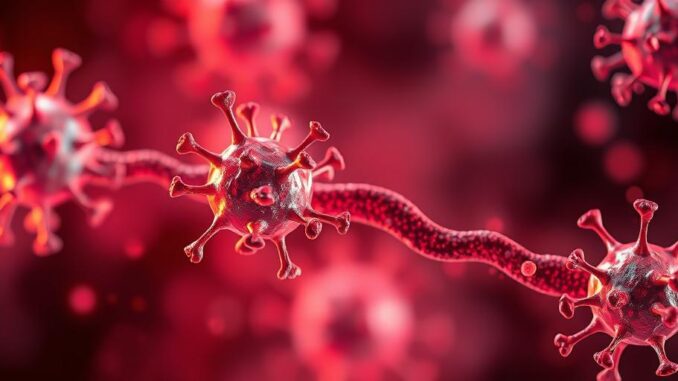
Abstract
Cytokine signaling pathways are critical for intercellular communication, regulating diverse cellular processes including proliferation, differentiation, survival, and immune responses. This review provides a comprehensive overview of cytokine signaling, focusing on the intricate mechanisms involved and the pleiotropic roles of these pathways in health and disease. We delve into the canonical and non-canonical signaling cascades initiated by cytokine receptor activation, emphasizing the complexities of receptor-ligand interactions, downstream effector molecules, and feedback regulatory mechanisms. Special attention is given to the Leukemia Inhibitory Factor Receptor (LIFR) pathway, exploring its diverse functions beyond adipogenesis, including its involvement in neuroprotection, stem cell maintenance, and immune modulation. Furthermore, we discuss the dysregulation of cytokine signaling in various pathologies, such as cancer, autoimmune disorders, and metabolic diseases. Finally, we assess the therapeutic potential of targeting cytokine signaling pathways, highlighting both the successes and challenges in developing effective and specific interventions. The report concludes by identifying key areas for future research, emphasizing the need for a more nuanced understanding of cytokine signaling networks to develop precision therapies with minimal off-target effects.
Many thanks to our sponsor Esdebe who helped us prepare this research report.
1. Introduction: The Cytokine Signaling Network
Cytokines are a diverse family of signaling molecules that mediate intercellular communication in both physiological and pathological contexts. These soluble proteins, peptides, or glycoproteins are secreted by a wide variety of cells, including immune cells, stromal cells, and even some cancer cells. Their effects are mediated by binding to specific receptors on target cells, triggering a cascade of intracellular signaling events that ultimately lead to changes in gene expression and cellular behavior. The cytokine signaling network is exceptionally complex, characterized by redundancy, pleiotropy, synergy, and antagonism. Redundancy refers to the ability of multiple cytokines to induce similar effects, while pleiotropy describes the capacity of a single cytokine to elicit diverse effects depending on the target cell type and context. Synergy occurs when the combined effect of two or more cytokines is greater than the sum of their individual effects, and antagonism refers to the ability of one cytokine to inhibit the activity of another.
Cytokine receptors can be broadly classified into several families based on their structure and signaling mechanisms, including:
- Type I cytokine receptors: These receptors typically possess conserved sequence motifs in their extracellular domains and include receptors for interleukins (ILs) such as IL-2, IL-6, and IL-12, as well as colony-stimulating factors (CSFs) and growth hormone (GH). They commonly signal through the JAK-STAT pathway.
- Type II cytokine receptors: This family includes receptors for interferons (IFNs), IL-10 family cytokines, and IL-28. Similar to type I receptors, they often utilize the JAK-STAT pathway.
- Tumor necrosis factor (TNF) receptors: This family includes receptors for TNF-α, lymphotoxin-α (LT-α), and Fas ligand (FasL). These receptors activate a variety of signaling pathways, including NF-κB, MAPK, and caspase-dependent apoptosis.
- Chemokine receptors: These G protein-coupled receptors (GPCRs) bind to chemokines and regulate cell migration, adhesion, and activation. They signal through heterotrimeric G proteins, leading to the activation of various downstream effectors, including MAP kinases and PI3K.
- Interleukin-1 (IL-1) receptors: This family includes receptors for IL-1, IL-18, and IL-33. These receptors activate the MyD88-dependent signaling pathway, leading to the activation of NF-κB and the production of inflammatory cytokines.
- Transforming growth factor-beta (TGF-β) receptors: These serine/threonine kinase receptors bind to TGF-β family ligands and activate the Smad signaling pathway, regulating cell growth, differentiation, and apoptosis.
The signaling pathways activated by cytokine receptors are diverse and interconnected, involving a complex network of intracellular kinases, phosphatases, transcription factors, and other regulatory molecules. Understanding these signaling pathways is crucial for comprehending the roles of cytokines in health and disease, and for developing targeted therapies that modulate cytokine activity.
Many thanks to our sponsor Esdebe who helped us prepare this research report.
2. Canonical Cytokine Signaling Pathways
The activation of cytokine receptors initiates a cascade of intracellular signaling events that ultimately lead to changes in gene expression and cellular behavior. Several canonical signaling pathways are commonly activated by cytokine receptors, including:
2.1 The JAK-STAT Pathway
The Janus kinase (JAK)-signal transducer and activator of transcription (STAT) pathway is a major signaling cascade activated by many type I and type II cytokine receptors. Upon ligand binding, the receptor undergoes a conformational change that brings the associated JAKs into close proximity, leading to their activation through transphosphorylation. Activated JAKs then phosphorylate tyrosine residues on the receptor cytoplasmic domain, creating docking sites for STAT proteins. STATs are recruited to the receptor, where they are phosphorylated by JAKs. Phosphorylated STATs dimerize, translocate to the nucleus, and bind to specific DNA sequences to regulate gene transcription. The JAK family comprises four members (JAK1, JAK2, JAK3, and TYK2), while the STAT family consists of seven members (STAT1, STAT2, STAT3, STAT4, STAT5A, STAT5B, and STAT6). Different cytokine receptors activate different combinations of JAKs and STATs, leading to diverse transcriptional responses. For instance, IFN-γ signaling predominantly activates JAK1 and JAK2, leading to the phosphorylation and activation of STAT1. In contrast, IL-6 signaling mainly activates JAK1 and JAK2, leading to the phosphorylation and activation of STAT3. The specificity of the JAK-STAT pathway is further regulated by receptor subunit composition and the presence of inhibitory proteins, such as suppressors of cytokine signaling (SOCS) and protein inhibitors of activated STATs (PIAS). SOCS proteins are induced by STAT activation and act as negative feedback regulators by binding to JAKs or receptor tyrosine kinases, thereby blocking STAT phosphorylation. PIAS proteins, on the other hand, directly bind to STATs and inhibit their DNA-binding activity. The JAK-STAT pathway is crucial for regulating a wide range of cellular processes, including cell proliferation, differentiation, survival, and immune responses. Dysregulation of this pathway has been implicated in various diseases, including cancer, autoimmune disorders, and inflammatory diseases.
2.2 The MAPK Pathway
The mitogen-activated protein kinase (MAPK) pathway is another major signaling cascade activated by cytokine receptors, as well as by growth factors, hormones, and stress signals. The MAPK pathway is a highly conserved signaling module consisting of a three-tiered kinase cascade: a MAPK kinase kinase (MAPKKK), a MAPK kinase (MAPKK), and a MAPK. Activation of the MAPK pathway is initiated by the recruitment of adaptor proteins, such as Grb2 and SOS, to the receptor. These adaptor proteins activate Ras, a small GTPase that triggers the activation of MAPKKK. The MAPKKK then phosphorylates and activates MAPKK, which in turn phosphorylates and activates MAPK. Once activated, MAPK translocates to the nucleus and phosphorylates transcription factors, leading to changes in gene expression. Several MAPK pathways have been identified, including the extracellular signal-regulated kinase (ERK) pathway, the p38 MAPK pathway, and the c-Jun N-terminal kinase (JNK) pathway. The ERK pathway is typically activated by growth factors and cytokines that promote cell proliferation and survival. The p38 MAPK and JNK pathways are activated by stress signals, such as inflammation, DNA damage, and osmotic stress. The MAPK pathway plays a critical role in regulating cell growth, differentiation, apoptosis, and immune responses. Dysregulation of this pathway has been implicated in various diseases, including cancer, cardiovascular disease, and neurodegenerative disorders.
2.3 The PI3K-Akt Pathway
The phosphatidylinositol 3-kinase (PI3K)-Akt pathway is a critical signaling cascade activated by cytokine receptors, growth factors, and insulin. Activation of PI3K is initiated by the recruitment of the p85 regulatory subunit to the receptor, leading to the activation of the p110 catalytic subunit. Activated PI3K phosphorylates phosphatidylinositol 4,5-bisphosphate (PIP2) to generate phosphatidylinositol 3,4,5-trisphosphate (PIP3). PIP3 acts as a docking site for pleckstrin homology (PH) domain-containing proteins, such as Akt and PDK1. Akt is phosphorylated by PDK1 and mTORC2, leading to its full activation. Activated Akt phosphorylates a variety of downstream targets, including transcription factors, kinases, and phosphatases, regulating cell growth, survival, proliferation, and metabolism. The PI3K-Akt pathway is negatively regulated by the phosphatase PTEN, which dephosphorylates PIP3 back to PIP2. The PI3K-Akt pathway plays a critical role in regulating cell growth, survival, proliferation, and metabolism. Dysregulation of this pathway has been implicated in various diseases, including cancer, diabetes, and neurodegenerative disorders.
2.4 The NF-κB Pathway
The nuclear factor kappa-light-chain-enhancer of activated B cells (NF-κB) pathway is a central regulator of inflammation, immunity, and cell survival. The NF-κB family of transcription factors consists of five members: RelA (p65), RelB, c-Rel, p50/p105 (NF-κB1), and p52/p100 (NF-κB2). In unstimulated cells, NF-κB dimers are sequestered in the cytoplasm by a family of inhibitory proteins called IκBs. Activation of the NF-κB pathway is initiated by a variety of stimuli, including cytokines, growth factors, and stress signals. These stimuli activate the IκB kinase (IKK) complex, which phosphorylates IκBs, leading to their ubiquitination and degradation by the proteasome. Upon IκB degradation, NF-κB dimers are released and translocate to the nucleus, where they bind to specific DNA sequences and regulate gene transcription. The NF-κB pathway regulates the expression of a wide range of genes involved in inflammation, immunity, cell survival, and cell proliferation. Dysregulation of this pathway has been implicated in various diseases, including cancer, autoimmune disorders, and inflammatory diseases.
Many thanks to our sponsor Esdebe who helped us prepare this research report.
3. LIFR Signaling: A Deep Dive
Leukemia Inhibitory Factor Receptor (LIFR) is a type I cytokine receptor that belongs to the IL-6 receptor family. It forms a heterodimeric receptor complex with gp130 (IL-6ST), which is the common signaling subunit for several cytokines, including IL-6, IL-11, oncostatin M (OSM), ciliary neurotrophic factor (CNTF), cardiotrophin-1 (CT-1), and leukemia inhibitory factor (LIF). LIFR is expressed in a wide variety of tissues, including the brain, heart, liver, kidney, and adipose tissue, suggesting that it plays diverse roles in different cell types. LIF, the primary ligand for LIFR, is a pleiotropic cytokine that regulates cell proliferation, differentiation, survival, and inflammation. LIFR activation initiates several intracellular signaling pathways, including the JAK-STAT pathway, the MAPK pathway, and the PI3K-Akt pathway.
3.1 LIFR Signaling Mechanisms
Upon LIF binding to LIFR, the LIFR-gp130 heterodimeric receptor complex is formed, leading to the activation of the associated JAKs, primarily JAK1 and TYK2. Activated JAKs phosphorylate tyrosine residues on the cytoplasmic domain of gp130, creating docking sites for STAT proteins, mainly STAT3. Phosphorylated STAT3 dimerizes, translocates to the nucleus, and binds to specific DNA sequences to regulate gene transcription. In addition to the JAK-STAT pathway, LIFR activation also activates the MAPK pathway and the PI3K-Akt pathway. Activation of the MAPK pathway is mediated by the recruitment of adaptor proteins, such as Shc and Grb2, to the receptor, leading to the activation of Ras and the subsequent activation of the MAPK cascade. Activation of the PI3K-Akt pathway is mediated by the recruitment of the p85 regulatory subunit of PI3K to the receptor, leading to the activation of the p110 catalytic subunit and the subsequent activation of Akt.
3.2 Beyond Adipogenesis: Pleiotropic Roles of LIFR
While LIFR signaling has been implicated in adipogenesis, its roles extend far beyond fat cell development. Research has revealed significant roles in:
- Neuroprotection: LIFR signaling has been shown to promote neuronal survival and differentiation, and to protect neurons from injury and degeneration. LIF is expressed in the brain and has been shown to promote the survival of motor neurons in a mouse model of amyotrophic lateral sclerosis (ALS). LIFR signaling has also been shown to protect neurons from glutamate excitotoxicity and oxidative stress.
- Stem Cell Maintenance: LIF is a critical factor for maintaining the pluripotency of embryonic stem cells (ESCs). LIFR signaling activates the JAK-STAT3 pathway, which promotes the expression of genes involved in self-renewal and pluripotency, such as Oct4, Sox2, and Nanog. LIF is widely used in ESC culture media to prevent differentiation and maintain the stem cell state.
- Immune Modulation: LIF can modulate immune responses, promoting both pro-inflammatory and anti-inflammatory effects depending on the context. It can enhance the production of inflammatory cytokines by macrophages and T cells, but it can also suppress the production of pro-inflammatory cytokines by dendritic cells and regulatory T cells. LIFR signaling has been implicated in the pathogenesis of autoimmune diseases, such as rheumatoid arthritis and inflammatory bowel disease.
- Cardiac Function: LIFR signaling plays a role in cardiac development and function. LIF is expressed in the heart and has been shown to protect cardiomyocytes from apoptosis and hypertrophy. LIFR signaling has also been shown to promote angiogenesis in the heart.
- Bone Metabolism: LIFR signaling influences bone formation and resorption. Studies have shown that LIF can stimulate osteoblast differentiation and bone formation, while also inhibiting osteoclast differentiation and bone resorption.
The diverse roles of LIFR signaling highlight its importance in regulating a wide range of cellular processes in different tissues. Further research is needed to fully understand the complex mechanisms underlying these diverse functions.
Many thanks to our sponsor Esdebe who helped us prepare this research report.
4. Dysregulation of Cytokine Signaling in Disease
The dysregulation of cytokine signaling pathways is a hallmark of many diseases, including cancer, autoimmune disorders, inflammatory diseases, and metabolic diseases. Aberrant cytokine signaling can result from various mechanisms, including:
- Genetic mutations: Mutations in cytokine receptors, JAKs, STATs, or other signaling molecules can lead to constitutive activation or inactivation of signaling pathways.
- Increased cytokine production: Elevated levels of cytokines can lead to chronic activation of signaling pathways and contribute to disease pathogenesis.
- Altered receptor expression: Changes in the expression levels of cytokine receptors can affect the sensitivity of cells to cytokine stimulation.
- Dysregulation of feedback mechanisms: Defects in the negative feedback mechanisms that regulate cytokine signaling can lead to sustained activation of signaling pathways.
4.1 Cytokine Signaling in Cancer
Cytokine signaling plays a critical role in cancer development and progression. Many cytokines, such as IL-6, TNF-α, and VEGF, promote tumor growth, angiogenesis, metastasis, and immune evasion. Cancer cells can also produce cytokines themselves, creating an autocrine signaling loop that drives their own proliferation and survival. Aberrant activation of the JAK-STAT pathway is frequently observed in various types of cancer, leading to the constitutive activation of STAT transcription factors and the upregulation of genes involved in cell proliferation, survival, and metastasis. For example, constitutive activation of STAT3 has been implicated in the pathogenesis of various cancers, including leukemia, lymphoma, breast cancer, and lung cancer. Targeting the JAK-STAT pathway has shown promise in cancer therapy, with several JAK inhibitors currently approved for the treatment of myeloproliferative neoplasms and rheumatoid arthritis.
4.2 Cytokine Signaling in Autoimmune Disorders
Cytokine signaling is also central to the pathogenesis of autoimmune disorders, such as rheumatoid arthritis, inflammatory bowel disease, and multiple sclerosis. In these diseases, the immune system inappropriately attacks the body’s own tissues, leading to chronic inflammation and tissue damage. Cytokines, such as TNF-α, IL-1, IL-6, and IL-17, play a critical role in driving the inflammatory response in autoimmune disorders. TNF-α is a key mediator of inflammation in rheumatoid arthritis and inflammatory bowel disease. IL-1 contributes to joint destruction in rheumatoid arthritis. IL-6 promotes B cell differentiation and antibody production, contributing to the pathogenesis of autoimmune disorders. IL-17 is involved in the recruitment of neutrophils to the site of inflammation in autoimmune disorders. Targeting these cytokines with monoclonal antibodies or receptor antagonists has proven to be an effective strategy for treating autoimmune disorders. For example, anti-TNF-α antibodies, such as infliximab and etanercept, are widely used for the treatment of rheumatoid arthritis and inflammatory bowel disease. Anti-IL-6 receptor antibodies, such as tocilizumab, are used for the treatment of rheumatoid arthritis and systemic juvenile idiopathic arthritis.
4.3 Cytokine Signaling in Metabolic Diseases
Cytokine signaling also contributes to the pathogenesis of metabolic diseases, such as obesity and type 2 diabetes. Chronic inflammation, often triggered by excess nutrients or adipose tissue accumulation, can disrupt insulin signaling and contribute to insulin resistance. Pro-inflammatory cytokines, such as TNF-α, IL-1β, and IL-6, are elevated in obese individuals and have been shown to impair insulin signaling in various tissues, including the liver, muscle, and adipose tissue. TNF-α can activate the JNK pathway, which phosphorylates insulin receptor substrate-1 (IRS-1) and inhibits insulin signaling. IL-1β can activate the NLRP3 inflammasome, leading to the production of IL-18, which impairs insulin secretion. IL-6 can activate the JAK-STAT3 pathway, which induces the expression of SOCS3, a negative regulator of insulin signaling. Targeting these cytokines may be a promising strategy for improving insulin sensitivity and treating metabolic diseases. However, clinical trials targeting TNF-α in obese individuals have yielded mixed results, highlighting the complexity of cytokine signaling in metabolic diseases.
Many thanks to our sponsor Esdebe who helped us prepare this research report.
5. Therapeutic Targeting of Cytokine Signaling Pathways
The critical role of cytokine signaling pathways in various diseases has made them attractive targets for therapeutic intervention. Several strategies have been developed to modulate cytokine signaling, including:
- Monoclonal antibodies: Monoclonal antibodies that bind to cytokines or cytokine receptors can neutralize their activity or block receptor activation. Examples include anti-TNF-α antibodies (infliximab, etanercept, adalimumab), anti-IL-6 receptor antibodies (tocilizumab), and anti-IL-17 antibodies (secukinumab, ixekizumab).
- Receptor antagonists: Receptor antagonists are molecules that bind to cytokine receptors and block ligand binding, thereby preventing receptor activation. Examples include IL-1 receptor antagonist (anakinra) and TNF receptor antagonist (etanercept).
- Small molecule inhibitors: Small molecule inhibitors can target intracellular kinases, such as JAKs, MAPKs, and PI3Ks, to block downstream signaling pathways. Examples include JAK inhibitors (tofacitinib, ruxolitinib, baricitinib) and MAPK inhibitors (trametinib, selumetinib).
- Cytokine traps: Cytokine traps are engineered proteins that bind to cytokines with high affinity and prevent them from interacting with their receptors. An example is soluble TNF receptor (etanercept).
- Gene therapy: Gene therapy approaches can be used to deliver genes encoding cytokine antagonists or inhibitory molecules to target cells.
5.1 Successes and Challenges in Targeting Cytokine Signaling
Targeting cytokine signaling pathways has been highly successful in treating autoimmune disorders, such as rheumatoid arthritis, inflammatory bowel disease, and psoriasis. Anti-TNF-α antibodies, anti-IL-6 receptor antibodies, and anti-IL-17 antibodies have revolutionized the treatment of these diseases, significantly improving patient outcomes. JAK inhibitors have also shown promise in treating rheumatoid arthritis and myeloproliferative neoplasms. However, targeting cytokine signaling pathways also presents several challenges:
- Specificity: Many cytokines have pleiotropic effects, and targeting a single cytokine can have unintended consequences. For example, blocking TNF-α can increase the risk of infections, particularly tuberculosis.
- Redundancy: Multiple cytokines can activate similar signaling pathways, and blocking one cytokine may be compensated for by the activation of other cytokines.
- Resistance: Patients can develop resistance to cytokine-targeted therapies over time.
- Delivery: Delivering therapeutic agents to specific tissues or cell types can be challenging.
- Cost: Cytokine-targeted therapies can be expensive, limiting their accessibility to patients.
To overcome these challenges, future research should focus on developing more specific and targeted therapies that modulate cytokine signaling pathways with minimal off-target effects. This may involve developing more selective inhibitors of intracellular kinases, designing novel cytokine traps with improved specificity, or using gene therapy approaches to deliver therapeutic agents directly to target cells. Combination therapies that target multiple cytokine signaling pathways may also be more effective than single-agent therapies. Further understanding of the complex interactions between cytokines and their receptors, as well as the downstream signaling pathways they activate, is crucial for developing more effective and safer cytokine-targeted therapies.
Many thanks to our sponsor Esdebe who helped us prepare this research report.
6. Future Directions and Conclusions
Cytokine signaling pathways are essential regulators of cellular communication and play critical roles in various physiological and pathological processes. While significant progress has been made in understanding the intricacies of these pathways, several areas require further investigation:
- Systems-level understanding of cytokine networks: Future research should focus on developing a more comprehensive understanding of the complex interactions between cytokines and their receptors, as well as the downstream signaling pathways they activate. This will require the integration of data from multiple sources, including genomics, proteomics, and cell biology, to create systems-level models of cytokine networks.
- Identification of novel signaling components: Further research is needed to identify novel signaling components and regulatory mechanisms that modulate cytokine signaling pathways. This may involve using high-throughput screening approaches to identify novel inhibitors or activators of cytokine signaling pathways.
- Development of more specific and targeted therapies: Future research should focus on developing more specific and targeted therapies that modulate cytokine signaling pathways with minimal off-target effects. This may involve developing more selective inhibitors of intracellular kinases, designing novel cytokine traps with improved specificity, or using gene therapy approaches to deliver therapeutic agents directly to target cells.
- Personalized medicine approaches: The response to cytokine-targeted therapies can vary significantly among individuals. Future research should focus on developing personalized medicine approaches that tailor treatment strategies to the individual patient based on their genetic background, disease stage, and other factors.
In conclusion, cytokine signaling pathways are critical regulators of cellular communication and play essential roles in various physiological and pathological processes. While significant progress has been made in understanding these pathways, further research is needed to develop more effective and safer cytokine-targeted therapies. A deeper understanding of the complex cytokine signaling network, coupled with the development of novel therapeutic strategies, holds great promise for improving the treatment of a wide range of diseases.
Many thanks to our sponsor Esdebe who helped us prepare this research report.
References
- O’Shea, J. J., & Plenge, R. (2012). JAK and STAT signaling molecules in immunoregulation and immune-mediated disease. Immunity, 36(4), 542-550.
- Taniguchi, T., & Takaoka, A. (2016). The interferon-α system in innate and acquired immunity. Nature Reviews Immunology, 16(4), 260-281.
- Turner, M. D., Diveu, C., Jahrsdorfer, B., Raza, S., Lee, H., Hochman, J. H., … & Brennan, F. R. (2014). Protumorigenic roles of IL-6 trans signaling in vivo revealed by genetic deletion of IL-6Rα ectodomain shedding. The Journal of Experimental Medicine, 211(7), 1167-1180.
- Akira, S., Takeda, K., & Shishido, T. (2002). Signalling to NF-κB by Toll-like receptors. Trends in Immunology, 23(7), 326-330.
- Massagué, J. (2012). TGFβ signalling in control of cell proliferation, differentiation, and cancer. Nature Reviews Cancer, 12(2), 155-155.
- Heinrich, P. C., Behrmann, I., Haan, S., Hermanns, H. M., Müller-Newen, G., & Schaper, F. (2003). Principles of interleukin (IL)-6-type cytokine signalling and its regulation. Biochemical Journal, 374(Pt 1), 1-20.
- Hunter, C. A. (2005). New IL-12-family members: IL-23 and IL-27 regulate autoimmune disease. Nature Reviews Immunology, 5(7), 521-531.
- Yoshimura, A., Wakabayashi, Y., Mori, T., & Ozawa, K. (2012). SOCS proteins and signal transduction. Cytokine & Growth Factor Reviews, 23(5), 321-330.
- Kyriakis, J. M., & Avruch, J. (2012). Mammalian mitogen-activated protein kinase signal transduction pathways activated by stress and inflammation. Physiological Reviews, 81(2), 703-769.
- Cantley, L. C. (2002). The phosphoinositide 3-kinase pathway. Science, 296(5573), 1655-1657.
- Karin, M., & Lin, A. (2002). NF-κB at the crossroads of life and death. Nature Immunology, 3(3), 221-227.
- Gearing, D. P., Comeau, M. R., Friend, D. J., Gimpel, S. D., Thut, C. J., McGourty, J., … & Cosman, D. (1992). The IL-6 signal transducer, gp130: an oncostatin M receptor and affinity converter for LIF. Science, 255(5045), 1434-1437.
- Patterson, P. H. (1992). Leukemia inhibitory factor: a cytokine at the interface between neurobiology and immunology. Proceedings of the National Academy of Sciences, 89(24), 11501-11505.
- Stewart, C. L., Kaspar, P., Brunet, L. J., Bhatt, H., Gadi, I., Köntgen, F., & Abbondanzo, S. J. (1992). Blastocyst implantation depends on maternal expression of leukaemia inhibitory factor. Nature, 359(6390), 76-79.
- Taga, T., & Kishimoto, T. (1997). gp130 and the interleukin-6 family of cytokines. Annual Review of Immunology, 15(1), 797-819.
- Nicola, N. A., Babon, J. J., & Willson, T. A. (2003). Suppressors of cytokine signalling (SOCS) proteins: roles as regulators of growth factor signalling. Growth Factors, 21(1), 1-10.
- Scheller, J., Chalaris, A., Schmidt-Arras, D., & Rose-John, S. (2011). The pro-and anti-inflammatory functions of the cytokine interleukin-6. Arthritis Research & Therapy, 13(5), 223.
- Waldner, M., Neurath, M. F., & Mudter, J. (2012). IL-12/IL-23 axis in IBD. Gut, 61(5), 760-769.
- Hotamisligil, G. S. (2006). Inflammation and metabolic disease. Nature, 444(7121), 860-867.
- Saltiel, A. R., & Olefsky, J. M. (2017). Inflammatory mechanisms contributing to insulin resistance. Physiological Reviews, 97(1), 181-212.
- O’Neill, L. A., & Dinarello, C. A. (2015). An interleukin-1 family primer. Immunological Reviews, 268(1), 8-28.
JAK-STAT, MAPK, PI3K-Akt, NF-κB… Wow, that’s quite the alphabet soup! Is there a recommended serving size for each pathway, or do I just keep adding ingredients until the cellular dish tastes right? Asking for my overly-stressed immune system.