapies have already transformed the treatment of many diseases, and the development of novel antibody formats and delivery strategies will undoubtedly lead to further breakthroughs in the years to come. Understanding the full potential of antibody profiling requires a multidisciplinary approach, integrating expertise from immunology, molecular biology, bioinformatics, and clinical medicine.
Many thanks to our sponsor Esdebe who helped us prepare this research report.
References
- Janeway CA Jr, Travers P, Walport M, et al. Immunobiology: The Immune System in Health and Disease. 5th edition. New York: Garland Science; 2001.
- Köhler G, Milstein C. Continuous cultures of fused cells secreting antibody of predefined specificity. Nature. 1975 Aug 7;256(5517):495-7.
- Smith GP. Filamentous fusion phage: novel expression vectors that display cloned antigens on the virion surface. Science. 1985 Jun 7;228(4705):1315-7.
- Lavinder JJ, Wine Y, Giesecke C, Ippolito GC, Ellefsen KM, Moulder T, Crowe JE Jr, Georgiou G. Identification and characterization of broadly neutralizing antibodies from individuals infected with the H1N1 influenza virus. J Virol. 2011 Feb;85(4):1635-47.
- Lichtman MK, Meng W, Gettie A, Haigwood NL, Overbaugh J, Stamatatos L. Broadly neutralizing antibodies to HIV-1 develop more frequently in subtype C-infected individuals than in subtype B-infected individuals. J Virol. 2012 Apr;86(8):4405-17.
- Larman HB, Quake SR. Massively parallel determination of antibody-antigen specificities. Elife. 2015 Apr 21;4:e05251.
- Kirkpatrick J, DeKosky BJ. High-throughput antibody repertoire sequencing. Curr Opin Chem Biol. 2021 Jun;62:1-10.
- Buss NA, Henderson SJ. Antibody therapeutics: success, potential and challenges. Trends Immunol. 2021 Jun;42(6):477-491.
- Chan AC, Carter PJ. Therapeutic antibodies for autoimmunity and inflammation. Nat Rev Immunol. 2010 May;10(5):301-16.
- Weiner GJ. Monoclonal antibody therapy of cancer. Annu Rev Med. 2015;66:291-304.
- Reichert JM. Antibody-drug conjugates: development trends. Expert Opin Drug Discov. 2013 Oct;8(10):1307-16.
- Labrijn AF, Janmaat ML, Reichert JM, de Goeij BE. Bispecific antibodies: a mechanistic perspective. Nat Rev Immunol. 2019 Aug;19(8):471-483.
- Nelson AL. Antibody engineering. Chem Soc Rev. 2010 Jun;39(6):2176-84.
- Sahlender DA, Portolano N, Weile J, Dearlove B, Lunter G, Sattar N, Vyse TJ, Cutler AJ, Deane CM, Somers O. Defining specificity landscapes of antibodies using high-density peptide arrays. Nat Commun. 2019 Oct 23;10(1):4825.
- Pons J, Nomura Y, Watanabe K, et al. Antigen specificity of antibodies to SARS-CoV-2 variants in patients with mild or severe COVID-19. Nat Commun. 2021;12(1):5250. doi:10.1038/s41467-021-25346-2
- He B, Bielekova B. Autoantibody profiling in autoimmune diseases. Autoimmun Rev. 2014;13(4-5):476-483. doi:10.1016/j.autrev.2013.11.007
- Clemente-Casares X, Younes SA, Allegretta M, Santamaria P. Antigen array technology for autoantibody profiling in type 1 diabetes. Methods Mol Biol. 2011;674:193-204. doi: 10.1007/978-1-60761-844-4_13.
- Sokolowska M, Hofmeier KS, Lauener R, Asam C, Bohle B, Untersmayr E. Allergen-specific IgE detection with recombinant allergens and peptide microarrays. Methods. 2013;60(3):268-276. doi:10.1016/j.ymeth.2013.01.009
- Deng J, Li B, Li Z, et al. High-throughput analysis of antibody repertoires using emulsion PCR and next-generation sequencing. BMC Immunol. 2016;17(1):52. Published 2016 Nov 8. doi:10.1186/s12865-016-0166-4
- Benitez-Ribas D, Cabezón E, Keam SP, et al. Bispecific antibodies for cancer immunotherapy. Expert Opin Biol Ther. 2015;15(9):1355-1365. doi:10.1517/14712598.2015.1062319
So, if our antibodies are constantly battling unseen foes, does that mean we’re all just extras in a microscopic, never-ending action movie? And where do I sign up for craft services?
That’s a fantastic analogy! It really highlights the constant activity within our bodies. Thinking of it as a microscopic action movie, it’s fascinating to consider how personalized these antibody responses can be. Every individual has their own unique ‘movie’ playing out. It raises the question, what are the blockbuster hits and hidden indie gems of our immune system?
Editor: MedTechNews.Uk
Thank you to our Sponsor Esdebe
Given the challenges of interpreting antibody profiles due to biological complexity, how might personalized patient history data, integrated with these profiles, improve the precision of disease prediction or therapeutic response?
That’s a great question! Integrating personalized patient history data with antibody profiles could indeed enhance precision. By considering factors like past infections, lifestyle, and genetics, we can create a more complete picture. This holistic approach could help us differentiate between protective and pathogenic antibody responses, leading to more accurate disease predictions and tailored treatment strategies.
Editor: MedTechNews.Uk
Thank you to our Sponsor Esdebe
Antibody profiling sounds like my kind of party! So many potential biomarkers, it’s like panning for diagnostic gold. But with data that complex, do we need a decoder ring… or just a really, really big spreadsheet?
That’s a great way of putting it! It’s true, the volume of data is huge, and a really, really big spreadsheet might be a starting point! However, we are increasingly reliant on sophisticated bioinformatics and machine learning tools to make sense of it all and identify patterns. The decoder ring is getting smarter!
Editor: MedTechNews.Uk
Thank you to our Sponsor Esdebe