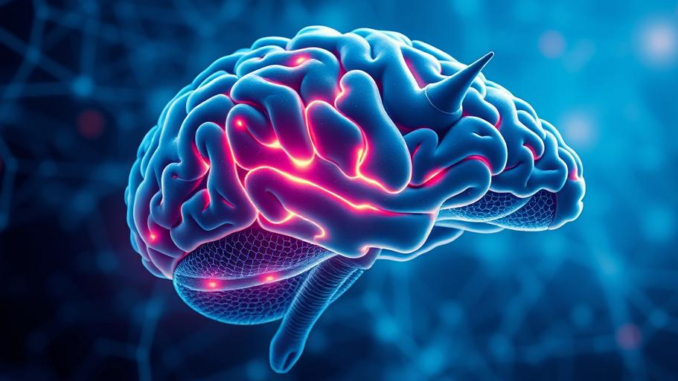
Abstract
The hippocampus, a seahorse-shaped structure nestled within the medial temporal lobe, has long been recognized as critical for memory formation. This review provides a comprehensive overview of the hippocampus, delving into its intricate structure, diverse functions beyond memory, remarkable plasticity, and pronounced vulnerability in a range of neurological and psychiatric disorders. We explore the hippocampal formation’s cytoarchitecture, its afferent and efferent connections, and the molecular mechanisms underlying long-term potentiation (LTP) and long-term depression (LTD), the cellular substrates of learning and memory. We extend the discussion beyond the hippocampus’s well-established role in spatial and episodic memory to encompass its involvement in emotional regulation, contextual processing, and cognitive mapping. Furthermore, we critically examine the impact of various neurological and psychiatric conditions, including Alzheimer’s disease, temporal lobe epilepsy, schizophrenia, depression, traumatic brain injury, and chronic stress, on hippocampal structure and function. We explore the underlying pathological mechanisms contributing to hippocampal dysfunction in these disorders, including neuroinflammation, oxidative stress, excitotoxicity, and impaired neurogenesis. Finally, we discuss potential therapeutic strategies aimed at preserving or restoring hippocampal function, including pharmacological interventions, lifestyle modifications, and novel approaches such as deep brain stimulation and gene therapy. This review aims to provide a comprehensive and up-to-date understanding of the hippocampus, highlighting its multifaceted roles in cognition and behavior and underscoring its importance as a therapeutic target in various neurological and psychiatric disorders.
Many thanks to our sponsor Esdebe who helped us prepare this research report.
1. Introduction
The hippocampus, derived from the Greek words “hippos” (horse) and “kampos” (sea monster), is a bilateral structure located deep within the medial temporal lobe of the mammalian brain. Its distinctive seahorse-like shape, first described by Giulio Cesare Aranzi in 1587, belies its complex cytoarchitecture and profound influence on cognition and behavior. While famously known for its pivotal role in memory formation, particularly declarative (explicit) memory, the hippocampus contributes to a broader spectrum of cognitive processes, including spatial navigation, contextual processing, and emotional regulation. Understanding the intricacies of the hippocampus – its structure, function, plasticity, and vulnerability – is crucial for unraveling the neural basis of memory and cognition and for developing effective treatments for neurological and psychiatric disorders characterized by hippocampal dysfunction.
The seminal case of patient H.M., who underwent bilateral medial temporal lobe resection in 1953 to alleviate severe epilepsy, irrevocably established the hippocampus’s role in the formation of new long-term memories. While H.M. retained his intellectual abilities and procedural memory, he was profoundly impaired in his ability to form new declarative memories, particularly episodic memories – memories of specific events and experiences. This case provided compelling evidence that the hippocampus is not the repository of long-term memories but rather a critical structure for their encoding and consolidation. Since then, decades of research using animal models, neuroimaging techniques, and computational modeling have significantly advanced our understanding of the hippocampus’s intricate functions and its interactions with other brain regions.
This review will delve into the multifaceted aspects of the hippocampus, examining its detailed anatomy and connectivity, exploring its diverse functional roles, highlighting its remarkable plasticity, and critically assessing its vulnerability in a variety of neurological and psychiatric disorders. We will also discuss potential therapeutic strategies aimed at preserving or restoring hippocampal function in these disorders.
Many thanks to our sponsor Esdebe who helped us prepare this research report.
2. Anatomical Structure and Connectivity
The hippocampal formation is not a single, monolithic structure but rather a complex network of interconnected subregions, each with distinct cytoarchitectural characteristics and functional properties. These subregions include the dentate gyrus (DG), the hippocampus proper (comprising the cornu ammonis (CA) fields CA1, CA2, CA3, and CA4), and the subiculum. The entorhinal cortex (EC), located adjacent to the hippocampus, serves as the primary gateway for information entering and leaving the hippocampal formation.
2.1. Cytoarchitecture
Each subregion of the hippocampus exhibits a distinct laminar organization, characterized by specific cell types and neuronal connectivity patterns. The dentate gyrus is characterized by its densely packed granule cells, which receive input from the EC via the perforant pathway. The CA fields are distinguished by their pyramidal cells, the principal neurons of the hippocampus, which are organized in a tightly packed layer. The subiculum, the transitional region between the hippocampus and the EC, integrates information from the CA1 field and projects to various cortical and subcortical structures.
The EC, with its six distinct layers, plays a crucial role in processing and relaying sensory information from various cortical regions to the hippocampus. Layer II of the EC projects to the DG via the perforant pathway, while layer III projects directly to the CA1 field. These distinct pathways allow for parallel processing of information within the hippocampal formation.
2.2. Intrinsic Circuitry
The flow of information within the hippocampal formation follows a largely unidirectional pathway known as the trisynaptic circuit. This circuit begins with the perforant pathway, which carries information from the EC to the DG. Granule cells in the DG project to the CA3 field via the mossy fiber pathway. CA3 pyramidal cells, in turn, project to the CA1 field via the Schaffer collaterals. Finally, CA1 pyramidal cells project to the subiculum, which then projects back to the EC, completing the loop. This trisynaptic circuit provides a framework for processing and integrating information within the hippocampus.
Beyond the trisynaptic circuit, the hippocampus also exhibits complex intrinsic circuitry involving interneurons. These interneurons, which constitute a diverse population of inhibitory neurons, play a critical role in regulating neuronal excitability and synchronizing neuronal activity within the hippocampus. Different types of interneurons, such as parvalbumin-expressing (PV+) interneurons and somatostatin-expressing (SST+) interneurons, target different compartments of pyramidal cells and exert distinct inhibitory influences on hippocampal activity.
2.3. Afferent and Efferent Connections
The hippocampus receives inputs from a wide range of cortical and subcortical structures, allowing it to integrate information from various sensory modalities and cognitive domains. The EC, as the primary input structure, receives projections from the neocortex, including the prefrontal cortex, parietal cortex, and temporal cortex. These cortical inputs provide the hippocampus with information about spatial location, object identity, and contextual cues. Subcortical inputs to the hippocampus include the amygdala, which provides information about emotional valence, and the septum, which modulates hippocampal activity via cholinergic projections.
The hippocampus projects to a variety of cortical and subcortical structures, allowing it to influence a wide range of cognitive and behavioral processes. The subiculum projects back to the EC, completing the loop and allowing for reciprocal communication between the hippocampus and the cortex. The hippocampus also projects to the prefrontal cortex, which is involved in working memory, decision-making, and executive functions. Subcortical projections from the hippocampus include the amygdala, which is involved in emotional processing, and the hypothalamus, which is involved in regulating stress responses.
Many thanks to our sponsor Esdebe who helped us prepare this research report.
3. Functional Roles of the Hippocampus
While the hippocampus is most famously associated with memory, its functional repertoire extends beyond memory formation to encompass a diverse range of cognitive processes, including spatial navigation, contextual processing, and emotional regulation.
3.1. Memory Formation
The hippocampus plays a critical role in the formation of declarative (explicit) memories, which include episodic memories (memories of specific events and experiences) and semantic memories (memories of facts and knowledge). The hippocampus is not the storage site for long-term memories but rather a critical structure for encoding and consolidating these memories. During encoding, the hippocampus binds together disparate elements of an experience, such as the spatial context, the objects present, and the emotional valence, into a coherent memory trace. Over time, this memory trace is gradually transferred to the neocortex for long-term storage, a process known as systems consolidation.
Animal studies have shown that hippocampal lesions disrupt the formation of new episodic-like memories, while human neuroimaging studies have demonstrated that hippocampal activity is increased during the encoding and retrieval of episodic memories. The hippocampus also plays a role in semantic memory formation, although its contribution is less pronounced than its role in episodic memory. Patients with hippocampal damage may exhibit deficits in acquiring new semantic knowledge, particularly when the knowledge is closely tied to personal experiences.
3.2. Spatial Navigation
Perhaps the best-understood function of the hippocampus, aside from declarative memory, is its role in spatial navigation. Pioneering work by O’Keefe and Nadel demonstrated that hippocampal neurons in rats, termed “place cells,” fire selectively when the animal is in a specific location in its environment. These place cells form a cognitive map of the environment, allowing the animal to navigate effectively. Subsequent research has identified other types of spatially tuned neurons in the hippocampus and surrounding structures, including grid cells in the EC, head direction cells in the subiculum, and border cells in the EC, all of which contribute to the brain’s spatial navigation system.
Human neuroimaging studies have confirmed the importance of the hippocampus for spatial navigation. Participants engaged in virtual navigation tasks show increased hippocampal activity, and patients with hippocampal damage exhibit deficits in spatial navigation abilities, such as finding their way in unfamiliar environments or learning new routes.
3.3. Contextual Processing
The hippocampus plays a crucial role in contextual processing, the ability to discriminate between different contexts and to retrieve memories that are specific to a particular context. Contextual information, such as the spatial location, the time of day, and the surrounding environment, provides a framework for organizing and retrieving memories. The hippocampus integrates contextual information from various cortical regions and uses this information to guide memory retrieval. Hippocampal damage can impair the ability to discriminate between different contexts and to retrieve memories that are specific to a particular context.
3.4. Emotional Regulation
While not traditionally considered a primary function, evidence suggests that the hippocampus contributes to emotional regulation. The hippocampus has reciprocal connections with the amygdala, a key brain region involved in emotional processing. These connections allow the hippocampus to modulate the activity of the amygdala and to influence emotional responses. Hippocampal damage can disrupt emotional regulation and may contribute to the development of anxiety and depression.
For example, the hippocampus is involved in the extinction of conditioned fear responses. During extinction, the hippocampus encodes contextual information associated with the absence of the aversive stimulus, which inhibits the activity of the amygdala and reduces the fear response. Impairment in hippocampal function can disrupt fear extinction and may contribute to the maintenance of anxiety disorders.
Many thanks to our sponsor Esdebe who helped us prepare this research report.
4. Hippocampal Plasticity
The hippocampus is a highly plastic structure, capable of undergoing significant changes in its structure and function in response to experience. This plasticity is thought to be the cellular basis of learning and memory. Two key forms of synaptic plasticity in the hippocampus are long-term potentiation (LTP) and long-term depression (LTD).
4.1. Long-Term Potentiation (LTP)
LTP is a long-lasting increase in the strength of synaptic transmission following high-frequency stimulation. LTP is considered the primary cellular mechanism underlying learning and memory. LTP in the hippocampus is typically induced by stimulating Schaffer collaterals, the axons that connect CA3 pyramidal cells to CA1 pyramidal cells. This stimulation leads to a sustained increase in the excitatory postsynaptic potential (EPSP) in CA1 pyramidal cells.
The induction of LTP requires the activation of NMDA receptors, a type of glutamate receptor that is permeable to calcium ions. When NMDA receptors are activated, calcium ions enter the postsynaptic neuron, triggering a cascade of intracellular signaling events that lead to the insertion of more AMPA receptors, another type of glutamate receptor, into the postsynaptic membrane. The increased number of AMPA receptors increases the neuron’s sensitivity to glutamate, resulting in a stronger synaptic response.
4.2. Long-Term Depression (LTD)
LTD is a long-lasting decrease in the strength of synaptic transmission following low-frequency stimulation. LTD is thought to be important for weakening irrelevant synapses and for preventing the saturation of synaptic connections. LTD in the hippocampus is typically induced by stimulating Schaffer collaterals at a low frequency. This stimulation leads to a sustained decrease in the EPSP in CA1 pyramidal cells.
The induction of LTD also requires the activation of NMDA receptors, although the intracellular signaling pathways involved are different from those involved in LTP. LTD is thought to involve the removal of AMPA receptors from the postsynaptic membrane, which reduces the neuron’s sensitivity to glutamate and weakens the synaptic response.
4.3. Neurogenesis
In addition to synaptic plasticity, the hippocampus is also one of the few brain regions where neurogenesis, the birth of new neurons, occurs throughout adulthood. Adult neurogenesis in the hippocampus is restricted to the dentate gyrus, where new granule cells are generated from neural stem cells. These new granule cells migrate into the granule cell layer and integrate into the existing hippocampal circuitry.
The function of adult neurogenesis in the hippocampus is not fully understood, but it is thought to contribute to various aspects of learning and memory, including pattern separation, the ability to discriminate between similar experiences, and temporal coding, the ability to remember the order in which events occurred. Neurogenesis is also influenced by various factors, including exercise, stress, and antidepressant medications.
Many thanks to our sponsor Esdebe who helped us prepare this research report.
5. Hippocampal Vulnerability in Neurological and Psychiatric Disorders
The hippocampus is particularly vulnerable to damage in a variety of neurological and psychiatric disorders, including Alzheimer’s disease, temporal lobe epilepsy, schizophrenia, depression, traumatic brain injury, and chronic stress. The underlying pathological mechanisms contributing to hippocampal dysfunction in these disorders vary but often involve neuroinflammation, oxidative stress, excitotoxicity, and impaired neurogenesis.
5.1. Alzheimer’s Disease
Alzheimer’s disease (AD) is a progressive neurodegenerative disorder characterized by cognitive decline and memory loss. The hippocampus is one of the first brain regions to be affected in AD. Pathological hallmarks of AD in the hippocampus include the accumulation of amyloid plaques and neurofibrillary tangles, as well as neuronal loss and synaptic dysfunction. These pathological changes disrupt hippocampal circuitry and impair its ability to encode and consolidate memories.
Hippocampal atrophy, as measured by MRI, is a reliable biomarker of AD progression. The degree of hippocampal atrophy correlates with the severity of cognitive impairment. In addition to structural changes, hippocampal function is also impaired in AD. Studies using functional MRI (fMRI) have shown that hippocampal activity is reduced during memory tasks in patients with AD.
5.2. Temporal Lobe Epilepsy
Temporal lobe epilepsy (TLE) is a chronic neurological disorder characterized by recurrent seizures originating in the temporal lobe, often involving the hippocampus. TLE can cause hippocampal damage, including neuronal loss, gliosis, and mossy fiber sprouting. These pathological changes disrupt hippocampal circuitry and contribute to cognitive impairments, particularly memory deficits.
Hippocampal sclerosis, a characteristic feature of TLE, involves the selective loss of neurons in the CA1 and CA3 fields. Mossy fiber sprouting, the aberrant growth of mossy fibers from the dentate gyrus into the inner molecular layer, is also a common finding in TLE. These changes can contribute to hyperexcitability in the hippocampus and promote seizure activity.
5.3. Schizophrenia
Schizophrenia is a chronic psychiatric disorder characterized by positive symptoms (hallucinations, delusions), negative symptoms (blunted affect, social withdrawal), and cognitive deficits. The hippocampus has been implicated in the pathophysiology of schizophrenia, and studies have reported reduced hippocampal volume and altered hippocampal activity in patients with schizophrenia.
While the exact mechanisms underlying hippocampal dysfunction in schizophrenia are not fully understood, several factors may contribute, including genetic vulnerability, neurodevelopmental abnormalities, and dysregulation of neurotransmitter systems. Some studies have suggested that impaired neurogenesis in the hippocampus may also contribute to the cognitive deficits associated with schizophrenia.
5.4. Depression
Major depressive disorder (MDD) is a common psychiatric disorder characterized by persistent sadness, loss of interest, and feelings of worthlessness. The hippocampus has been implicated in the pathophysiology of depression, and studies have reported reduced hippocampal volume and altered hippocampal activity in patients with depression.
Chronic stress, a major risk factor for depression, can suppress neurogenesis in the hippocampus and lead to hippocampal atrophy. Antidepressant medications, such as selective serotonin reuptake inhibitors (SSRIs), can increase neurogenesis in the hippocampus and may contribute to their therapeutic effects. The hippocampus is thought to play a role in regulating stress responses, and dysfunction in the hippocampus may contribute to the dysregulation of the hypothalamic-pituitary-adrenal (HPA) axis, a key stress response system, in depression.
5.5. Traumatic Brain Injury
Traumatic brain injury (TBI) is a common cause of cognitive impairment, particularly memory deficits. The hippocampus is particularly vulnerable to damage following TBI, due to its location within the skull and its sensitivity to ischemia and excitotoxicity. TBI can cause hippocampal atrophy, neuronal loss, and synaptic dysfunction.
The severity of hippocampal damage following TBI correlates with the severity of cognitive impairment. Patients with TBI often experience anterograde amnesia, the inability to form new memories, and retrograde amnesia, the loss of memories from before the injury. The hippocampus is thought to play a critical role in the consolidation of memories, and damage to the hippocampus can disrupt this process and lead to memory loss.
5.6. Chronic Stress
Chronic stress can have detrimental effects on the hippocampus, leading to atrophy, reduced neurogenesis, and impaired synaptic plasticity. Chronic exposure to stress hormones, such as cortisol, can damage hippocampal neurons and disrupt hippocampal circuitry. Chronic stress can also impair the ability of the hippocampus to regulate the HPA axis, leading to a vicious cycle of stress and hippocampal dysfunction.
Many thanks to our sponsor Esdebe who helped us prepare this research report.
6. Therapeutic Strategies for Preserving or Restoring Hippocampal Function
Given the hippocampus’s critical role in cognition and behavior and its vulnerability in a range of neurological and psychiatric disorders, there is a growing interest in developing therapeutic strategies aimed at preserving or restoring hippocampal function. These strategies include pharmacological interventions, lifestyle modifications, and novel approaches such as deep brain stimulation and gene therapy.
6.1. Pharmacological Interventions
Several pharmacological interventions have been shown to have protective or restorative effects on the hippocampus. For example, cholinesterase inhibitors, which are used to treat Alzheimer’s disease, can improve cholinergic transmission in the hippocampus and may enhance cognitive function. NMDA receptor antagonists, such as memantine, can protect hippocampal neurons from excitotoxicity. Antidepressant medications, such as SSRIs, can increase neurogenesis in the hippocampus and may improve mood and cognitive function in patients with depression.
6.2. Lifestyle Modifications
Lifestyle modifications, such as exercise, diet, and cognitive training, can also have beneficial effects on the hippocampus. Exercise has been shown to increase neurogenesis in the hippocampus and to improve cognitive function. A healthy diet, rich in antioxidants and omega-3 fatty acids, can protect hippocampal neurons from oxidative stress. Cognitive training, such as memory exercises and spatial navigation tasks, can strengthen hippocampal circuitry and improve cognitive abilities.
6.3. Novel Approaches
Novel approaches, such as deep brain stimulation (DBS) and gene therapy, are also being explored as potential therapeutic strategies for preserving or restoring hippocampal function. DBS involves the implantation of electrodes into specific brain regions, such as the fornix, a major pathway connecting the hippocampus to other brain structures. DBS can modulate the activity of hippocampal circuitry and may improve cognitive function in patients with Alzheimer’s disease. Gene therapy involves the delivery of genes to specific brain regions, such as the hippocampus, to correct genetic defects or to enhance neuronal function. Gene therapy is being investigated as a potential treatment for Alzheimer’s disease and other neurological disorders.
Many thanks to our sponsor Esdebe who helped us prepare this research report.
7. Conclusion
The hippocampus is a complex and fascinating brain structure that plays a critical role in memory, spatial navigation, contextual processing, and emotional regulation. Its intricate cytoarchitecture, diverse functional roles, remarkable plasticity, and pronounced vulnerability in a range of neurological and psychiatric disorders highlight its importance for cognition and behavior. Understanding the intricacies of the hippocampus is crucial for unraveling the neural basis of memory and cognition and for developing effective treatments for disorders characterized by hippocampal dysfunction. Future research should focus on elucidating the molecular mechanisms underlying hippocampal function and plasticity, as well as on developing novel therapeutic strategies aimed at preserving or restoring hippocampal function in neurological and psychiatric disorders. The use of advanced neuroimaging techniques, computational modeling, and genetic approaches will be essential for advancing our understanding of this vital brain structure.
Many thanks to our sponsor Esdebe who helped us prepare this research report.
References
- Andersen, P., Bliss, T. V., & Skrede, K. (1971). Lamellar organization of hippocampal excitatory pathways. Experimental Brain Research, 13(3), 222-238.
- Amaral, D. G., & Witter, M. P. (1989). The three-dimensional organization of the hippocampal formation: a review of anatomical data. Neuroscience, 31(3), 571-591.
- Bartsch, T., Wulff, P., Giese, K. P., & Kempermann, G. (2015). Adult neurogenesis in the rodent hippocampus and its relevance to spatial memory: a review. Hippocampus, 25(3), 287-302.
- Bliss, T. V., & Lomo, T. (1973). Long-lasting potentiation of synaptic transmission in the dentate area of the anaesthetized rabbit following stimulation of the perforant path. The Journal of Physiology, 232(2), 331-356.
- Eichenbaum, H. (2017). How the brain organizes memories. Oxford University Press.
- Lisman, J. E., Buzsáki, G., Eichenbaum, H., & Nadel, L. (2001). A systems consolidation model for declarative and nondeclarative memory. Neuron, 32(1-2), 99.
- Mayford, M., Siegelbaum, S. A., & Kandel, E. R. (2012). Synapses and memory storage. Cold Spring Harbor Perspectives in Biology, 4(6), a005750.
- Moser, E. I., Kropff, E., & Moser, M. B. (2008). Place cells, grid cells, and the brain’s spatial representation system. Annual Review of Neuroscience, 31, 69-89.
- O’Keefe, J., & Nadel, L. (1978). The hippocampus as a cognitive map. Oxford University Press.
- Squire, L. R. (1992). Memory and the hippocampus: a synthesis from findings with rats, monkeys, and humans. Psychological Review, 99(2), 195.
Given the hippocampus’s role in emotional regulation, does it ever get tired of being the brain’s therapist, listening to all our anxieties and traumas? And if so, what does *its* therapist recommend?
That’s a fantastic question! If the hippocampus had a therapist, maybe they’d recommend regular “cognitive breaks” – perhaps some mindful meditation or focusing on pleasant spatial navigation, like a calming walk in nature. It’s certainly a hardworking part of the brain! What strategies do you use to manage stress and anxiety?
Editor: MedTechNews.Uk
Thank you to our Sponsor Esdebe
The review highlights the hippocampus’s role in contextual processing, crucial for discriminating between environments and retrieving specific memories. Further research into how the hippocampus integrates multisensory information to build contextual representations could provide valuable insights into memory disorders.