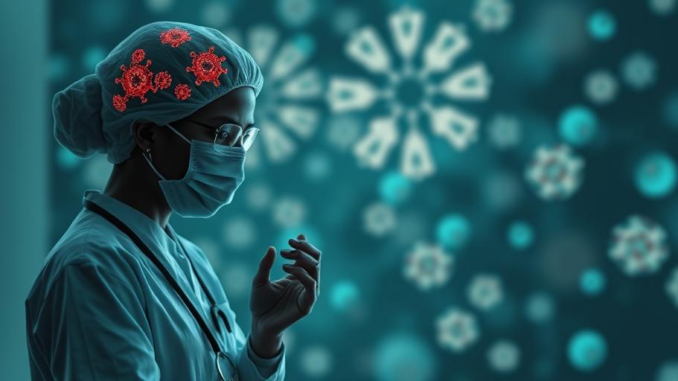
Abstract
Cancer therapy is undergoing a paradigm shift, moving beyond conventional cytotoxic approaches to more targeted and personalized strategies. This review examines the latest advancements in cancer therapy, focusing on areas showing significant promise and highlighting their current limitations. We critically evaluate targeted therapies, immunotherapies (including checkpoint inhibitors, CAR-T cell therapy, and oncolytic viruses), gene therapies, and emerging modalities such as cancer vaccines and precision radiotherapy. Furthermore, we discuss the challenges associated with treatment resistance, toxicity, accessibility, and cost, offering perspectives on future research directions aimed at overcoming these hurdles and improving patient outcomes across diverse cancer types.
Many thanks to our sponsor Esdebe who helped us prepare this research report.
1. Introduction
For decades, the mainstay of cancer treatment relied on surgery, chemotherapy, and radiation therapy. While these modalities have proven effective in many contexts, they often lack specificity, leading to significant off-target toxicities and limited efficacy against advanced or metastatic disease. The past two decades have witnessed a revolution in our understanding of cancer biology, revealing the intricate molecular mechanisms driving tumor development, progression, and metastasis. This knowledge has paved the way for the development of more targeted and sophisticated therapeutic strategies that aim to selectively eradicate cancer cells while minimizing harm to healthy tissues.
This review provides a comprehensive overview of the most promising emerging cancer therapies. We delve into the scientific principles underpinning each modality, assess their clinical efficacy and safety profiles, and discuss the challenges and future directions in their development and application. Our goal is to provide a critical analysis of the evolving landscape of cancer therapy, highlighting both the opportunities and the limitations that lie ahead.
Many thanks to our sponsor Esdebe who helped us prepare this research report.
2. Targeted Therapies: Precision Strikes Against Cancer Vulnerabilities
Targeted therapies represent a significant advancement over conventional cytotoxic chemotherapy. These agents are designed to selectively inhibit specific molecular targets that are critical for cancer cell growth, survival, and proliferation. These targets are often proteins involved in signal transduction pathways, such as growth factor receptors, kinases, and transcription factors. Targeted therapies are typically small molecules or monoclonal antibodies.
2.1. Small Molecule Inhibitors
Small molecule inhibitors are designed to bind to and inhibit the activity of specific intracellular targets, such as kinases. Examples include tyrosine kinase inhibitors (TKIs) such as imatinib for chronic myeloid leukemia (CML) targeting the BCR-ABL fusion protein, EGFR inhibitors (gefitinib, erlotinib) for non-small cell lung cancer (NSCLC) harboring EGFR mutations, and BRAF inhibitors (vemurafenib, dabrafenib) for melanoma with BRAF V600E mutations. The efficacy of these agents is highly dependent on the presence of the target mutation or overexpression of the target protein. The development of resistance is a significant challenge with small molecule inhibitors. Resistance can arise through various mechanisms, including secondary mutations in the target protein, activation of alternative signaling pathways, or increased drug efflux. Overcoming resistance often requires the development of second- or third-generation inhibitors that target different epitopes or pathways or combination therapies that block multiple signaling pathways simultaneously.
2.2. Monoclonal Antibodies
Monoclonal antibodies (mAbs) are antibodies that are specifically designed to bind to a particular target, typically a cell surface protein. mAbs can exert their anti-cancer effects through several mechanisms, including direct inhibition of the target protein, antibody-dependent cell-mediated cytotoxicity (ADCC), and complement-dependent cytotoxicity (CDC). Examples include trastuzumab for HER2-positive breast cancer, bevacizumab for VEGF-expressing cancers, and cetuximab for EGFR-expressing colorectal cancer. A major limitation of monoclonal antibody therapy is that it can be costly to produce at scale. Furthermore, some cancers evade antibody action through mechanisms like antigen shedding and receptor internalization.
2.3. Antibody-Drug Conjugates (ADCs)
Antibody-drug conjugates (ADCs) combine the specificity of monoclonal antibodies with the cytotoxic potency of chemotherapy drugs. ADCs consist of an antibody linked to a cytotoxic payload via a chemical linker. Upon binding to the target antigen on the cancer cell surface, the ADC is internalized and the cytotoxic drug is released, leading to cell death. Trastuzumab emtansine (T-DM1) is an example of ADC for HER2-positive breast cancer and Brentuximab vedotin used in treatment of lymphoma. ADCs represent a promising approach to improve the therapeutic index of chemotherapy drugs by selectively delivering them to cancer cells. However, challenges remain, including linker stability, drug payload toxicity, and development of resistance.
Many thanks to our sponsor Esdebe who helped us prepare this research report.
3. Immunotherapy: Harnessing the Power of the Immune System
Immunotherapy has emerged as a transformative approach to cancer therapy, leveraging the power of the patient’s own immune system to recognize and eliminate cancer cells. Unlike traditional therapies that directly target cancer cells, immunotherapy aims to enhance the anti-tumor immune response, providing durable and potentially curative benefits in some patients.
3.1. Immune Checkpoint Inhibitors
Immune checkpoint inhibitors (ICIs) are antibodies that block immune checkpoint proteins, such as CTLA-4, PD-1, and PD-L1, which normally function to suppress the immune response and prevent autoimmunity. By blocking these checkpoints, ICIs release the brakes on the immune system, allowing T cells to recognize and attack cancer cells. ICIs have demonstrated remarkable efficacy in a variety of cancers, including melanoma, lung cancer, kidney cancer, and bladder cancer. Pembrolizumab and Nivolumab are well known examples. However, ICIs are not without their limitations. Only a subset of patients respond to ICIs, and responses can vary greatly depending on the cancer type and patient characteristics. Furthermore, ICIs can cause immune-related adverse events (irAEs), which can range from mild to severe and can affect virtually any organ system. The development of predictive biomarkers to identify patients who are most likely to respond to ICIs and the management of irAEs are important areas of ongoing research.
3.2. CAR-T Cell Therapy
Chimeric antigen receptor (CAR) T-cell therapy is a form of adoptive cell therapy that involves engineering a patient’s own T cells to express a CAR that specifically recognizes a tumor-associated antigen. The engineered CAR-T cells are then infused back into the patient, where they can target and kill cancer cells. CAR-T cell therapy has shown remarkable efficacy in the treatment of relapsed/refractory B-cell malignancies, such as acute lymphoblastic leukemia (ALL) and diffuse large B-cell lymphoma (DLBCL). However, CAR-T cell therapy is associated with significant toxicities, including cytokine release syndrome (CRS) and neurotoxicity. Furthermore, the high cost and complex manufacturing process of CAR-T cell therapy limit its accessibility to many patients. Research is ongoing to develop safer and more effective CAR-T cell therapies and to expand their application to other cancer types.
3.3. Oncolytic Viruses
Oncolytic viruses (OVs) are viruses that are engineered to selectively infect and kill cancer cells while sparing normal cells. OVs can exert their anti-cancer effects through several mechanisms, including direct lysis of infected cancer cells, induction of anti-tumor immunity, and disruption of tumor vasculature. Talimogene laherparepvec (T-VEC) is an example of OV approved for treatment of melanoma. OVs represent a promising approach to cancer therapy, but challenges remain, including the development of resistance, off-target effects, and difficulty in delivering OVs to tumor sites. Clinical trials utilizing various genetically modified viruses are ongoing for multiple cancer types.
3.4. Cancer Vaccines
Cancer vaccines aim to stimulate the immune system to recognize and attack cancer cells. Therapeutic cancer vaccines are designed to treat existing cancer, while preventive cancer vaccines are designed to prevent cancer from developing in the first place. Several types of cancer vaccines are being developed, including peptide vaccines, DNA vaccines, and cell-based vaccines. While some cancer vaccines have shown promise in clinical trials, their efficacy has generally been limited. The development of more effective cancer vaccines requires a better understanding of the tumor microenvironment and the mechanisms of immune evasion.
Many thanks to our sponsor Esdebe who helped us prepare this research report.
4. Gene Therapy: Correcting Genetic Aberrations in Cancer
Gene therapy involves the introduction of genetic material into cells to treat or prevent disease. In cancer, gene therapy can be used to correct genetic defects that contribute to tumor development, enhance the immune response against cancer cells, or deliver cytotoxic genes to selectively kill cancer cells.
4.1. Viral Vectors
Viral vectors are the most commonly used method for delivering genes into cells. Adenoviruses, retroviruses, and adeno-associated viruses (AAVs) are commonly used as viral vectors. Viral vectors can be engineered to deliver therapeutic genes into cancer cells, such as tumor suppressor genes, suicide genes, or genes that encode for immunomodulatory proteins. While viral vectors are highly efficient at delivering genes into cells, they can also be associated with safety concerns, such as insertional mutagenesis and immunogenicity.
4.2. Non-Viral Vectors
Non-viral vectors, such as liposomes and nanoparticles, offer a safer alternative to viral vectors. Non-viral vectors are less efficient at delivering genes into cells, but they are less likely to cause insertional mutagenesis or elicit an immune response. Non-viral vectors can be used to deliver therapeutic genes, RNA interference (RNAi), or CRISPR-Cas9 gene editing tools to cancer cells.
4.3. CRISPR-Cas9 Gene Editing
CRISPR-Cas9 is a revolutionary gene editing technology that allows scientists to precisely target and modify DNA sequences. CRISPR-Cas9 has the potential to correct genetic defects that drive cancer development, such as mutations in tumor suppressor genes or oncogenes. CRISPR-Cas9 can also be used to engineer immune cells to enhance their anti-tumor activity. While CRISPR-Cas9 holds great promise for cancer therapy, challenges remain, including off-target effects and delivery to tumor sites.
Many thanks to our sponsor Esdebe who helped us prepare this research report.
5. Emerging Modalities and Future Directions
Beyond the therapies discussed above, several other emerging modalities are showing promise in cancer therapy. These include:
- Precision Radiotherapy: Advances in imaging and radiation delivery techniques are enabling more precise targeting of tumors with radiation, minimizing damage to surrounding healthy tissues. Stereotactic body radiation therapy (SBRT) and proton therapy are examples of precision radiotherapy techniques.
- Adoptive Cell Therapies Beyond CAR-T: Other adoptive cell therapies, such as tumor-infiltrating lymphocytes (TILs) and natural killer (NK) cell therapies, are being investigated for their potential to treat cancer. These therapies involve isolating immune cells from the patient’s tumor or blood, expanding them ex vivo, and then infusing them back into the patient.
- RNA-Based Therapies: RNA interference (RNAi) and messenger RNA (mRNA) therapies are being developed to target specific genes or proteins involved in cancer development. RNAi can be used to silence oncogenes or enhance the expression of tumor suppressor genes. mRNA therapies can be used to deliver therapeutic proteins or vaccines to cancer cells.
- Microbiome Modulation: The gut microbiome has been shown to play a role in cancer development and response to therapy. Strategies to modulate the gut microbiome, such as fecal microbiota transplantation (FMT) and probiotics, are being investigated for their potential to improve cancer treatment outcomes.
- Combination Therapies: Combining different therapeutic modalities, such as targeted therapies, immunotherapies, and radiation therapy, is emerging as a promising strategy to overcome resistance and improve treatment efficacy. Careful design of combination therapies is essential to minimize toxicities and maximize synergistic effects.
The future of cancer therapy is likely to involve a more personalized and integrated approach, where treatment decisions are based on the individual patient’s tumor characteristics, genetic background, and immune status. Advances in genomics, proteomics, and imaging are enabling a better understanding of cancer biology and facilitating the development of more targeted and personalized therapies. Artificial intelligence (AI) and machine learning (ML) are also playing an increasingly important role in cancer research, helping to identify new drug targets, predict treatment response, and optimize treatment strategies.
Many thanks to our sponsor Esdebe who helped us prepare this research report.
6. Challenges and Limitations
Despite the remarkable progress in cancer therapy, significant challenges and limitations remain.
6.1. Treatment Resistance
A major challenge in cancer therapy is the development of resistance to treatment. Cancer cells can develop resistance to targeted therapies, immunotherapies, and chemotherapy through various mechanisms, including mutations in the target protein, activation of alternative signaling pathways, and increased drug efflux. Overcoming resistance requires a better understanding of the mechanisms of resistance and the development of strategies to target resistance pathways.
6.2. Toxicity
Many cancer therapies are associated with significant toxicities, which can limit their use and impact patient quality of life. Targeted therapies can cause on-target toxicities, while immunotherapies can cause immune-related adverse events. Chemotherapy can cause a wide range of toxicities, including nausea, vomiting, hair loss, and myelosuppression. Minimizing toxicities requires the development of more selective therapies and the implementation of strategies to manage treatment-related adverse events. Prophylactic administration of medications like antiemetics and growth factors are commonly applied to reduce toxicity.
6.3. Accessibility and Cost
Many of the newer cancer therapies are expensive and not readily accessible to all patients. The high cost of these therapies can create disparities in access to care, particularly for patients in low-income countries. Furthermore, the complex manufacturing process of some therapies, such as CAR-T cell therapy, limits their availability. Reducing the cost and improving the accessibility of cancer therapies are important goals.
6.4. Tumor Heterogeneity
Tumor heterogeneity, both within and between patients, poses a significant challenge to cancer therapy. Different cancer cells within the same tumor can have different genetic and molecular characteristics, which can affect their response to therapy. Tumor heterogeneity can lead to the development of resistance and disease relapse. Addressing tumor heterogeneity requires the development of personalized treatment strategies that take into account the individual patient’s tumor characteristics.
Many thanks to our sponsor Esdebe who helped us prepare this research report.
7. Conclusion
The field of cancer therapy is rapidly evolving, with new modalities and approaches constantly emerging. Targeted therapies, immunotherapies, and gene therapies have shown remarkable promise in the treatment of cancer, but significant challenges and limitations remain. Overcoming these challenges will require a continued investment in basic and translational research, as well as a commitment to developing more personalized and accessible therapies. The future of cancer therapy lies in a more integrated and personalized approach, where treatment decisions are based on the individual patient’s tumor characteristics, genetic background, and immune status. As we continue to unravel the complexities of cancer biology, we can expect to see even more innovative and effective therapies emerge in the years to come, ultimately leading to improved outcomes for patients with cancer.
Many thanks to our sponsor Esdebe who helped us prepare this research report.
References
- Schreiber, R. D., Old, L. J., & Smyth, M. J. (2011). Cancer immunoediting: integrating immunity’s roles in cancer suppression and promotion. Science, 331(6024), 1565-1570.
- Holohan, C., Van Schaeybroeck, S., Longley, D. B., & Johnston, P. G. (2013). Cancer drug resistance: an evolving paradigm. Nature Reviews Cancer, 13(10), 714-726.
- Rosenberg, S. A., Restifo, N. P. (2015). Adoptive cell transfer as personalized immunotherapy for human cancer. Science, 348(6230), 62-68.
- Ribas, A., & Wolchok, J. D. (2018). Cancer immunotherapy comes of age. Science, 359(6382), 1350-1355.
- National Cancer Institute. (n.d.). Targeted Cancer Therapies. Retrieved from https://www.cancer.gov/about-cancer/treatment/types/targeted-therapies
- Bagley, S. J., Gray, R., & Gray, J. W. (2023). Precision Oncology: Past, Present, and Future. Annual Review of Medicine, 74, 457-474.
- June, C. H., Sadelain, M., & Maus, M. V. (2016). Engineering T cells for cancer therapy. Cancer Immunology Research, 2(2), 79-84.
- Vesely, M. D., Kershaw, M. H., Schreiber, R. D., & Smyth, M. J. (2011). Natural innate and adaptive immunity to cancer. Annual Review of Immunology, 29, 235-271.
- Seymour, L. W., Fisher, K. D., & Shankaraiah, R. C. (2017). Systemic cancer gene therapy: Hurdles and opportunities. British Journal of Cancer, 117(1), 1-15.
- Friedman, A. A., Letai, A., Fisher, D. E., & Flaherty, K. T. (2015). Feedback mechanisms regulating MAPK pathway signaling in cancer. Journal of Molecular Cell Biology, 7(2), 131-143.
- Tang, L., Swiderska-Syn, M., Finberg, R. W., & Chen, J. (2020). Oncolytic virus-based cancer immunotherapy: prospects and challenges. Immunotherapy, 12(9), 681-696.
- Gattinoni, L., Klebanoff, C. A., Restifo, N. P. (2011). Paths to stemness: building the ultimate antitumour T cell. Nature Reviews Cancer, 12(1), 671-684.
- Liu, C., Zhang, J., Zhong, M., Li, C., Yu, J., Yin, W., … & Wang, F. (2022). Advances in cancer vaccines. Signal Transduction and Targeted Therapy, 7(1), 178.
The discussion on overcoming treatment resistance is critical. Further exploration into predictive biomarkers and real-time monitoring of drug efficacy could significantly improve personalized treatment strategies and patient outcomes.