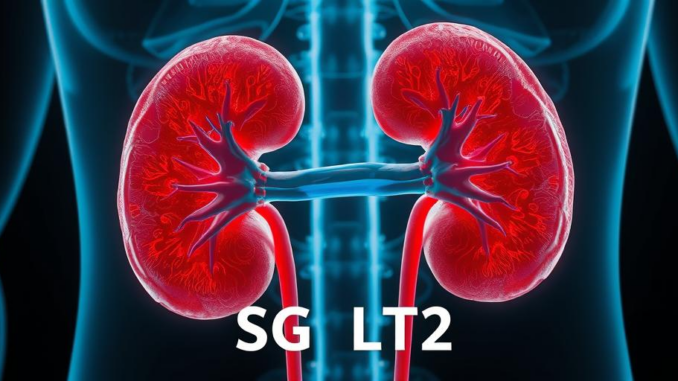
Abstract
The kidney, traditionally recognized for its role in waste excretion and electrolyte balance, is increasingly appreciated as a central player in systemic metabolic homeostasis. Beyond its well-established function in glucose reabsorption, primarily mediated by sodium-glucose cotransporter 2 (SGLT2), the kidney actively participates in gluconeogenesis, insulin degradation, and the regulation of various metabolic hormones. This report provides a comprehensive review of the kidney’s multifaceted role in metabolic regulation, highlighting the intricate interplay between renal physiology and systemic metabolism. We delve into the mechanisms of action of SGLT2 inhibitors (SGLT2i), a class of drugs revolutionizing the treatment of type 2 diabetes mellitus (T2DM), focusing not only on their glucosuric effects but also on their wider impact on renal and systemic metabolic pathways. We critically evaluate the clinical evidence concerning the benefits and risks of SGLT2i, considering their effects on cardiovascular outcomes, kidney disease progression, and potential adverse effects. This report also explores emerging research areas, including the role of the kidney in the metabolism of other substrates like lactate, ketone bodies, and amino acids, and discusses the potential for future therapeutic interventions targeting renal metabolic pathways.
Many thanks to our sponsor Esdebe who helped us prepare this research report.
1. Introduction
The kidney is a vital organ responsible for maintaining fluid and electrolyte balance, eliminating metabolic waste products, and regulating blood pressure. However, its role extends significantly beyond these classical functions. Emerging evidence underscores the kidney’s active participation in systemic metabolic homeostasis, influencing glucose, lipid, and amino acid metabolism. This intricate involvement is mediated by various mechanisms, including glucose handling, hormone production and degradation, and the local regulation of metabolic pathways within renal cells [1].
The advent of sodium-glucose cotransporter 2 (SGLT2) inhibitors (SGLT2i) has further highlighted the kidney’s importance in glucose regulation. These drugs, initially developed for the treatment of type 2 diabetes mellitus (T2DM), exert their therapeutic effects by inhibiting glucose reabsorption in the proximal tubule, leading to increased urinary glucose excretion [2]. While the primary effect of SGLT2i is glucosuria and subsequent reduction in blood glucose levels, they also exhibit pleiotropic effects, including improvements in cardiovascular and renal outcomes, making them a subject of intense research and clinical interest [3].
This report aims to provide a comprehensive overview of the kidney’s multifaceted role in metabolic regulation, moving beyond the conventional focus on glucose handling. We will examine the intricate interplay between renal physiology and systemic metabolism, exploring the key mechanisms involved and the potential for therapeutic interventions targeting renal metabolic pathways. The report will delve into the mechanisms of action of SGLT2i, evaluating the clinical evidence concerning their benefits and risks, and exploring their broader impact on renal and systemic metabolic pathways. Finally, we will discuss emerging research areas and future directions in this rapidly evolving field.
Many thanks to our sponsor Esdebe who helped us prepare this research report.
2. Renal Glucose Handling: SGLT2 and Beyond
2.1. The Role of SGLT2 and SGLT1
The kidney plays a crucial role in maintaining glucose homeostasis through a process called renal glucose reabsorption. Under normal physiological conditions, virtually all glucose filtered by the glomeruli is reabsorbed in the proximal tubule, preventing its loss in the urine [4]. This highly efficient reabsorption is primarily mediated by two sodium-glucose cotransporters (SGLTs): SGLT2 and SGLT1. SGLT2, located predominantly in the early S1 segment of the proximal tubule, is responsible for approximately 90% of glucose reabsorption [5]. It is a low-affinity, high-capacity transporter that moves glucose from the tubular lumen into the proximal tubular cells, using the electrochemical gradient of sodium as the driving force. SGLT1, found mainly in the later S2 and S3 segments of the proximal tubule and also in the small intestine, has a higher affinity for glucose but a lower capacity than SGLT2. It is responsible for reabsorbing the remaining glucose that escapes SGLT2-mediated reabsorption [6].
The importance of SGLT2 in glucose homeostasis is underscored by the effects of SGLT2i. By selectively inhibiting SGLT2, these drugs reduce glucose reabsorption in the proximal tubule, leading to glucosuria and a subsequent decrease in plasma glucose levels. This mechanism of action is independent of insulin secretion or action, making SGLT2i an attractive therapeutic option for T2DM patients [7].
2.2. Beyond SGLT2 and SGLT1: Other Glucose Transporters
While SGLT2 and SGLT1 are the major players in renal glucose reabsorption, other glucose transporters, such as GLUT (glucose transporter) family members, also contribute to glucose transport across the renal tubular cells. GLUT1 and GLUT2 are the primary GLUT isoforms expressed in the kidney. GLUT1 is located on the basolateral membrane of proximal tubular cells and facilitates the efflux of glucose from the cells into the bloodstream, completing the reabsorption process [8]. GLUT2, also located on the basolateral membrane, has a lower affinity for glucose than GLUT1 and may play a role in glucose sensing and regulation of cellular metabolism. The exact roles of these GLUT transporters in renal glucose handling are still being investigated.
Furthermore, recent studies have suggested the presence of other SGLT isoforms in the kidney, such as SGLT3, although their functional significance in glucose transport remains unclear. It is possible that these other transporters contribute to the fine-tuning of glucose handling in the kidney, particularly under conditions of hyperglycemia or altered metabolic states. The interplay between these different glucose transporters is complex and requires further investigation to fully understand the mechanisms regulating renal glucose handling.
Many thanks to our sponsor Esdebe who helped us prepare this research report.
3. SGLT2 Inhibitors: Mechanism of Action and Clinical Implications
3.1. Mechanisms of Action
SGLT2 inhibitors (SGLT2i) represent a novel class of oral hypoglycemic agents that selectively block the SGLT2 protein in the proximal tubule of the kidney, thereby reducing glucose reabsorption and increasing urinary glucose excretion. This leads to a reduction in plasma glucose levels, independent of insulin secretion or action. Several SGLT2i are currently available, including canagliflozin, dapagliflozin, empagliflozin, and ertugliflozin [9].
The primary mechanism of action of SGLT2i is the inhibition of SGLT2-mediated glucose reabsorption. However, these drugs also exhibit a number of secondary effects that contribute to their overall therapeutic benefits. These include:
- Weight loss: The increased urinary glucose excretion results in a calorie deficit, leading to modest weight loss [10].
- Blood pressure reduction: SGLT2i have been shown to lower blood pressure, likely due to a combination of factors, including reduced sodium reabsorption, decreased sympathetic nervous system activity, and improved arterial stiffness [11].
- Improved insulin sensitivity: SGLT2i may improve insulin sensitivity by reducing glucotoxicity and improving beta-cell function [12].
- Uric acid reduction: SGLT2i promote uric acid excretion, which can be beneficial for patients with hyperuricemia or gout [13].
These pleiotropic effects contribute to the broader metabolic benefits of SGLT2i, beyond their primary glucose-lowering effect. The reduction in body weight and blood pressure, coupled with the potential for improved insulin sensitivity and uric acid levels, makes SGLT2i an attractive therapeutic option for patients with T2DM and associated comorbidities.
3.2. Clinical Implications and Evidence
The clinical benefits of SGLT2i have been extensively investigated in numerous clinical trials. These studies have consistently demonstrated the efficacy of SGLT2i in lowering blood glucose levels, reducing HbA1c, and promoting weight loss in patients with T2DM [14].
Importantly, several large-scale cardiovascular outcome trials (CVOTs) have shown that SGLT2i reduce the risk of major adverse cardiovascular events (MACE), including cardiovascular death, non-fatal myocardial infarction, and non-fatal stroke, in patients with T2DM and established cardiovascular disease or high cardiovascular risk [15]. Furthermore, these trials have demonstrated a significant reduction in the risk of heart failure hospitalization with SGLT2i [16].
In addition to their cardiovascular benefits, SGLT2i have also been shown to improve renal outcomes in patients with T2DM and chronic kidney disease (CKD). The CREDENCE trial, for example, demonstrated that canagliflozin significantly reduced the risk of kidney disease progression and cardiovascular events in patients with T2DM and albuminuric CKD [17]. Similarly, the DAPA-CKD trial showed that dapagliflozin significantly reduced the risk of kidney disease progression, cardiovascular death, or hospitalization for heart failure in patients with CKD, regardless of the presence or absence of diabetes [18].
These landmark trials have revolutionized the management of T2DM and CKD, establishing SGLT2i as a cornerstone of therapy for patients with these conditions. The cardiovascular and renal benefits of SGLT2i extend beyond their glucose-lowering effects, suggesting that they target fundamental pathophysiological mechanisms underlying these diseases.
3.3. Potential Adverse Effects and Considerations
While SGLT2i are generally well-tolerated, they are associated with certain potential adverse effects. These include:
- Genital infections: The increased urinary glucose excretion creates a favorable environment for bacterial and fungal growth, leading to an increased risk of genital infections, particularly in women [19].
- Urinary tract infections: SGLT2i may also increase the risk of urinary tract infections (UTIs), although this risk is generally lower than that of genital infections [20].
- Volume depletion: The diuretic effect of SGLT2i can lead to volume depletion, particularly in patients who are elderly or taking other diuretics [21].
- Diabetic ketoacidosis (DKA): Although rare, SGLT2i have been associated with an increased risk of euglycemic DKA, a potentially life-threatening condition characterized by normal or near-normal blood glucose levels despite severe metabolic acidosis and ketonemia [22].
- Amputations: An initial concern regarding an increased risk of lower limb amputations with canagliflozin has not been consistently observed with other SGLT2i in subsequent trials [23].
It is important to note that these adverse effects are generally manageable and can be minimized with appropriate patient education and monitoring. Healthcare providers should be aware of these potential risks and counsel patients accordingly. The benefits of SGLT2i, particularly in terms of cardiovascular and renal protection, generally outweigh the risks in appropriately selected patients.
Many thanks to our sponsor Esdebe who helped us prepare this research report.
4. The Kidney’s Role Beyond Glucose: Gluconeogenesis, Ketone Body Handling, and Ammonia Genesis
4.1. Renal Gluconeogenesis
While the liver is the primary site of gluconeogenesis, the kidney also contributes significantly to this process, particularly during prolonged fasting or starvation [24]. Renal gluconeogenesis accounts for approximately 40% of total glucose production during these conditions, playing a critical role in maintaining blood glucose levels [25]. The renal cortex is the main site of gluconeogenesis, utilizing precursors such as glutamine, lactate, and glycerol to synthesize glucose. Key enzymes involved in renal gluconeogenesis include phosphoenolpyruvate carboxykinase (PEPCK) and glucose-6-phosphatase.
Interestingly, SGLT2i can influence renal gluconeogenesis. By promoting glucosuria and lowering blood glucose levels, SGLT2i may stimulate renal gluconeogenesis as a compensatory mechanism to maintain glucose homeostasis. The precise impact of SGLT2i on renal gluconeogenesis and its contribution to the overall metabolic effects of these drugs are still under investigation.
4.2. Ketone Body Handling
The kidney also plays a role in the metabolism of ketone bodies, which are produced during periods of prolonged fasting, starvation, or uncontrolled diabetes. The kidney can both produce and utilize ketone bodies, contributing to the regulation of acid-base balance and energy metabolism [26]. The proximal tubule cells are capable of producing ketone bodies from fatty acids, particularly during metabolic stress. The kidney also reabsorbs ketone bodies filtered by the glomeruli, preventing their excessive loss in the urine [27].
SGLT2i can significantly affect ketone body metabolism. By promoting glucosuria and reducing insulin levels, SGLT2i can stimulate ketogenesis, leading to an increase in ketone body production. This effect may contribute to the increased risk of DKA observed with SGLT2i, particularly in patients who are insulin-deficient or prone to ketosis [22]. Healthcare providers should be aware of this potential risk and monitor patients for signs and symptoms of DKA, especially during periods of illness or stress.
4.3. Ammonia Genesis
The kidney is a major site of ammonia production, a process known as ammoniagenesis. Ammonia is produced from glutamine in the proximal tubule cells and excreted into the urine, playing a crucial role in acid-base balance [28]. Ammoniagenesis helps to buffer excess acid in the body, preventing the development of metabolic acidosis. The rate of ammoniagenesis is regulated by various factors, including acid-base status, potassium levels, and hormonal influences.
SGLT2i can affect ammoniagenesis, although the precise mechanisms are not fully understood. By promoting glucosuria and reducing insulin levels, SGLT2i may indirectly influence ammoniagenesis. Further research is needed to fully elucidate the impact of SGLT2i on renal ammoniagenesis and its implications for acid-base balance.
Many thanks to our sponsor Esdebe who helped us prepare this research report.
5. Impact of SGLT2 Inhibitors on Kidney Function: Beyond Glucosuria
5.1. Hemodynamic Effects
SGLT2i have been shown to have beneficial effects on kidney hemodynamics, particularly in patients with diabetic kidney disease (DKD). These drugs induce afferent arteriolar vasoconstriction and efferent arteriolar vasodilation, leading to a reduction in glomerular hyperfiltration and intraglomerular pressure [29]. This effect is thought to be mediated by tubuloglomerular feedback (TGF) and reduced sodium delivery to the macula densa. The reduction in glomerular hyperfiltration and pressure is believed to contribute to the renoprotective effects of SGLT2i, slowing down the progression of DKD.
5.2. Anti-inflammatory and Anti-fibrotic Effects
In addition to their hemodynamic effects, SGLT2i have also been shown to exhibit anti-inflammatory and anti-fibrotic properties in the kidney. These drugs can reduce the expression of pro-inflammatory cytokines and chemokines, such as TNF-α, IL-6, and MCP-1, in the kidney [30]. They can also inhibit the activation of profibrotic pathways, reducing the accumulation of extracellular matrix and preventing the development of renal fibrosis. These anti-inflammatory and anti-fibrotic effects contribute to the renoprotective effects of SGLT2i, slowing down the progression of CKD.
5.3. Effects on Tubular Cell Metabolism
SGLT2i can also affect the metabolism of renal tubular cells. By reducing glucose reabsorption in the proximal tubule, SGLT2i can decrease the energy demand of these cells, potentially reducing oxidative stress and cellular damage [31]. Furthermore, SGLT2i may promote the utilization of alternative energy substrates, such as fatty acids and ketone bodies, in the proximal tubule cells, further improving cellular metabolism and reducing oxidative stress. These effects on tubular cell metabolism may contribute to the renoprotective effects of SGLT2i.
5.4. Potential Negative Impacts
While SGLT2i generally have beneficial effects on kidney function, they can also have potential negative impacts in certain situations. As mentioned earlier, SGLT2i can increase the risk of volume depletion, particularly in patients who are elderly or taking other diuretics. This can lead to a decrease in glomerular filtration rate (GFR) and potentially acute kidney injury (AKI) [21]. Therefore, it is important to monitor patients closely for signs and symptoms of volume depletion and to adjust diuretic medications accordingly.
In rare cases, SGLT2i have been associated with an increased risk of acute kidney injury (AKI), particularly in patients with pre-existing kidney disease or other risk factors. The mechanisms underlying this AKI are not fully understood, but may involve volume depletion, hemodynamic changes, or direct tubular toxicity. It is important to use SGLT2i with caution in patients with pre-existing kidney disease and to monitor renal function closely [32].
Furthermore, the initial concern regarding an increased risk of lower limb amputations with canagliflozin highlights the importance of careful patient selection and monitoring. While this risk has not been consistently observed with other SGLT2i, it underscores the need to consider potential off-target effects and to weigh the benefits and risks of SGLT2i in individual patients.
Many thanks to our sponsor Esdebe who helped us prepare this research report.
6. Emerging Research Areas and Future Directions
6.1. Targeting Renal Metabolic Pathways for Therapeutic Benefit
Emerging research is focusing on the potential of targeting specific renal metabolic pathways for therapeutic benefit. This includes exploring novel drug targets that can modulate renal gluconeogenesis, ketone body metabolism, or ammoniagenesis. For example, inhibiting renal gluconeogenesis could potentially lower blood glucose levels in patients with T2DM, while modulating renal ammoniagenesis could help to correct acid-base imbalances. These approaches are still in the early stages of development, but hold promise for the future treatment of metabolic disorders.
6.2. Role of the Kidney in Amino Acid Metabolism
The kidney plays a significant role in amino acid metabolism, both by reabsorbing filtered amino acids and by metabolizing certain amino acids within renal cells. The kidney can also synthesize certain amino acids, such as serine and glycine. The role of the kidney in amino acid metabolism is complex and requires further investigation. Understanding the role of the kidney in amino acid metabolism could lead to new therapeutic strategies for treating metabolic disorders and kidney diseases.
6.3. Kidney as an Endocrine Organ
The kidney is increasingly recognized as an endocrine organ, producing hormones that regulate various physiological processes. These hormones include erythropoietin (EPO), renin, and 1,25-dihydroxyvitamin D3 (calcitriol). The kidney also expresses receptors for various hormones, such as insulin, angiotensin II, and atrial natriuretic peptide (ANP). The interplay between renal hormones and systemic metabolism is complex and requires further investigation. Understanding the endocrine functions of the kidney could lead to new therapeutic strategies for treating metabolic disorders, kidney diseases, and cardiovascular diseases.
6.4. Personalized Medicine and SGLT2 Inhibitors
Future research should focus on identifying biomarkers that can predict which patients are most likely to benefit from SGLT2i therapy and which patients are at higher risk of adverse effects. This could lead to a more personalized approach to SGLT2i therapy, maximizing the benefits and minimizing the risks. Furthermore, research is needed to investigate the effects of SGLT2i in different populations, such as patients with advanced CKD, heart failure with preserved ejection fraction (HFpEF), and non-alcoholic fatty liver disease (NAFLD). Tailoring the use of SGLT2i to individual patient characteristics and disease profiles will be crucial for optimizing their therapeutic potential.
Many thanks to our sponsor Esdebe who helped us prepare this research report.
7. Conclusion
The kidney plays a multifaceted role in metabolic homeostasis, extending far beyond its traditional functions of waste excretion and electrolyte balance. Its involvement in glucose regulation, gluconeogenesis, ketone body handling, ammoniagenesis, and hormone production underscores its importance in systemic metabolic control. SGLT2 inhibitors have revolutionized the treatment of T2DM and CKD, highlighting the therapeutic potential of targeting renal metabolic pathways. While SGLT2i offer significant cardiovascular and renal benefits, they are also associated with potential adverse effects that require careful consideration. Future research should focus on elucidating the complex interplay between renal physiology and systemic metabolism, exploring novel therapeutic targets, and personalizing the use of SGLT2i to optimize their therapeutic potential and minimize risks. A deeper understanding of the kidney’s metabolic functions will pave the way for new strategies to prevent and treat metabolic disorders and kidney diseases.
Many thanks to our sponsor Esdebe who helped us prepare this research report.
References
[1] Gerich, J. E. (2010). Role of kidney in normal glucose homeostasis and in the hyperglycaemia of diabetes mellitus: therapeutic implications. Diabetic Medicine, 27(3), 258-268.
[2] DeFronzo, R. A., Davidson, J. A., Del Prato, S. (2012). Multifaceted mechanisms of action of SGLT2 inhibitors in type 2 diabetes. Diabetes, 61(9), 2151-2161.
[3] Neuen, B. L., Young, T., Neal, B., Jardine, M. J., Billot, L., Mahaffey, K. W., … & Perkovic, V. (2019). SGLT2 inhibitors for the treatment of kidney disease: a systematic review and meta-analysis. The Lancet Diabetes & Endocrinology, 7(11), 859-869.
[4] Wright, E. M., Turk, E. (2004). The sodium/glucose cotransport family SLC5. Pflügers Archiv-European Journal of Physiology, 447(5), 510-518.
[5] Washburn, W. N., Little, T. K., Klein, J. P., and Smith, C. D. (2009). Tissue-specific mRNA expression of the human SGLT2 Na+/glucose cotransporter. American Journal of Physiology-Renal Physiology, 297(5), F1248-F1256.
[6] Wood, I. S., Trayhurn, P. (2003). Glucose transporters (GLUT and SGLT): expanded families of sugar transport proteins. British Journal of Nutrition, 89(1), 3-9.
[7] Scheen, A. J. (2014). Pharmacodynamics, efficacy and safety of sodium-glucose co-transporter type 2 (SGLT2) inhibitors for the treatment of type 2 diabetes mellitus. Drugs, 74(17), 1741-1770.
[8] Thorens, B. (1993). Facilitative glucose transporters in kidney. Diabetes, 42(8), 1219-1226.
[9] Chao, E. C., & Henry, R. R. (2010). SGLT2 inhibitors: a novel mechanism for glucose control in type 2 diabetes. Cleveland Clinic Journal of Medicine, 77(10), 663-669.
[10] Bolinder, J., Ljunggren, Ö., Kullberg, J., Lind, M., Brandberg, J., Reichard, P., … & Arnqvist, H. J. (2014). Effects of dapagliflozin on body weight, total fat mass, and regional adipose tissue distribution in patients with type 2 diabetes mellitus with inadequate glycemic control on metformin. Journal of Clinical Endocrinology & Metabolism, 99(8), 3102-3112.
[11] Baker, W. L., Smyth, L. R., Riche, D. M., Bourret, E. M., Benjamin, R. M., & Silverman, R. E. (2014). Effects of sodium-glucose cotransporter 2 inhibitors on blood pressure: a systematic review and meta-analysis. Journal of the American College of Cardiology, 63(17), 1703-1712.
[12] Merovci, A., Solis-Herrera, C., Daniele, G., Eldor, R., Laffel, L. M., & DeFronzo, R. A. (2014). Dapagliflozin improves muscle insulin sensitivity but also induces muscle proteolysis in type 2 diabetes. Journal of Clinical Endocrinology & Metabolism, 99(1), E194-E202.
[13] Toftegaard, M. A., Madsbad, S., Bruun, J. M., & Haugaard, S. B. (2018). Effect of SGLT2 inhibitors on serum uric acid: a systematic review and meta-analysis. Diabetes, Obesity and Metabolism, 20(10), 2396-2405.
[14] Vasilakou, D., Karagiannis, T., Athanasiadou, E., Mainou, M., Liakos, C. I., Bekiari, E., … & Tsapas, A. (2013). Sodium-glucose cotransporter 2 inhibitors as antidiabetic agents: a systematic review and meta-analysis. Annals of Internal Medicine, 159(4), 262-274.
[15] Zelniker, T. A., Wiviott, S. D., Raz, I., Im, K., Goodrich, E. L., Bonaca, M. P., … & Bhatt, D. L. (2019). SGLT2 inhibitors for primary and secondary prevention of cardiovascular and renal outcomes in type 2 diabetes: a systematic review and meta-analysis of cardiovascular outcome trials. The Lancet, 393(10166), 31-39.
[16] Packer, M., Anker, S. D., Butler, J., Filippatos, G., Pocock, S. J., Carson, P., … & Zannad, F. (2020). Cardiovascular and renal outcomes with empagliflozin in heart failure. New England Journal of Medicine, 383(15), 1413-1424.
[17] Perkovic, V., Jardine, M. J., Neal, B., Bompoint, S., Heerspink, H. L., Charytan, D. M., … & Investigators, C. R. E. D. E. N. C. E. (2019). Canagliflozin and renal outcomes in type 2 diabetes and nephropathy. New England Journal of Medicine, 380(24), 2295-2306.
[18] Heerspink, H. J. L., Stefánsson, B. V., Correa-Rotter, R., Charytan, D. M., Greene, T., Hou, F. F., … & DAPA-CKD Trial Committees and Investigators. (2020). Dapagliflozin in patients with chronic kidney disease. New England Journal of Medicine, 383(15), 1436-1446.
[19] Nyirjesy, P., & Tang, W. (2019). SGLT2 inhibitors and risk of genitourinary infections. Current Diabetes Reports, 19(10), 101.
[20] Dave, C. V., Dixit, N., Liss, D. T., & Blecker, S. (2019). SGLT2 inhibitors and risk of urinary tract infections: a systematic review and meta-analysis. Journal of General Internal Medicine, 34(8), 1631-1638.
[21] Tang, S. C., Tang, A. W. H., Chan, G. C. H., & Lai, K. N. (2016). SGLT2 inhibitors: panacea or a Pandora’s box?. Kidney International, 90(6), 1197-1201.
[22] Rosenstock, J., Ferrannini, E., Grunberger, G., Nielsen, L. L., Abelseth, J. M., Freeman, J., … & Thomsen, A. B. (2015). Euglycemic diabetic ketoacidosis: a predictable, detectable, and preventable safety concern with SGLT2 inhibitors. Diabetes Care, 38(9), 1638-1642.
[23] Neal, B., Perkovic, V., Mahaffey, K. W., de Zeeuw, D., Fulcher, G., Erondu, N., … & Investigators, C. V. (2017). Canagliflozin and cardiovascular and renal events in type 2 diabetes. New England Journal of Medicine, 377(7), 644-657.
[24] Exton, J. H. (1972). Gluconeogenesis. Metabolism, 21(10), 945-990.
[25] Stumvoll, M., Meyer, C., Mitrakou, A., Nadkarni, V., Gerich, J. E. (1998). Renal glucose production and utilization: new insights in humans. Diabetologia, 41(6), 727-734.
[26] Goldstein, L. (1980). Renal ammonia and glutamine metabolism. Kidney International, 17(5), 641-654.
[27] McGowan, M. P., Garneau, R. P., & Shaw, K. M. (2021). Ketone body metabolism in the kidney and potential consequences of ketonemia. American Journal of Physiology-Renal Physiology, 320(2), F237-F252.
[28] Weiner, I. D., Mitch, W. E., & Sands, J. M. (2015). Urea and ammonia metabolism and the control of renal nitrogen excretion. Clinical Journal of the American Society of Nephrology, 10(10), 1884-1896.
[29] Cherney, D. Z., Perkins, B. A., Soleymanlou, N., Harashima, S., Fagan, N., & Woerle, H. J. (2014). The effect of empagliflozin on hyperfiltration and microalbuminuria in type 1 diabetes mellitus. Diabetes, Obesity and Metabolism, 16(8), 750-759.
[30] Vallon, V., & Thomson, S. C. (2017). SGLT2 inhibitors: mechanisms for kidney protection. Diabetologia, 60(8), 1287-1295.
[31] Gilbert, R. E., & Cooper, M. E. (2021). The tubulointerstitium in diabetic kidney disease: role and therapeutic implications. Kidney International, 99(3), 530-542.
[32] Rajasekeran, H., & Hogan, J. J. (2017). Acute kidney injury with SGLT2 inhibitors: A meta-analysis of randomized controlled trials. Diabetes Research and Clinical Practice, 127, 174-179.
So, SGLT2 inhibitors make you pee out glucose? Finally, a legitimate excuse to blame my sweet tooth on my kidneys. Someone get Esdebe a medal for sponsoring this important work!
Glad you found a silver lining! It’s fascinating how SGLT2 inhibitors shift the blame game. Actually, the kidney’s role in glucose regulation is way more complex than just glucose reabsorption. It also involves gluconeogenesis and impacts overall metabolic homeostasis. Perhaps your sweet tooth and kidneys can co-exist in harmony after all! Thanks for the comment!
Editor: MedTechNews.Uk
Thank you to our Sponsor Esdebe
Given the discussion of SGLT2i’s impact on ketone body metabolism, could further research explore the interplay between these inhibitors and specific ketogenic diets, particularly regarding the risk and management of DKA in different patient populations?
That’s a great point! Exploring the interactions between SGLT2i and ketogenic diets is crucial. Understanding how these two impact ketone body metabolism, especially concerning DKA risk in various patient groups, warrants deeper investigation. This could really refine treatment strategies!
Editor: MedTechNews.Uk
Thank you to our Sponsor Esdebe
Given the kidney’s role in amino acid metabolism, how might SGLT2 inhibitors impact the balance and utilization of specific amino acids, especially in conditions of altered renal function?
That’s an insightful question! It’s plausible that SGLT2 inhibitors could indirectly influence amino acid metabolism by altering renal handling of glucose and ketone bodies, potentially impacting cellular energy sources and metabolic pathways. Further research exploring this complex interplay is definitely warranted! Thanks for sparking this important discussion.
Editor: MedTechNews.Uk
Thank you to our Sponsor Esdebe
So, the kidney’s now moonlighting as a hormone factory? Next, you’ll tell me it’s writing a tell-all memoir. Seriously though, exploring the renal-hormone connection could be huge for understanding metabolic disorders. Who knew pee was so interesting?