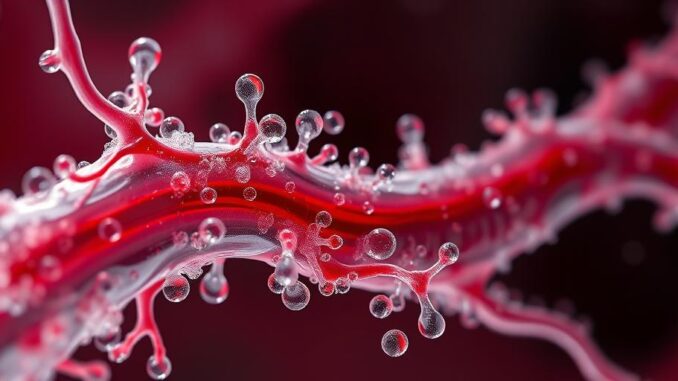
The Interplay of Microplastics, Atherosclerosis, and Stroke: A Comprehensive Review of Plaque Pathogenesis, Progression, and Management Strategies
Abstract
Atherosclerosis, characterized by the accumulation of plaque within arterial walls, remains a leading cause of cardiovascular morbidity and mortality globally. The pathophysiology of atherosclerosis is complex and multifactorial, involving lipid deposition, inflammation, oxidative stress, and smooth muscle cell proliferation. Recent evidence suggests a potentially significant, yet under-investigated, role for environmental pollutants, particularly microplastics, in exacerbating atherosclerotic plaque formation and instability. This review comprehensively examines the multifaceted aspects of atherosclerosis, including plaque formation, composition, and progression, focusing on the interplay between microplastic exposure and heightened plaque vulnerability, particularly in individuals with a history of stroke. Furthermore, we delve into the critical roles of inflammation and oxidative stress in destabilizing plaques, leading to rupture and subsequent thromboembolic events. Diagnostic modalities for assessing plaque burden and vulnerability are also critically evaluated, alongside a detailed analysis of current therapeutic strategies, such as statins, antiplatelet agents, and lifestyle modifications, aimed at preventing and managing atherosclerotic disease and mitigating cardiovascular events. Finally, we highlight the knowledge gaps and future research directions imperative for further elucidating the impact of microplastics on atherosclerotic disease and developing targeted prevention and therapeutic strategies.
Many thanks to our sponsor Esdebe who helped us prepare this research report.
1. Introduction
Atherosclerosis is a chronic, progressive disease characterized by the buildup of plaques within the arterial walls, ultimately leading to narrowing of the arterial lumen and restriction of blood flow. This process, traditionally understood to be driven by lipid accumulation and inflammation, has been the subject of intense research for decades. The resulting consequences, including myocardial infarction, stroke, and peripheral artery disease, are major contributors to global mortality and morbidity. While established risk factors such as hyperlipidemia, hypertension, smoking, and diabetes mellitus are well-recognized, emerging evidence points toward the potential influence of environmental factors, specifically microplastic pollution, on the development and progression of atherosclerotic disease.
Microplastics, defined as plastic particles smaller than 5 mm, are ubiquitous environmental contaminants found in air, water, and food sources. Human exposure to microplastics is now widespread, with detectable levels found in blood, lungs, and even the placenta. The potential health effects of microplastic exposure are a growing concern, with studies indicating that these particles can induce inflammation, oxidative stress, and cellular toxicity in various tissues. Recent research has suggested a link between microplastic exposure and increased atherosclerotic plaque burden, particularly in individuals with a history of stroke. This association warrants further investigation to elucidate the underlying mechanisms and assess the potential public health implications.
This review aims to provide a comprehensive overview of the pathophysiology of atherosclerosis, focusing on the role of microplastics in exacerbating plaque formation and instability. We will delve into the formation, composition, and progression of atherosclerotic plaques, the contributions of inflammation and oxidative stress to plaque rupture, and the diagnostic methods used to assess plaque burden and vulnerability. Furthermore, we will discuss current therapeutic strategies for preventing and managing atherosclerotic disease and mitigating cardiovascular events, while emphasizing the potential implications of microplastic exposure on treatment efficacy. Finally, we will identify key knowledge gaps and suggest future research directions needed to better understand the complex interplay between microplastics, atherosclerosis, and cardiovascular health.
Many thanks to our sponsor Esdebe who helped us prepare this research report.
2. Formation, Composition, and Progression of Atherosclerotic Plaque
The development of atherosclerotic plaque is a complex process that begins with endothelial dysfunction. The endothelium, the inner lining of blood vessels, plays a crucial role in maintaining vascular homeostasis by regulating vascular tone, preventing platelet aggregation, and inhibiting leukocyte adhesion. Damage to the endothelium, often caused by risk factors such as hypertension, hyperlipidemia, and smoking, increases permeability to lipoproteins, particularly low-density lipoprotein (LDL). LDL particles accumulate in the subendothelial space, where they undergo oxidation, leading to the formation of oxidized LDL (oxLDL). OxLDL is highly atherogenic and triggers an inflammatory response by activating endothelial cells and recruiting monocytes.
Recruited monocytes differentiate into macrophages, which engulf oxLDL, transforming into foam cells. Foam cells accumulate in the intima, forming fatty streaks, the earliest visible lesions of atherosclerosis. As the disease progresses, smooth muscle cells migrate from the media to the intima and proliferate, contributing to the growth and complexity of the plaque. These smooth muscle cells synthesize extracellular matrix components, such as collagen, elastin, and proteoglycans, which provide structural support to the plaque.
Over time, the plaque evolves into a more complex structure, characterized by a lipid-rich core, a fibrous cap, and an inflammatory infiltrate. The lipid-rich core contains accumulated cholesterol, cholesterol esters, and cellular debris, including dead foam cells. The fibrous cap, composed primarily of collagen and smooth muscle cells, provides structural stability to the plaque and prevents its rupture. However, ongoing inflammation and proteolytic activity can weaken the fibrous cap, making the plaque vulnerable to rupture. The composition of the plaque, particularly the ratio of lipid core to fibrous cap thickness, is a critical determinant of plaque stability. Plaques with large lipid cores and thin fibrous caps are considered high-risk plaques, prone to rupture and subsequent thrombotic events.
The progression of atherosclerosis is not linear but rather a dynamic process characterized by periods of quiescence and periods of rapid growth and instability. Factors that contribute to plaque progression include persistent inflammation, continued lipid accumulation, and repetitive injury to the endothelium. The presence of microplastics may further accelerate plaque progression by exacerbating inflammation and oxidative stress, as discussed in subsequent sections.
Many thanks to our sponsor Esdebe who helped us prepare this research report.
3. The Role of Inflammation and Oxidative Stress in Plaque Instability and Rupture
Inflammation plays a pivotal role in all stages of atherosclerosis, from initiation to progression and ultimately, rupture. The inflammatory response is orchestrated by a complex network of immune cells, including macrophages, T lymphocytes, and mast cells, which release a variety of pro-inflammatory cytokines and chemokines. These mediators amplify the inflammatory cascade, promoting leukocyte recruitment, endothelial activation, and smooth muscle cell proliferation.
Within the atherosclerotic plaque, inflammation contributes to plaque instability by promoting the degradation of the fibrous cap. Macrophages and other immune cells secrete matrix metalloproteinases (MMPs), a family of enzymes that degrade collagen and other components of the extracellular matrix. Increased MMP activity weakens the fibrous cap, making it more susceptible to rupture. Furthermore, inflammatory cytokines such as interferon-gamma (IFN-γ) can inhibit collagen synthesis by smooth muscle cells, further compromising the integrity of the fibrous cap.
Oxidative stress, characterized by an imbalance between the production of reactive oxygen species (ROS) and antioxidant defenses, is another key contributor to plaque instability. ROS can modify LDL particles, leading to the formation of oxLDL, which is a potent inducer of inflammation and foam cell formation. Furthermore, ROS can directly damage endothelial cells, contributing to endothelial dysfunction and increased permeability. Oxidative stress also promotes the activation of MMPs and inhibits collagen synthesis, further contributing to plaque instability.
The interplay between inflammation and oxidative stress creates a vicious cycle that accelerates plaque progression and increases the risk of rupture. Microplastic exposure may further exacerbate this cycle by inducing oxidative stress and activating inflammatory pathways. Studies have shown that microplastics can trigger the production of ROS in various cell types, including macrophages and endothelial cells. Furthermore, microplastics can activate the inflammasome, a multi-protein complex that promotes the release of pro-inflammatory cytokines such as interleukin-1β (IL-1β). The release of IL-1β can further amplify the inflammatory response and contribute to plaque instability. The surface properties and chemical composition of the microplastics also influences the level of inflammation that is triggered.
Many thanks to our sponsor Esdebe who helped us prepare this research report.
4. Diagnostic Methods for Assessing Plaque Burden and Vulnerability
Accurate assessment of plaque burden and vulnerability is crucial for identifying individuals at high risk of cardiovascular events and for guiding therapeutic interventions. A variety of diagnostic methods are available, each with its own strengths and limitations. These methods can be broadly categorized into non-invasive and invasive techniques.
4.1 Non-Invasive Techniques
-
Carotid Ultrasound: This widely available and relatively inexpensive technique uses sound waves to visualize the carotid arteries and measure the thickness of the intima-media complex (IMT). Increased IMT is an early marker of atherosclerosis and is associated with an increased risk of cardiovascular events. Carotid ultrasound can also detect the presence of plaque and assess its size and morphology. However, carotid ultrasound provides limited information about plaque composition and vulnerability.
-
Computed Tomography Angiography (CTA): CTA uses X-rays and contrast dye to visualize the coronary and carotid arteries. CTA can accurately quantify plaque burden and identify areas of stenosis. Recent advances in CTA technology allow for the assessment of plaque composition, including the presence of calcium and lipid-rich necrotic core. However, CTA involves radiation exposure and the use of contrast dye, which can be harmful to individuals with kidney disease.
-
Magnetic Resonance Angiography (MRA): MRA uses magnetic fields and radio waves to visualize the arteries. MRA is particularly useful for assessing the carotid arteries and can provide information about plaque composition, including the presence of lipid-rich necrotic core and intraplaque hemorrhage. MRA does not involve radiation exposure but can be more expensive and time-consuming than CTA.
-
Positron Emission Tomography (PET): PET imaging uses radioactive tracers to detect areas of increased metabolic activity. [18F]-fluorodeoxyglucose (FDG) PET can be used to assess inflammation in atherosclerotic plaques. Increased FDG uptake is associated with plaque instability and an increased risk of cardiovascular events. However, PET imaging is expensive and requires specialized equipment.
4.2 Invasive Techniques
-
Coronary Angiography: Coronary angiography, also known as cardiac catheterization, involves inserting a catheter into an artery and injecting contrast dye to visualize the coronary arteries. Coronary angiography is the gold standard for assessing coronary artery stenosis and is often used to guide percutaneous coronary intervention (PCI). However, coronary angiography provides limited information about plaque composition and vulnerability.
-
Intravascular Ultrasound (IVUS): IVUS involves inserting a small ultrasound probe into the coronary artery to visualize the vessel wall. IVUS can provide detailed information about plaque size, morphology, and composition. IVUS is particularly useful for assessing the lipid-rich necrotic core and the thickness of the fibrous cap.
-
Optical Coherence Tomography (OCT): OCT uses light waves to create high-resolution images of the coronary artery wall. OCT can provide even more detailed information about plaque morphology and composition than IVUS. OCT is particularly useful for assessing fibrous cap thickness and identifying thin-cap fibroatheromas, which are considered high-risk plaques.
The choice of diagnostic method depends on several factors, including the clinical question, the patient’s risk factors, and the availability of resources. Non-invasive techniques are generally preferred for screening and risk stratification, while invasive techniques are typically reserved for patients with known or suspected coronary artery disease. The use of advanced imaging techniques, such as CTA, MRA, IVUS, and OCT, can provide valuable information about plaque composition and vulnerability, allowing for more personalized and targeted therapeutic interventions. Furthermore, the development of novel biomarkers for assessing plaque instability, such as circulating microRNAs and inflammatory mediators, holds promise for improving risk prediction and guiding treatment strategies.
Many thanks to our sponsor Esdebe who helped us prepare this research report.
5. Therapeutic Strategies for Preventing and Managing Atherosclerotic Plaque and Cardiovascular Events
The primary goal of therapeutic strategies for atherosclerosis is to prevent or slow the progression of plaque formation, stabilize existing plaques, and reduce the risk of cardiovascular events. These strategies can be broadly categorized into lifestyle modifications, pharmacological interventions, and revascularization procedures.
5.1 Lifestyle Modifications
Lifestyle modifications are the cornerstone of atherosclerosis prevention and management. These include:
-
Dietary Changes: A heart-healthy diet, rich in fruits, vegetables, whole grains, and lean protein, and low in saturated and trans fats, cholesterol, and sodium, can help lower LDL cholesterol levels, reduce inflammation, and improve overall cardiovascular health. The Mediterranean diet, in particular, has been shown to be beneficial in reducing the risk of cardiovascular events.
-
Regular Exercise: Regular physical activity, such as aerobic exercise and strength training, can help lower LDL cholesterol levels, raise HDL cholesterol levels, improve blood pressure, and reduce insulin resistance. Aim for at least 150 minutes of moderate-intensity or 75 minutes of vigorous-intensity aerobic exercise per week.
-
Smoking Cessation: Smoking is a major risk factor for atherosclerosis and significantly increases the risk of cardiovascular events. Quitting smoking is one of the most important things individuals can do to improve their cardiovascular health.
-
Weight Management: Maintaining a healthy weight can help lower LDL cholesterol levels, improve blood pressure, and reduce insulin resistance.
5.2 Pharmacological Interventions
-
Statins: Statins are the most widely used medications for lowering LDL cholesterol levels. Statins inhibit HMG-CoA reductase, an enzyme involved in cholesterol synthesis. Statins have been shown to reduce the risk of cardiovascular events in individuals with and without known coronary artery disease. They also have pleiotropic effects, including reducing inflammation and improving endothelial function.
-
Antiplatelet Agents: Antiplatelet agents, such as aspirin and clopidogrel, inhibit platelet aggregation and reduce the risk of thrombus formation. Antiplatelet agents are commonly used in individuals with known coronary artery disease to prevent myocardial infarction and stroke.
-
Anticoagulants: Anticoagulants, such as warfarin and direct oral anticoagulants (DOACs), prevent blood clot formation. Anticoagulants are used in individuals with atrial fibrillation, venous thromboembolism, and other conditions that increase the risk of blood clots.
-
ACE Inhibitors and Angiotensin Receptor Blockers (ARBs): ACE inhibitors and ARBs lower blood pressure by blocking the renin-angiotensin-aldosterone system (RAAS). ACE inhibitors and ARBs have been shown to reduce the risk of cardiovascular events in individuals with hypertension, heart failure, and diabetes.
-
Beta-Blockers: Beta-blockers lower blood pressure and heart rate by blocking the effects of adrenaline. Beta-blockers are used in individuals with hypertension, angina, and heart failure.
-
PCSK9 Inhibitors: PCSK9 inhibitors are a newer class of medications that lower LDL cholesterol levels by inhibiting proprotein convertase subtilisin/kexin type 9 (PCSK9), an enzyme that degrades LDL receptors. PCSK9 inhibitors are highly effective at lowering LDL cholesterol levels and have been shown to reduce the risk of cardiovascular events in individuals at high risk.
5.3 Revascularization Procedures
-
Percutaneous Coronary Intervention (PCI): PCI involves inserting a catheter into the coronary artery and inflating a balloon to open up the blocked artery. A stent, a small metal mesh tube, is often placed in the artery to keep it open.
-
Coronary Artery Bypass Grafting (CABG): CABG involves surgically grafting a blood vessel from another part of the body to bypass the blocked coronary artery.
The choice of therapeutic strategy depends on several factors, including the severity of the atherosclerosis, the patient’s risk factors, and the presence of other medical conditions. Lifestyle modifications are essential for all individuals with atherosclerosis. Pharmacological interventions are often used in conjunction with lifestyle modifications to further reduce the risk of cardiovascular events. Revascularization procedures are reserved for individuals with severe coronary artery disease who are not responding to medical therapy.
The potential impact of microplastic exposure on the efficacy of these therapeutic strategies remains largely unknown. It is conceivable that microplastics could interfere with the absorption or metabolism of statins or other medications, reducing their effectiveness. Furthermore, the pro-inflammatory and pro-oxidative effects of microplastics could counteract the beneficial effects of lifestyle modifications and pharmacological interventions. Therefore, further research is needed to assess the impact of microplastic exposure on the efficacy of current therapeutic strategies for atherosclerosis.
Many thanks to our sponsor Esdebe who helped us prepare this research report.
6. Microplastics and Atherosclerosis: Evidence and Mechanisms
The emerging evidence linking microplastic exposure to adverse cardiovascular effects, particularly in the context of atherosclerosis, is a cause for concern. The mechanisms by which microplastics might exacerbate atherosclerosis are complex and likely involve a combination of direct and indirect effects.
6.1 Direct Effects
-
Endothelial Dysfunction: Microplastics can directly interact with endothelial cells, disrupting their function and increasing permeability. This can promote the influx of LDL particles into the subendothelial space, initiating the atherosclerotic process. Some studies suggest that certain types of microplastics are more toxic to endothelial cells than others, depending on their size, shape, and chemical composition.
-
Inflammation: Microplastics can trigger inflammatory responses in various cell types, including macrophages, endothelial cells, and smooth muscle cells. This can lead to the release of pro-inflammatory cytokines and chemokines, which contribute to plaque instability and rupture. The surface characteristics and chemical composition of microplastics play a significant role in determining the extent of the inflammatory response.
-
Oxidative Stress: Microplastics can induce oxidative stress by generating ROS and impairing antioxidant defenses. Oxidative stress can modify LDL particles, leading to the formation of oxLDL, which is a potent inducer of inflammation and foam cell formation.
6.2 Indirect Effects
-
Adsorption and Transport of Toxic Chemicals: Microplastics can adsorb and transport a variety of toxic chemicals, including persistent organic pollutants (POPs) and heavy metals. These chemicals can leach from the microplastics and exert toxic effects on cells and tissues, further contributing to the development of atherosclerosis.
-
Gut Microbiome Disruption: Microplastic exposure can disrupt the gut microbiome, leading to increased intestinal permeability and systemic inflammation. Changes in the gut microbiome composition have been linked to increased risk of cardiovascular disease.
-
Immune System Modulation: Microplastics can modulate the immune system, potentially leading to chronic inflammation and an increased risk of autoimmune diseases. Chronic inflammation is a major driver of atherosclerosis.
The recent study linking microplastics to increased plaque burden in individuals with a history of stroke provides further evidence of the potential cardiovascular risks associated with microplastic exposure. While the exact mechanisms underlying this association remain to be elucidated, the study highlights the need for further research to assess the impact of microplastics on atherosclerotic disease and cardiovascular health.
Many thanks to our sponsor Esdebe who helped us prepare this research report.
7. Future Research Directions and Knowledge Gaps
Despite the growing body of evidence linking microplastics to adverse cardiovascular effects, several knowledge gaps remain. Future research should focus on the following areas:
-
Quantifying Microplastic Exposure: Developing accurate and reliable methods for quantifying microplastic exposure in humans is crucial for assessing the true extent of the problem and for conducting epidemiological studies. Current methods for measuring microplastic levels in biological samples are limited and require further refinement.
-
Elucidating Mechanisms of Action: Further research is needed to elucidate the precise mechanisms by which microplastics exacerbate atherosclerosis. This includes investigating the effects of different types of microplastics on endothelial function, inflammation, oxidative stress, and plaque stability. In vitro and in vivo studies are needed to better understand the cellular and molecular mechanisms involved.
-
Assessing Long-Term Health Effects: Longitudinal studies are needed to assess the long-term health effects of microplastic exposure, particularly on cardiovascular health. These studies should examine the relationship between microplastic exposure and the incidence of cardiovascular events, such as myocardial infarction, stroke, and peripheral artery disease.
-
Developing Mitigation Strategies: Strategies are needed to reduce microplastic pollution and minimize human exposure. This includes reducing plastic production, improving waste management practices, and developing biodegradable alternatives to plastic. Furthermore, research is needed to identify and develop interventions to mitigate the adverse health effects of microplastic exposure.
-
Impact on Therapeutic Efficacy: It is crucial to investigate if microplastic exposure alters the efficacy of medications used to treat and prevent cardiovascular disease. The effects of microplastics on drug metabolism, absorption, and distribution need to be carefully assessed.
Addressing these knowledge gaps will require a multidisciplinary approach involving environmental scientists, toxicologists, cardiologists, and epidemiologists. By working together, we can better understand the potential health risks associated with microplastic exposure and develop effective strategies to protect human health.
Many thanks to our sponsor Esdebe who helped us prepare this research report.
8. Conclusion
Atherosclerosis remains a significant global health challenge, and emerging evidence suggests that microplastic pollution may contribute to its pathogenesis and progression. The complex interplay between microplastics, inflammation, oxidative stress, and plaque instability warrants further investigation. While current therapeutic strategies effectively manage atherosclerotic disease and reduce cardiovascular events, the potential impact of microplastic exposure on treatment efficacy remains largely unknown. Addressing the knowledge gaps through multidisciplinary research and developing effective mitigation strategies are crucial for protecting human health and mitigating the cardiovascular risks associated with microplastic pollution.
Many thanks to our sponsor Esdebe who helped us prepare this research report.
References
-
New England Journal of Medicine – Identification of Plastic Nanoparticles in Human Atherosclerotic Plaques
https://www.nejm.org/doi/full/10.1056/NEJMc2300582 -
Vethaak AD, Legradi J, de Voogt P, et al. Microplastics as a vector for chemicals in the aquatic environment: A state-of-the-art review. Environmental Toxicology and Chemistry. 2011;30(12):2783-2799.
-
Wright SL, Kelly FJ. Plastic and Human Health: A Microscopic Review. Wiley Interdisciplinary Reviews: Water. 2017;4(6):e1268.
-
Lambert S, Wagner M. Environmental performance of bio-based and biodegradable plastics: the road ahead. Chemical Society Reviews. 2017;46(22):6855-6871.
-
Rossi G, Barni S, Brandelli A, et al. Ecotoxicity of microplastics: what do we know and what should we investigate?. Environmental Pollution. 2019;245:469-480.
-
Landrigan PJ, Fuller R, Acosta NJR, et al. The Lancet Commission on pollution and health. The Lancet. 2018;391(10119):462-512.
-
Goldstein MC, Rosenberg M, Cheng L. Increased oceanic microplastic debris enhances oviposition in an endemic pelagic insect. Biology Letters. 2012;8(5):817-820.
-
Hahladakis JN, Velis CA, Weber R, Iacovidou E, Pappa S, Gidarakos E. An overview of chemical additives present in plastics: Migration, release, fate and environmental impact during their use, disposal and recycling. Journal of Hazardous Materials. 2018;344:179-199.
-
Boucher J, Friot D. Primary Microplastics in the Oceans: A Global Evaluation of Sources. Gland, Switzerland: IUCN; 2017.
-
da Costa JP, Santos PS, Duarte AC, Rocha-Santos T. (Nano)plastics in the environment–Sources, fates and effects. Science of The Total Environment. 2016;566-567:15-26.
-
Eriksen M, Lebreton LCM, Carson HS, et al. Plastic pollution in the world’s oceans: more than 5 trillion plastic pieces weighing over 250,000 tons afloat at sea. PLoS One. 2014;9(12):e111913.
-
Andrady AL. Microplastics in the marine environment. Marine Pollution Bulletin. 2011;62(8):1596-1605.
-
Avogadro A, Banfi G, Vergani L. Atherosclerosis: role of oxidation and inflammation. Nutrition, Metabolism and Cardiovascular Diseases. 2005;15(5):369-377.
-
Libby P, Ridker PM, Hansson GK. Inflammation in atherosclerosis. Circulation. 2009;119(3):436-444.
-
Hansson GK, Robertson AK, Söderberg-Nauclér C. Inflammation and atherosclerosis. Annual Review of Immunology. 2006;24:225-255.
-
Lusis AJ. Atherosclerosis. Nature. 2000;407(6801):233-241.
-
Moore KJ, Freeman MW. Scavenger receptors in atherosclerosis. Current Opinion in Lipidology. 2006;17(5):489-495.
-
Libby P. The changing landscape of atherosclerosis. Nature. 2021;592(7855):524-533.
-
Libby P. Inflammation in atherosclerosis: from pathophysiology to practice. Journal of the American College of Cardiology. 2021;77(13):1675-1689.
-
Rajagopalan S, Brook RD, Rubenfire M, et al. Air pollution and atherosclerosis: a review. Atherosclerosis. 2003;166(2):279-290.
-
Valavanidis A, Vlachogianni T, Dassenakis M, Scoullos M. Occurrence and impact of microplastics in the marine environment. Journal of Hazardous Materials. 2016;318:257-275.
-
Revel M, Châtel A, Mouneyrac C. Micro(nano)plastics: A threat to human health?. Current Opinion in Environmental Science & Health. 2018;4:17-23.
Microplastics contributing to atherosclerosis? So, besides dodging dietary cholesterol, now we need to worry about filtering our blood for rogue plastic particles? Suddenly, my Brita filter seems inadequate for the task at hand. Is there a premium model with microplastic filtration I should be looking into?
That’s a great point! It really highlights how widespread microplastic exposure is becoming. While a standard Brita might not catch everything, research into advanced filtration systems for drinking water and even air purification is definitely gaining momentum. It will be interesting to see what innovative solutions emerge. Thanks for your comment!
Editor: MedTechNews.Uk
Thank you to our Sponsor Esdebe
This comprehensive review highlights a critical emerging concern. Investigating the potential impact of varying microplastic compositions (e.g., polyethylene vs. PVC) on plaque vulnerability and inflammatory responses could yield valuable insights for targeted preventative measures.
Thank you for your insightful comment! I agree that understanding the specific impact of different microplastic types is crucial. Future research focusing on the varying toxicity and inflammatory potential of materials like polyethylene and PVC could significantly advance our preventative strategies against atherosclerosis. This is something we hope to address in future studies.
Editor: MedTechNews.Uk
Thank you to our Sponsor Esdebe