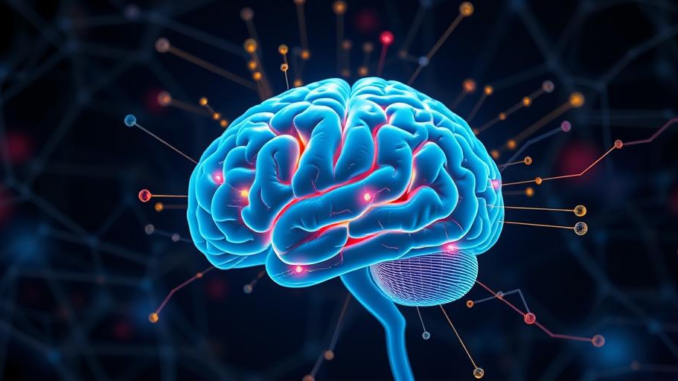
The Intricate Dance of Early Brain Development: Influences, Vulnerabilities, and Functional Implications
Abstract
The developing human brain undergoes a period of rapid and intricate structural and functional maturation during the prenatal and early postnatal periods. This period is exquisitely sensitive to a range of influences, both genetic and environmental, that can significantly shape neurodevelopmental trajectories. This review delves into the critical stages of early brain development, highlighting the intricate processes of neurogenesis, migration, differentiation, synaptogenesis, and myelination. We examine the impact of various factors on brain structure, including genetic predispositions, exposure to teratogens (such as viruses and alcohol), maternal stress, and socioeconomic disparities. The report emphasizes the significance of gray matter volume and cortical folding as key indices of brain maturation and function. We evaluate the methodologies employed to study infant brain structure, focusing on the applications and limitations of magnetic resonance imaging (MRI) techniques. Furthermore, we explore the long-term cognitive and behavioral outcomes associated with specific structural brain differences observed in infancy, emphasizing the challenges in establishing direct causal links. This review aims to provide a comprehensive overview of the complexities of early brain development, highlighting the importance of early interventions to mitigate the impact of adverse exposures and optimize neurodevelopmental outcomes.
1. Introduction
The human brain, arguably the most complex structure in the known universe, undergoes a remarkable journey of development, particularly during the prenatal and early postnatal periods. This period, characterized by rapid neurogenesis, migration, differentiation, synaptogenesis, and myelination, sets the foundation for lifelong cognitive, emotional, and behavioral functioning. The intricate dance of these processes is exquisitely sensitive to a myriad of influences, making the developing brain vulnerable to disruption and shaping its trajectory in profound ways.
Understanding the complexities of early brain development is crucial for several reasons. First, it provides a framework for identifying individuals at risk for neurodevelopmental disorders. Second, it offers insights into the etiology of these disorders, paving the way for targeted interventions. Third, it informs public health policies aimed at promoting optimal brain development in all children.
This review aims to provide a comprehensive overview of early brain development, focusing on the factors that can influence its trajectory and the long-term consequences of atypical development. We will delve into the key stages of brain development, discuss the impact of genetic and environmental factors, examine the methodologies used to study infant brain structure, and explore the cognitive and behavioral outcomes associated with specific structural differences.
2. Milestones in Early Brain Development
The development of the human brain is a continuous and dynamic process that begins shortly after conception and extends well into adulthood. However, the prenatal and early postnatal periods are particularly critical, characterized by a rapid pace of neurodevelopment. Several key milestones mark this period, each playing a crucial role in shaping the mature brain.
2.1 Neurogenesis and Neural Tube Formation: The initial stages of brain development involve the formation of the neural tube, the precursor to the central nervous system. This process begins around the third week of gestation and is highly susceptible to disruption by factors such as folate deficiency. Following neural tube closure, neurogenesis, the birth of new neurons, commences. This process is especially prominent in the ventricular and subventricular zones, where neural stem cells reside. Neurogenesis generates a vast overproduction of neurons, exceeding the number ultimately required for the adult brain. This overproduction is followed by programmed cell death (apoptosis), a crucial process for sculpting neural circuits.
2.2 Neuronal Migration: Once generated, neurons must migrate to their final destinations within the brain. This process is guided by a complex interplay of chemical signals and cellular interactions. Radial glial cells, acting as scaffolding, guide migrating neurons from the ventricular zone to the cortical plate. Disruptions in neuronal migration can lead to a variety of neurodevelopmental disorders, including lissencephaly (smooth brain) and heterotopias (clusters of neurons in abnormal locations).
2.3 Differentiation and Synaptogenesis: After reaching their final destinations, neurons begin to differentiate, acquiring specific morphological and functional characteristics. This process is influenced by both genetic programming and environmental cues. Simultaneously, neurons begin to form synapses, the connections through which they communicate with each other. Synaptogenesis is a particularly rapid process in the early postnatal period, leading to a dramatic increase in the number of synapses in the brain. This period of synaptic exuberance is followed by synaptic pruning, a process in which unused or weak synapses are eliminated, refining neural circuits and increasing efficiency.
2.4 Myelination: Myelination, the process of coating nerve fibers with myelin, a fatty substance that insulates axons and increases the speed of nerve impulse transmission, is another critical developmental process. Myelination begins prenatally and continues well into adulthood. The timing and progression of myelination are highly specific to different brain regions, with sensory and motor areas myelinated earlier than higher-order cognitive areas. Disruptions in myelination can lead to impaired neural communication and contribute to a variety of neurological and psychiatric disorders.
2.5 Cortical Folding: Cortical folding, or gyrification, significantly increases the surface area of the cerebral cortex, allowing for greater neural density and computational power. The degree of cortical folding increases dramatically during the third trimester of gestation and early postnatal period. Variations in cortical folding patterns have been associated with cognitive abilities and susceptibility to neurological disorders.
Understanding these developmental milestones and their potential vulnerabilities is essential for identifying early indicators of atypical brain development and for developing targeted interventions to mitigate the impact of adverse exposures.
3. Factors Influencing Early Brain Structure
The developing brain is highly susceptible to a range of influences, both genetic and environmental, that can significantly shape its structure and function. These influences can act at different stages of development, leading to a variety of neurodevelopmental outcomes.
3.1 Genetic Factors: Genetic factors play a fundamental role in determining the basic architecture of the brain. Genes encode the instructions for cell proliferation, migration, differentiation, and synapse formation. Mutations in these genes can disrupt these processes, leading to a variety of neurodevelopmental disorders. For example, mutations in genes involved in neuronal migration can cause lissencephaly or heterotopias. Furthermore, genetic predispositions can interact with environmental factors to increase susceptibility to certain disorders.
3.2 Teratogens: Teratogens are substances that can cause birth defects. Exposure to teratogens during prenatal development can have devastating effects on brain structure and function. Alcohol, for example, is a well-known teratogen that can cause fetal alcohol spectrum disorders (FASDs), characterized by a range of physical, cognitive, and behavioral impairments. Other teratogens include certain medications, drugs, and environmental toxins. The timing and duration of exposure to teratogens are critical factors determining the severity of the effects.
3.3 Maternal Stress: Maternal stress during pregnancy can also have a significant impact on fetal brain development. Stress hormones, such as cortisol, can cross the placenta and affect fetal brain development. Chronic maternal stress has been associated with reduced gray matter volume, altered cortical folding, and increased risk of behavioral problems in offspring. The mechanisms underlying these effects are complex and likely involve epigenetic modifications, which alter gene expression without changing the underlying DNA sequence.
3.4 Maternal Immune Activation (MIA): Maternal immune activation (MIA), triggered by infection or inflammation during pregnancy, can also impact fetal brain development. Elevated levels of inflammatory cytokines can cross the placenta and disrupt neuronal migration, differentiation, and synapse formation. MIA has been linked to an increased risk of neurodevelopmental disorders, including autism spectrum disorder (ASD) and schizophrenia. The specific effects of MIA depend on the timing, severity, and type of infection.
3.5 Socioeconomic Factors: Socioeconomic disparities can also influence brain development. Children from low-income families are more likely to experience chronic stress, poor nutrition, and exposure to environmental toxins, all of which can negatively impact brain structure and function. These factors can contribute to disparities in cognitive and academic achievement.
3.6 Viral Infections (including COVID-19): Prenatal exposure to viral infections, including SARS-CoV-2 (the virus that causes COVID-19), has emerged as a potential risk factor for altered neurodevelopment. While the direct impact of SARS-CoV-2 on the fetal brain is still under investigation, studies have suggested that maternal COVID-19 infection during pregnancy may be associated with changes in newborn brain structure, particularly in gray matter volume and cortical folding [1,2]. It is hypothesized that maternal inflammatory responses to the virus, rather than direct viral invasion of the fetal brain, may be the primary mechanism driving these alterations. Further research is needed to fully understand the long-term consequences of prenatal COVID-19 exposure on neurodevelopmental outcomes.
The interplay of these genetic and environmental factors is complex and dynamic. Understanding how these factors interact to shape brain development is crucial for developing effective strategies to prevent neurodevelopmental disorders and promote optimal brain health.
4. Gray Matter Volume and Cortical Folding: Key Indices of Brain Maturation
Gray matter, consisting primarily of neuronal cell bodies, dendrites, and synapses, plays a crucial role in information processing. Gray matter volume (GMV) is a quantitative measure of the amount of gray matter in a specific brain region or the entire brain. Cortical folding, or gyrification, refers to the folding patterns of the cerebral cortex, which significantly increases its surface area and neural density. Both GMV and cortical folding are key indices of brain maturation and function, providing valuable insights into neurodevelopmental processes.
4.1 Gray Matter Volume: GMV increases rapidly during infancy and early childhood, reflecting the proliferation of neurons, the growth of dendrites, and the formation of synapses. This increase is followed by a period of synaptic pruning, which leads to a gradual decrease in GMV during adolescence and adulthood. Variations in GMV have been associated with a wide range of cognitive abilities, including intelligence, language skills, and memory. Reduced GMV in specific brain regions has been observed in individuals with neurodevelopmental disorders such as ASD, ADHD, and schizophrenia. Age-related changes in gray matter volume are also well documented [3].
4.2 Cortical Folding: The degree of cortical folding is another important indicator of brain maturation. The gyrification index (GI) is a commonly used measure of cortical folding, quantifying the ratio of the actual cortical surface area to the smoothed outer surface area. Higher GI values indicate greater cortical folding. Cortical folding increases dramatically during the third trimester of gestation and early postnatal period. Variations in cortical folding patterns have been associated with cognitive abilities and susceptibility to neurological disorders. Altered cortical folding has been observed in individuals with ASD, schizophrenia, and other neurodevelopmental disorders. For example, studies have shown decreased folding in the temporal lobes of children with autism [4].
4.3 Reference Ranges and Expected Changes: Establishing reference ranges for GMV and cortical folding is essential for identifying atypical brain development. However, these ranges can vary depending on factors such as age, sex, ethnicity, and MRI acquisition parameters. Longitudinal studies are crucial for tracking expected changes in GMV and cortical folding over time and for identifying deviations from typical developmental trajectories. These studies provide critical information for early detection and intervention.
4.4 Gray Matter and Cortical Folding: Functional Relevance: The size and folding of the cortex are known to be related to cognitive function. Greater cortical surface area allows for greater neuronal density, more complex neural networks, and thus, increased computational power. The folding pattern itself can influence the connections between different brain regions, facilitating information transfer. Disruptions to either GMV or cortical folding can, therefore, affect cognitive processes such as attention, language, memory, and social cognition [5]. It is important to note, however, that the relationship between brain structure and function is complex and multifactorial. Structural differences may represent compensatory mechanisms, or may not directly translate into functional impairments. Furthermore, environmental factors can play a significant role in modulating the functional consequences of structural brain differences.
5. Methodologies for Analyzing Infant Brain Structure
Studying infant brain structure presents unique challenges due to the vulnerability of the developing brain and the limitations of invasive techniques. Non-invasive neuroimaging techniques, such as magnetic resonance imaging (MRI) and electroencephalography (EEG), are the primary methods used to analyze infant brain structure and function.
5.1 Magnetic Resonance Imaging (MRI): MRI is a powerful neuroimaging technique that provides high-resolution images of brain structure. MRI uses strong magnetic fields and radio waves to generate images based on the different magnetic properties of tissues. Several MRI techniques are used to study infant brain structure, including:
- Structural MRI: Structural MRI provides detailed images of brain anatomy, allowing for the measurement of GMV, cortical thickness, and cortical folding. Different MRI sequences, such as T1-weighted and T2-weighted imaging, provide different contrasts, highlighting different tissue properties.
- Diffusion Tensor Imaging (DTI): DTI measures the diffusion of water molecules in the brain, providing information about the structure and integrity of white matter tracts. DTI can be used to assess myelination, axonal density, and fiber organization.
- Functional MRI (fMRI): fMRI measures brain activity by detecting changes in blood flow. fMRI can be used to study the neural correlates of cognitive and behavioral functions in infants.
- Magnetic Resonance Spectroscopy (MRS): MRS measures the concentrations of different neurochemicals in the brain, providing information about metabolic processes and neuronal function.
While MRI is a powerful technique, it also has limitations. MRI scans are expensive, time-consuming, and require infants to remain still for extended periods. Motion artifact can be a significant problem in infant MRI studies. To address this issue, researchers often use sedation or natural sleep to minimize motion. Furthermore, the interpretation of MRI data requires specialized expertise and sophisticated image processing techniques.
5.2 Electroencephalography (EEG): EEG is a non-invasive neurophysiological technique that measures electrical activity in the brain using electrodes placed on the scalp. EEG provides information about brain function and can be used to assess sleep patterns, detect seizures, and study cognitive processes. EEG is relatively inexpensive and easy to use, making it a valuable tool for studying infant brain function. However, EEG has limited spatial resolution, making it difficult to pinpoint the precise location of brain activity.
5.3 Advanced Analysis Techniques: Beyond simply visually inspecting MRI images, researchers employ sophisticated computational methods to quantify brain structure. These include:
- Voxel-Based Morphometry (VBM): VBM is a statistical technique used to analyze differences in GMV between groups of individuals. VBM involves segmenting MRI images into gray matter, white matter, and cerebrospinal fluid, and then comparing the volume of each tissue type across different groups.
- Surface-Based Morphometry (SBM): SBM focuses on the analysis of the cortical surface, allowing for the measurement of cortical thickness, cortical folding, and other surface-based features.
- Connectivity Analysis: Connectivity analysis examines the structural and functional connections between different brain regions. This can be done using DTI (structural connectivity) or fMRI (functional connectivity).
The selection of appropriate neuroimaging techniques and analysis methods depends on the specific research question and the characteristics of the study population. Combining different neuroimaging techniques can provide a more comprehensive understanding of infant brain structure and function.
6. Long-Term Cognitive and Behavioral Outcomes
Understanding how structural brain differences observed in infancy translate into functional differences and long-term cognitive and behavioral outcomes is a major challenge in developmental neuroscience. While specific structural differences, such as reduced GMV or altered cortical folding, have been associated with various neurodevelopmental disorders, establishing direct causal links is often difficult.
6.1 Cognitive Outcomes: Variations in brain structure can influence a wide range of cognitive abilities, including intelligence, language skills, memory, attention, and executive functions. For example, reduced GMV in the prefrontal cortex has been associated with impaired executive functions, such as planning, working memory, and impulse control. Altered cortical folding in the temporal lobes has been linked to language deficits. However, the relationship between brain structure and cognitive function is complex and multifactorial. Environmental factors, such as early experiences and educational opportunities, can also play a significant role in shaping cognitive development.
6.2 Behavioral Outcomes: Structural brain differences can also contribute to behavioral problems, such as anxiety, depression, aggression, and social difficulties. For example, altered amygdala volume and connectivity have been associated with increased anxiety and social withdrawal. Reduced GMV in the orbitofrontal cortex has been linked to impulsive behavior and aggression. However, it is important to note that behavior is influenced by a complex interplay of genetic, environmental, and neurobiological factors. Structural brain differences may increase susceptibility to certain behavioral problems, but they do not necessarily determine outcomes.
6.3 Neurodevelopmental Disorders: Specific structural brain differences have been consistently observed in individuals with neurodevelopmental disorders, such as ASD, ADHD, and schizophrenia. For example, individuals with ASD often exhibit reduced GMV in the frontal and temporal lobes, altered cortical folding patterns, and atypical white matter connectivity. Individuals with ADHD may have reduced GMV in the prefrontal cortex and basal ganglia. Individuals with schizophrenia may show reduced GMV in the frontal and temporal lobes, enlarged ventricles, and altered white matter connectivity. However, it is important to note that these are group-level findings, and there is considerable variability in brain structure among individuals with the same diagnosis.
6.4 Challenges in Establishing Causal Links: Establishing direct causal links between structural brain differences and long-term cognitive and behavioral outcomes is a major challenge. Several factors contribute to this difficulty:
- Complexity of Brain Development: Brain development is a complex and dynamic process, influenced by a myriad of genetic and environmental factors. It is difficult to isolate the specific contribution of any single factor to long-term outcomes.
- Compensatory Mechanisms: The brain is highly plastic and capable of adapting to challenges. Structural brain differences may be compensated for by other brain regions or by environmental interventions.
- Heterogeneity of Neurodevelopmental Disorders: Neurodevelopmental disorders are highly heterogeneous, with varying degrees of severity and different symptom profiles. This makes it difficult to identify consistent brain-behavior relationships.
- Ethical Considerations: Longitudinal studies that track individuals from infancy to adulthood are essential for establishing causal links, but they can be ethically challenging to conduct.
Despite these challenges, ongoing research is making progress in understanding the relationship between brain structure and function. Advanced neuroimaging techniques, sophisticated statistical methods, and large-scale longitudinal studies are providing valuable insights into the complexities of early brain development and its impact on long-term outcomes.
7. Conclusion
Early brain development is a period of remarkable plasticity and vulnerability. Understanding the factors that can influence brain structure and function during this critical period is essential for promoting optimal neurodevelopmental outcomes and for preventing neurodevelopmental disorders. While genetic factors play a fundamental role in shaping brain architecture, environmental factors, such as exposure to teratogens, maternal stress, and socioeconomic disparities, can significantly impact brain development. Gray matter volume and cortical folding are key indices of brain maturation and function, providing valuable insights into neurodevelopmental processes. Advanced neuroimaging techniques, such as MRI and EEG, are essential tools for studying infant brain structure. Establishing direct causal links between structural brain differences and long-term cognitive and behavioral outcomes remains a major challenge. However, ongoing research is making progress in understanding the complex interplay of genetic, environmental, and neurobiological factors that shape brain development and behavior. By continuing to invest in research and early intervention programs, we can work towards ensuring that all children have the opportunity to reach their full neurodevelopmental potential.
References
[1] Alzate-Correa, D., et al. (2023). Association of Prenatal Exposure to Severe Acute Respiratory Syndrome Coronavirus 2 (SARS-CoV-2) With Neonatal Brain Structure. JAMA Network Open, 6(1), e2245907.
[2] Coker, D. A., et al. (2023). Impact of Gestational COVID-19 on Infant Brain Development. medRxiv, 2023-03.
[3] Raz, N., Gunning-Dixon, F. M., Head, D., Rodrigues, K. J., Williamson, A., & Acker, J. D. (2005). Age-related regional differences in brain structure: trajectories of change in the adult lifespan. Cerebral cortex, 15(11), 1676-1689.
[4] Hardan, A. Y., Minshew, N. J., & Keshavan, M. S. (2006). Decreased regional brain gray matter volume in autism. Archives of general psychiatry, 63(1), 54-61.
[5] Lyu, I., Khundrakpam, B. S., Yoon, U., Kim, J., Lee, J. M., & Kim, K. S. (2020). Association of cortical folding patterns with cognitive performance in healthy adults. Brain structure and function, 225, 1909-1925.
So, if maternal stress can tweak baby brains, does that mean future parents should binge-watch only calming nature documentaries? Asking for a friend who may or may not be planning a very zen pregnancy. What’s the most hilariously ineffective method anyone’s tried to de-stress for baby’s sake?
That’s a fantastic question! The idea of a ‘zen pregnancy’ is definitely appealing. While calming nature documentaries might help, perhaps laughter is also key. I’m curious, has anyone tried laughter yoga or something similar for stress during pregnancy? Would love to hear about any experiences!
Editor: MedTechNews.Uk
Thank you to our Sponsor Esdebe
So, if our brains are meticulously folding like origami masterpieces in utero, does that mean listening to podcasts about advanced paper-folding techniques might give my future offspring a cognitive edge? Or would it just result in a baby who’s oddly obsessed with cranes?
That’s such a fun thought! While origami podcasts might not directly impact brain folding, exposing babies to a variety of stimulating experiences, like music or stories, is definitely beneficial for their cognitive development. Maybe a little Mozart, or a children’s book would be a better alternative. Who knows, perhaps they’ll develop a love of origami later in life!
Editor: MedTechNews.Uk
Thank you to our Sponsor Esdebe