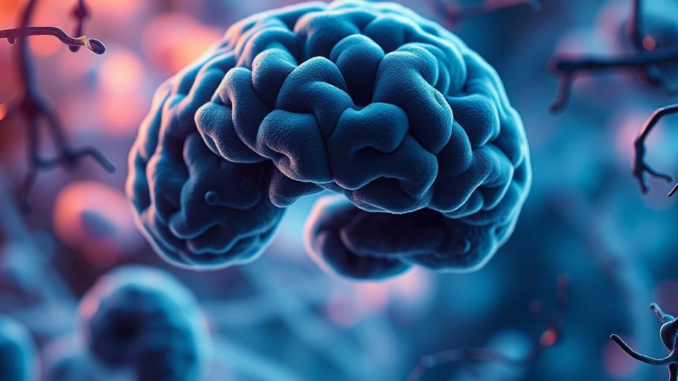
The Gut Microbiome: A Central Regulator of Host Metabolism, Immunity, and Neurodevelopment – Implications for Health and Disease
Many thanks to our sponsor Esdebe who helped us prepare this research report.
Abstract
The gut microbiome, a complex and dynamic ecosystem of microorganisms residing within the gastrointestinal tract, has emerged as a pivotal regulator of host physiology. Beyond its established role in nutrient metabolism, recent research has highlighted the microbiome’s profound influence on immune system development, inflammatory responses, neurodevelopment, and even behavior. This report provides a comprehensive overview of the multifaceted roles of the gut microbiome, exploring its composition, function, and impact on various aspects of host health and disease. We delve into the intricate mechanisms by which the microbiome interacts with the host, including the production of microbial metabolites, modulation of immune cell populations, and direct signaling to the nervous system. Furthermore, we discuss the influence of environmental factors, such as diet, antibiotics, and lifestyle, on microbiome composition and function. Finally, we critically examine the potential for microbiome-targeted interventions, including fecal microbiota transplantation (FMT), prebiotics, probiotics, and phage therapy, to treat or prevent a range of diseases, while acknowledging the inherent challenges and ethical considerations associated with manipulating this complex ecosystem.
Many thanks to our sponsor Esdebe who helped us prepare this research report.
1. Introduction
The human gut microbiome is a vast and dynamic community comprising trillions of bacteria, archaea, fungi, viruses, and other microorganisms. This intricate ecosystem, collectively weighing approximately 2 kg, plays a crucial role in host physiology, extending beyond its traditional association with digestion and nutrient absorption. The development of advanced sequencing technologies, such as 16S rRNA gene sequencing and metagenomics, has revolutionized our understanding of the gut microbiome’s composition and functional potential. These techniques have revealed an astounding level of microbial diversity, with each individual harboring a unique microbial fingerprint shaped by genetic predisposition, diet, lifestyle, and environmental exposures [1, 2].
Growing evidence suggests that the gut microbiome functions as a virtual organ, actively participating in numerous host processes, including the fermentation of dietary fibers, synthesis of essential vitamins, metabolism of xenobiotics, and development of the immune system [3]. Disruptions in the composition and function of the gut microbiome, termed dysbiosis, have been implicated in a wide range of diseases, including inflammatory bowel disease (IBD), obesity, type 2 diabetes, cardiovascular disease, autoimmune disorders, and even neurological conditions such as autism spectrum disorder (ASD) and Parkinson’s disease [4, 5].
This report aims to provide a comprehensive overview of the gut microbiome’s diverse roles in host health and disease. We will explore the intricate mechanisms by which the microbiome interacts with the host, the factors that influence its composition and function, and the potential for microbiome-targeted interventions to prevent or treat various diseases. We will also discuss the challenges and ethical considerations associated with manipulating the gut microbiome, emphasizing the need for rigorous scientific investigation and cautious implementation of microbiome-based therapies.
Many thanks to our sponsor Esdebe who helped us prepare this research report.
2. Composition and Function of the Gut Microbiome
The gut microbiome is a complex and dynamic ecosystem whose composition varies considerably among individuals. While hundreds of bacterial species reside in the human gut, the majority of the microbial biomass is represented by a relatively small number of phyla, including Firmicutes, Bacteroidetes, Actinobacteria, Proteobacteria, and Verrucomicrobia [6]. The relative abundance of these phyla, as well as the specific species within each phylum, can vary significantly depending on factors such as age, diet, and geographical location.
The Firmicutes phylum is typically the most abundant in the human gut, encompassing a diverse range of species involved in the fermentation of dietary fibers and the production of short-chain fatty acids (SCFAs). Bacteroidetes are also highly prevalent and are particularly adept at degrading complex carbohydrates. Actinobacteria, including the genus Bifidobacterium, are often associated with beneficial effects on host health, particularly in infants and young children. Proteobacteria, while typically present in lower abundance, can become dominant during periods of dysbiosis and have been implicated in inflammatory processes [7].
Beyond bacteria, the gut microbiome also harbors a diverse array of archaea, fungi, viruses, and other microorganisms. Archaea, particularly methanogens, play a crucial role in the metabolism of hydrogen produced during bacterial fermentation. Fungi, while present in lower abundance than bacteria, can significantly impact host immunity and gut homeostasis. Viruses, including bacteriophages, can influence bacterial populations through predation and horizontal gene transfer, thereby shaping the overall structure and function of the microbiome [8].
The functional capabilities of the gut microbiome are vast and diverse, encompassing a wide range of metabolic processes that impact host health. Key functions include:
- Fermentation of dietary fibers: The microbiome ferments undigested dietary fibers, producing SCFAs such as acetate, propionate, and butyrate. SCFAs serve as a primary energy source for colonocytes, promote gut barrier integrity, and exert anti-inflammatory effects throughout the body [9].
- Synthesis of vitamins: The microbiome synthesizes essential vitamins, including vitamin K and B vitamins, which are absorbed by the host.
- Metabolism of bile acids: The microbiome modifies bile acids secreted by the liver, influencing cholesterol metabolism and the regulation of gut microbiota composition [10].
- Production of neurotransmitters: Certain gut bacteria produce neurotransmitters such as serotonin, dopamine, and GABA, which can influence mood, behavior, and gut motility [11].
- Xenobiotic metabolism: The microbiome metabolizes xenobiotics, including drugs and environmental toxins, potentially influencing their bioavailability and toxicity [12].
Many thanks to our sponsor Esdebe who helped us prepare this research report.
3. Mechanisms of Microbiome-Host Interaction
The gut microbiome interacts with the host through a variety of complex mechanisms, influencing host physiology at both local and systemic levels. These interactions involve the production of microbial metabolites, modulation of immune cell populations, and direct signaling to the nervous system.
3.1. Microbial Metabolites
Microbial metabolites are small molecules produced by gut microbes that can exert a wide range of effects on host cells and tissues. SCFAs, as mentioned above, are among the most well-studied microbial metabolites. Butyrate, in particular, is a key energy source for colonocytes and plays a critical role in maintaining gut barrier integrity. Acetate and propionate are absorbed into the bloodstream and can influence hepatic glucose metabolism and lipid metabolism [13].
Other important microbial metabolites include:
- Tryptophan metabolites: The microbiome metabolizes tryptophan, an essential amino acid, into a variety of bioactive compounds, including indole derivatives, which can activate the aryl hydrocarbon receptor (AhR) and modulate immune responses [14].
- Bile acid metabolites: The microbiome modifies bile acids into secondary bile acids, such as deoxycholic acid (DCA) and lithocholic acid (LCA), which can influence cholesterol metabolism and regulate gut microbiota composition [15].
- Trimethylamine N-oxide (TMAO): Certain gut bacteria convert dietary choline and L-carnitine into trimethylamine (TMA), which is then oxidized in the liver to TMAO. Elevated levels of TMAO have been associated with an increased risk of cardiovascular disease [16].
3.2. Immune Modulation
The gut microbiome plays a critical role in the development and regulation of the host immune system. The constant exposure of the immune system to microbial antigens in the gut promotes the maturation and differentiation of immune cells, shaping the balance between pro-inflammatory and anti-inflammatory responses.
Specific mechanisms of immune modulation include:
- Activation of pattern recognition receptors (PRRs): Gut microbes express a variety of pathogen-associated molecular patterns (PAMPs) that are recognized by PRRs on immune cells, such as Toll-like receptors (TLRs) and NOD-like receptors (NLRs). Activation of these receptors triggers downstream signaling pathways that can influence the production of cytokines and chemokines, shaping the inflammatory response [17].
- Induction of regulatory T cells (Tregs): Certain gut bacteria, such as Faecalibacterium prausnitzii and Bacteroides fragilis, can promote the differentiation and expansion of Tregs, which suppress inflammation and maintain immune homeostasis [18].
- Production of secretory IgA (sIgA): The gut microbiome stimulates the production of sIgA, an antibody that binds to microbes and prevents their adherence to the gut epithelium, thereby limiting their ability to trigger inflammatory responses [19].
3.3. Gut-Brain Axis
The gut microbiome communicates with the brain through a bidirectional pathway known as the gut-brain axis. This axis involves neural, hormonal, and immune signaling pathways, allowing for cross-talk between the gut and the central nervous system.
Specific mechanisms of gut-brain communication include:
- Vagus nerve signaling: The vagus nerve, the longest cranial nerve, provides a direct neural connection between the gut and the brain. Gut microbes can influence vagal nerve activity through the production of neurotransmitters and other signaling molecules [20].
- Enteroendocrine cells: Enteroendocrine cells in the gut secrete hormones that can influence brain function. Gut microbes can stimulate the release of these hormones, modulating appetite, mood, and behavior [21].
- Immune signaling: Gut microbes can influence brain function through the modulation of systemic inflammation. Dysbiosis and increased intestinal permeability can lead to systemic inflammation, which can affect brain function and contribute to neurological disorders [22].
Many thanks to our sponsor Esdebe who helped us prepare this research report.
4. Factors Influencing Gut Microbiome Composition and Function
The composition and function of the gut microbiome are influenced by a variety of factors, including genetics, diet, lifestyle, and environmental exposures. Understanding these factors is crucial for developing strategies to modulate the microbiome and promote health.
4.1. Genetics
Genetic factors can influence the composition of the gut microbiome. Studies of twins have shown that genetically identical twins share more similar gut microbiome profiles than non-identical twins [23]. Specific genes involved in immune function, gut barrier integrity, and nutrient metabolism can influence the types of microbes that colonize the gut.
4.2. Diet
Diet is a major determinant of gut microbiome composition and function. Different dietary components can selectively promote the growth of certain types of microbes. For example, diets high in fiber promote the growth of bacteria that ferment dietary fibers, such as Bifidobacterium and Lactobacillus, while diets high in saturated fat can promote the growth of bacteria that produce lipopolysaccharide (LPS), a potent pro-inflammatory molecule [24].
4.3. Antibiotics
Antibiotics can have a profound impact on gut microbiome composition and function. Antibiotics can kill both beneficial and harmful bacteria, leading to a reduction in microbial diversity and a disruption of the balance between different microbial populations. Antibiotic use has been associated with an increased risk of various diseases, including IBD, obesity, and Clostridium difficile infection [25].
4.4. Lifestyle
Lifestyle factors such as exercise, sleep, and stress can also influence gut microbiome composition and function. Exercise has been shown to increase microbial diversity and promote the growth of beneficial bacteria. Sleep deprivation and chronic stress can disrupt the gut microbiome, leading to increased intestinal permeability and systemic inflammation [26].
4.5. Environmental Exposures
Environmental exposures, such as exposure to pollutants and toxins, can also influence gut microbiome composition and function. Exposure to certain pesticides and heavy metals can disrupt the gut microbiome and increase the risk of various diseases [27].
Many thanks to our sponsor Esdebe who helped us prepare this research report.
5. Microbiome-Targeted Interventions
Given the profound influence of the gut microbiome on host health and disease, there is growing interest in developing microbiome-targeted interventions to prevent or treat various conditions. These interventions include fecal microbiota transplantation (FMT), prebiotics, probiotics, and phage therapy.
5.1. Fecal Microbiota Transplantation (FMT)
FMT involves the transfer of fecal material from a healthy donor to a recipient with a disrupted gut microbiome. FMT has been shown to be highly effective in treating recurrent Clostridium difficile infection and is being investigated as a potential therapy for other conditions, including IBD, obesity, and metabolic syndrome [28].
5.2. Prebiotics
Prebiotics are non-digestible food ingredients that selectively promote the growth of beneficial bacteria in the gut. Prebiotics, such as inulin and fructooligosaccharides (FOS), can increase the abundance of Bifidobacterium and Lactobacillus, which are associated with improved gut health and immune function [29].
5.3. Probiotics
Probiotics are live microorganisms that, when administered in adequate amounts, confer a health benefit on the host. Probiotics, such as Lactobacillus and Bifidobacterium strains, can improve gut health by competing with harmful bacteria, producing antimicrobial substances, and modulating immune responses [30].
5.4. Phage Therapy
Phage therapy involves the use of bacteriophages, viruses that infect and kill bacteria, to selectively target and eliminate harmful bacteria in the gut. Phage therapy has the potential to be a more targeted and precise approach to modulating the gut microbiome than antibiotics [31].
Many thanks to our sponsor Esdebe who helped us prepare this research report.
6. Challenges and Ethical Considerations
While microbiome-targeted interventions hold great promise for treating and preventing various diseases, there are also significant challenges and ethical considerations that need to be addressed. These include:
- Complexity of the microbiome: The gut microbiome is a complex and dynamic ecosystem, and our understanding of its composition and function is still incomplete. It is challenging to predict how specific interventions will affect the microbiome and the host.
- Inter-individual variability: The gut microbiome varies considerably among individuals, making it difficult to develop universal microbiome-targeted interventions that will be effective for everyone.
- Long-term effects: The long-term effects of microbiome-targeted interventions are not fully understood. It is important to monitor individuals who undergo these interventions for any adverse effects.
- Ethical considerations: FMT raises ethical concerns about donor screening, informed consent, and the potential for unintended consequences. The use of probiotics and prebiotics also raises ethical concerns about marketing claims and the potential for misleading consumers.
Many thanks to our sponsor Esdebe who helped us prepare this research report.
7. Future Directions
Future research on the gut microbiome should focus on the following areas:
- Developing more precise methods for characterizing the gut microbiome: This includes the development of new sequencing technologies and computational tools for analyzing microbiome data.
- Identifying specific microbial species and metabolites that are associated with health and disease: This will require large-scale studies that correlate microbiome data with clinical outcomes.
- Developing more targeted and effective microbiome-targeted interventions: This includes the development of personalized microbiome-based therapies that are tailored to the individual’s specific microbiome profile.
- Addressing the ethical challenges associated with microbiome manipulation: This includes the development of guidelines for donor screening, informed consent, and the responsible marketing of probiotics and prebiotics.
Many thanks to our sponsor Esdebe who helped us prepare this research report.
8. Conclusion
The gut microbiome is a central regulator of host metabolism, immunity, and neurodevelopment. Disruptions in the gut microbiome have been implicated in a wide range of diseases, highlighting the importance of maintaining a healthy gut microbiome. Microbiome-targeted interventions, such as FMT, prebiotics, probiotics, and phage therapy, hold great promise for treating and preventing various diseases. However, these interventions also raise significant challenges and ethical considerations that need to be addressed. Future research should focus on developing more precise methods for characterizing the gut microbiome, identifying specific microbial species and metabolites that are associated with health and disease, and developing more targeted and effective microbiome-targeted interventions.
Many thanks to our sponsor Esdebe who helped us prepare this research report.
References
[1] Qin, J., et al. (2010). A human gut microbial gene catalogue established by metagenomic sequencing. Nature, 464(7285), 59-65.
[2] Knights, D., et al. (2011). Complex ecological dynamics of the human gut microbiome. Science, 332(6028), 434-438.
[3] Gill, S. R., et al. (2006). Metagenomic analysis of the human distal gut microbiome. Science, 312(5778), 1355-1359.
[4] Rooks, M. G., & Garrett, W. S. (2016). Gut microbiota, metabolites and host immunity. Nature Reviews Immunology, 16(6), 341-352.
[5] Cryan, J. F., et al. (2019). The gut microbiome in neurological disorders. The Lancet Neurology, 18(9), 895-908.
[6] Eckburg, P. B., et al. (2005). Diversity of the human intestinal microbial flora. Science, 308(5728), 1635-1638.
[7] Shin, N. R., et al. (2015). An overview of representative gut microbiota. Gut microbes, 6(4), 313-333.
[8] Reyes, A., et al. (2010). Viral dark matter in the human gut. Nature, 466(7307), 754-756.
[9] Morrison, D. J., & Preston, T. (2016). Formation of short chain fatty acids by the gut microbiota and their impact on human health. Gut microbes, 7(3), 189-200.
[10] Ridlon, J. M., et al. (2016). Bile acids and the gut microbiome. Current opinion in gastroenterology, 32(6), 499.
[11] Strandwitz, P., et al. (2019). GABA-modulating bacteria of the human gut. Nature microbiology, 4(3), 399-403.
[12] Claus, S. P., et al. (2016). The gut microbiota: a modulator of xenobiotic metabolism. Current drug metabolism, 17(2), 122-136.
[13] den Besten, G., et al. (2013). The role of short-chain fatty acids in the interplay between diet, gut microbiota, and host energy metabolism. Journal of lipid research, 54(9), 2325-2340.
[14] Agus, A., et al. (2018). The role of the gut microbiota in tryptophan metabolism and its influence on the gut-brain axis. Gut microbes, 9(3), 228-253.
[15] Song, X., et al. (2020). Gut microbial bile acid metabolism in host physiology and disease. Cell metabolism, 31(2), 259-272.
[16] Tang, W. H., et al. (2013). Intestinal microbial metabolism of phosphatidylcholine and cardiovascular risk. New England Journal of Medicine, 368(17), 1575-1584.
[17] Kawai, T., & Akira, S. (2010). The role of pattern-recognition receptors in innate immunity: update on Toll-like receptors. Nature immunology, 11(5), 373-384.
[18] Round, J. L., & Mazmanian, S. K. (2010). Inducible Foxp3+ regulatory T-cell development by a commensal bacterium of the intestinal microbiota. Proceedings of the National Academy of Sciences, 107(27), 12204-12209.
[19] Mantis, N. J., et al. (2011). Secretory IgA and intestinal homeostasis. Immunological reviews, 241(1), 153-162.
[20] Breit, S., et al. (2018). Vagus nerve as modulator of the brain-gut axis in psychiatric and inflammatory disorders. Frontiers in psychiatry, 9, 44.
[21] Holzer, P. (2011). The gut-brain axis: implications for enteric and extraenteric diseases. Current gastroenterology reports, 13(3), 230-238.
[22] Dinan, T. G., et al. (2015). Collective unconscious: how gut microbes shape human behavior. Journal of psychiatric research, 68, 1-9.
[23] Goodrich, J. K., et al. (2014). Human genetics shape the gut microbiome. Cell, 159(4), 789-799.
[24] David, L. A., et al. (2014). Diet rapidly and reproducibly alters the human gut microbiome. Nature, 505(7484), 559-563.
[25] Modi, S. R., et al. (2011). Antibiotics and the gut microbiota. Gut microbes, 2(3), 170-175.
[26] Madison, A., & Kiecolt-Glaser, J. K. (2019). Stress, depression, diet, and the gut microbiota: human-bacteria interactions at the core of psychoneuroimmunology. Current opinion in behavioral sciences, 28, 105-110.
[27] Lozupone, C. A., et al. (2012). Environmental exposure and human gut microbiota composition. Environmental microbiology, 14(7), 1638-1649.
[28] van Nood, E., et al. (2013). Fecal microbiota transplantation for recurrent Clostridium difficile infection. New England Journal of Medicine, 368(5), 407-415.
[29] Roberfroid, M. (2007). Prebiotics: the concept revisited. The Journal of nutrition, 137(3 Suppl 2), 830S-837S.
[30] Hill, C., et al. (2014). Expert consensus document: The International Scientific Association for Probiotics and Prebiotics consensus statement on the scope and appropriate use of the term probiotic. Nature reviews gastroenterology & hepatology, 11(8), 506-514.
[31] Kortright, K. E., et al. (2019). Phage therapy: a renewed approach to combat antibiotic-resistant bacteria. Cell host & microbe, 25(2), 219-232.
This is a fascinating overview of the gut microbiome’s impact. The discussion of phage therapy as a targeted intervention is particularly interesting. How might personalized phage cocktails be developed to address individual microbiome imbalances more effectively?
Thank you for your insightful comment! The development of personalized phage cocktails is indeed a crucial area. One approach might involve using advanced diagnostics to map individual microbiome compositions, followed by designing phage cocktails targeting specific problematic bacteria identified in each person’s unique profile. This approach promises greater effectiveness and reduced off-target effects.
Editor: MedTechNews.Uk
Thank you to our Sponsor Esdebe
Fascinating stuff! But if our guts are so unique, does that mean my meticulously crafted sourdough starter, nurtured for years, is just a glorified random bacterial soup to everyone else? Should I abandon my dreams of world-class bread and embrace the personalized-microbiome-targeted-sourdough future?
That’s a great question! While individual microbiomes *are* unique, your sourdough starter likely contains beneficial microbial communities that could positively interact with *some* gut microbiomes. Instead of abandoning your bread dreams, perhaps explore ways to analyze and tailor starters for specific benefits! The personalized food future might be closer than we think.
Editor: MedTechNews.Uk
Thank you to our Sponsor Esdebe