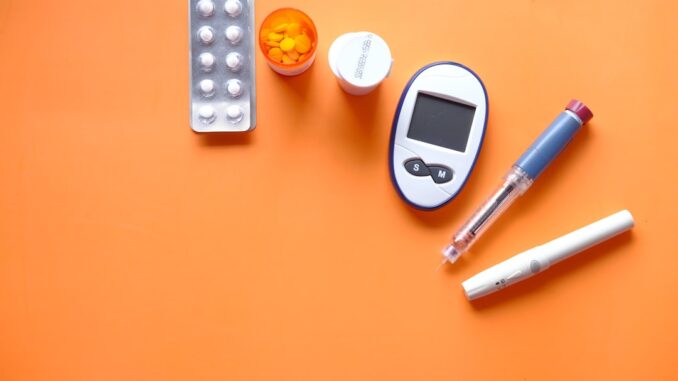
The Multifaceted Role of Glucose: Beyond Diabetes and into Personalized Physiology
Abstract
Glucose, a ubiquitous monosaccharide, is fundamentally recognized as the primary energy source for cellular metabolism. While its dysregulation is widely associated with diabetes mellitus and related metabolic disorders, the significance of glucose extends far beyond these pathological states. This report provides a comprehensive overview of glucose metabolism, encompassing its intricate biochemical pathways, hormonal regulation, and diverse physiological roles. We critically examine the implications of varying glucose levels on human health, including the long-term consequences of both hyperglycemia and hypoglycemia. Furthermore, we delve into the burgeoning field of continuous glucose monitoring (CGM) and its applications in athletic performance, cognitive function, and personalized health optimization. This report explores the potential and challenges of utilizing glucose data for proactive health management and aims to provide a nuanced perspective on the multifaceted role of glucose in human physiology.
1. Introduction
Glucose, a six-carbon sugar (C6H12O6), is a vital fuel source for all living organisms. Its central role in energy production stems from its relatively simple structure and ease of catabolism through glycolysis, the tricarboxylic acid (TCA) cycle, and oxidative phosphorylation. The human body tightly regulates glucose homeostasis to ensure a constant supply of energy for tissues, particularly the brain, which relies almost exclusively on glucose under normal conditions. The liver, pancreas, and kidneys play crucial roles in maintaining this delicate balance. While glucose metabolism is often studied in the context of diabetes, its influence permeates virtually every aspect of human health, including cognitive function, immune response, and cardiovascular health [1].
Traditionally, glucose monitoring has been reactive, primarily aimed at managing diabetes. However, advances in technology, particularly the development of CGMs, are revolutionizing our understanding of glucose dynamics in healthy individuals. These devices provide real-time glucose data, enabling personalized interventions to optimize metabolic health and performance. This report will explore these advancements and their potential to shift the focus from disease management to proactive health optimization.
2. Glucose Metabolism: A Complex Network
Glucose metabolism is a complex interplay of biochemical pathways that can be broadly divided into anabolism (synthesis) and catabolism (breakdown). Catabolic pathways extract energy from glucose, while anabolic pathways utilize glucose to build larger molecules. The key pathways involved include:
- Glycolysis: The breakdown of glucose into pyruvate, generating a small amount of ATP and NADH. This process occurs in the cytoplasm and does not require oxygen.
- Gluconeogenesis: The synthesis of glucose from non-carbohydrate precursors, such as amino acids, lactate, and glycerol. This occurs primarily in the liver and kidneys.
- Glycogenesis: The synthesis of glycogen, a storage form of glucose, primarily in the liver and muscles.
- Glycogenolysis: The breakdown of glycogen into glucose.
- Pentose Phosphate Pathway (PPP): This pathway produces NADPH, essential for reducing oxidative stress and synthesizing fatty acids, and ribose-5-phosphate, a precursor for nucleotide biosynthesis.
- Tricarboxylic Acid Cycle (TCA Cycle) / Krebs Cycle: Pyruvate is converted to acetyl-CoA, which enters the TCA cycle, generating ATP, NADH, and FADH2.
- Oxidative Phosphorylation: The final stage of glucose metabolism, where NADH and FADH2 donate electrons to the electron transport chain, driving the synthesis of a large amount of ATP.
2.1 Hormonal Regulation of Glucose Metabolism
The intricate balance of glucose metabolism is tightly regulated by hormones, primarily insulin and glucagon. Insulin, secreted by the pancreatic β-cells, is released in response to elevated blood glucose levels. It promotes glucose uptake by cells, stimulates glycogen synthesis, and inhibits gluconeogenesis. Glucagon, secreted by the pancreatic α-cells, acts antagonistically to insulin. It is released in response to low blood glucose levels and stimulates glycogenolysis and gluconeogenesis in the liver, increasing blood glucose levels. Other hormones, such as cortisol, epinephrine, and growth hormone, also influence glucose metabolism by increasing glucose production and decreasing glucose utilization [2].
The interplay between these hormones ensures that blood glucose levels remain within a narrow physiological range, typically between 70 and 140 mg/dL. Disruptions in this hormonal regulation, as seen in diabetes, can lead to chronic hyperglycemia or hypoglycemia.
2.2 Tissue-Specific Glucose Metabolism
Glucose metabolism varies significantly across different tissues. The brain, for example, primarily relies on glucose as its energy source and has a high glucose uptake rate. Skeletal muscle utilizes glucose during exercise and stores glucose as glycogen. The liver plays a central role in regulating blood glucose levels by storing and releasing glucose as needed. Adipose tissue takes up glucose and converts it into triglycerides for storage. Understanding these tissue-specific differences is crucial for comprehending the systemic effects of glucose dysregulation.
3. Glucose Monitoring Technologies: From Fingersticks to Continuous Insights
Monitoring blood glucose levels is essential for managing diabetes and increasingly important for understanding individual metabolic responses in a broader context. The available technologies range from traditional methods to advanced continuous monitoring systems.
-
Self-Monitoring of Blood Glucose (SMBG): This involves pricking a finger with a lancet and applying a drop of blood to a test strip, which is then read by a glucose meter. SMBG provides a snapshot of glucose levels at a specific time. It is widely used for managing diabetes and allows individuals to adjust their insulin doses or dietary intake accordingly.
-
Continuous Glucose Monitoring (CGM): CGMs use a small sensor inserted under the skin to measure glucose levels in interstitial fluid. These devices provide continuous glucose readings, typically every 5 to 15 minutes, generating a detailed glucose profile over time. CGMs also provide alerts for high and low glucose levels, enabling proactive intervention. Two main types of CGMs exist: real-time CGMs (rt-CGM), which transmit glucose data continuously to a receiver or smartphone, and intermittently scanned CGMs (isCGM), which require manual scanning to retrieve glucose data.
-
Flash Glucose Monitoring (FGM): This is a type of isCGM that measures glucose levels in the interstitial fluid and requires scanning the sensor to obtain readings. Unlike rt-CGMs, FGM does not provide real-time alerts for high or low glucose levels.
3.1 Advantages and Limitations of Different Monitoring Technologies
SMBG is relatively inexpensive and readily available, but it only provides a snapshot of glucose levels at a specific point in time. CGMs provide a more comprehensive picture of glucose dynamics, revealing patterns and trends that SMBG cannot capture. This allows for more informed decision-making regarding diet, exercise, and medication management. However, CGMs can be more expensive and require initial calibration with SMBG readings. Furthermore, there can be a lag between interstitial fluid glucose levels and blood glucose levels, which can affect the accuracy of CGM readings, especially during rapid glucose fluctuations [3].
3.2 The Rise of CGM for Non-Diabetics
Traditionally, CGMs were primarily used by individuals with diabetes. However, there is a growing interest in using CGMs for non-diabetic individuals to optimize metabolic health and performance. CGMs can provide valuable insights into how different foods, activities, and stressors affect glucose levels, allowing individuals to make personalized lifestyle adjustments. For example, athletes may use CGMs to optimize their carbohydrate intake and training schedules to improve performance. Individuals seeking to improve their overall health may use CGMs to identify foods that cause glucose spikes and adjust their diets accordingly.
4. Factors Affecting Glucose Levels: A Web of Interconnected Variables
Numerous factors influence glucose levels, including diet, exercise, stress, sleep, and underlying medical conditions. Understanding these factors is crucial for effective glucose management and optimization.
-
Diet: Carbohydrates are the primary dietary source of glucose. The type and amount of carbohydrates consumed significantly impact blood glucose levels. Foods with a high glycemic index (GI) and glycemic load (GL) cause rapid spikes in blood glucose, while foods with a low GI and GL are digested more slowly and have a less pronounced effect on blood glucose. Protein and fat can also influence glucose levels, albeit to a lesser extent than carbohydrates. Dietary fiber can slow down glucose absorption and improve insulin sensitivity [4].
-
Exercise: Physical activity increases glucose uptake by muscles, lowering blood glucose levels. Exercise also improves insulin sensitivity, making cells more responsive to insulin. Both aerobic and resistance exercise can have beneficial effects on glucose metabolism, but the optimal type and intensity of exercise may vary depending on individual factors.
-
Stress: Stress hormones, such as cortisol and epinephrine, can increase blood glucose levels by stimulating gluconeogenesis and glycogenolysis. Chronic stress can lead to insulin resistance and impaired glucose tolerance.
-
Sleep: Inadequate sleep can disrupt glucose metabolism and increase insulin resistance. Sleep deprivation can also lead to increased levels of stress hormones, further exacerbating glucose dysregulation.
-
Medications: Certain medications, such as corticosteroids and some diuretics, can increase blood glucose levels. Other medications, such as metformin and insulin, are used to lower blood glucose levels in individuals with diabetes.
-
Underlying Medical Conditions: Various medical conditions, such as infections, liver disease, and kidney disease, can affect glucose metabolism.
4.1 The Gut Microbiome and Glucose Regulation
The gut microbiome, the community of microorganisms residing in the digestive tract, is increasingly recognized as a key regulator of glucose metabolism. Certain gut bacteria can influence glucose absorption, insulin sensitivity, and inflammation. Dysbiosis, an imbalance in the gut microbiome, has been linked to insulin resistance and an increased risk of type 2 diabetes. Diet is a major determinant of gut microbiome composition, highlighting the importance of dietary interventions in promoting gut health and improving glucose metabolism. Emerging research suggests that probiotics and prebiotics may have beneficial effects on glucose regulation by modulating the gut microbiome [5].
5. Long-Term Health Consequences of Poorly Managed Glucose Levels
Chronic hyperglycemia, a hallmark of diabetes, can lead to a wide range of long-term health complications, affecting virtually every organ system. These complications include:
-
Cardiovascular Disease: Hyperglycemia promotes atherosclerosis, increasing the risk of heart attacks, strokes, and peripheral artery disease.
-
Neuropathy: Nerve damage caused by hyperglycemia can lead to pain, numbness, and loss of sensation, particularly in the feet and hands.
-
Nephropathy: Kidney damage caused by hyperglycemia can lead to chronic kidney disease and eventually kidney failure.
-
Retinopathy: Damage to the blood vessels in the retina can lead to vision loss and blindness.
-
Foot Ulcers: Nerve damage and poor circulation increase the risk of foot ulcers, which can be difficult to heal and may require amputation.
5.1 The Significance of Glucose Variability
Beyond average glucose levels, glucose variability, the fluctuations in glucose levels over time, is also emerging as an important predictor of long-term health outcomes. High glucose variability has been linked to increased oxidative stress, inflammation, and endothelial dysfunction. Studies have shown that individuals with greater glucose variability have a higher risk of cardiovascular disease and other complications, even when their average glucose levels are within the target range [6]. Therefore, minimizing glucose variability is an important goal in managing diabetes and optimizing metabolic health.
5.2 Hypoglycemia: An Often Overlooked Consequence
While hyperglycemia receives considerable attention, hypoglycemia (low blood glucose) can also have significant health consequences, particularly for individuals with diabetes who are taking insulin or other glucose-lowering medications. Hypoglycemia can cause symptoms such as confusion, dizziness, sweating, and seizures. Severe hypoglycemia can lead to coma and even death. Recurrent hypoglycemia can also impair cognitive function and increase the risk of falls. It’s critical that patients, clinicians, and researchers continue to develop solutions to reduce hypoglycemic risk for those patients requiring insulin therapies.
6. Glucose Monitoring for Athletes: Optimizing Performance and Recovery
Athletes are increasingly using CGMs to optimize their training and nutrition. CGMs can provide valuable insights into how different types of exercise, carbohydrate intake, and recovery strategies affect glucose levels. This information can be used to fine-tune training schedules and dietary plans to improve performance and prevent fatigue.
-
Fueling Strategies: CGMs can help athletes determine the optimal carbohydrate intake before, during, and after exercise. By monitoring glucose levels in real-time, athletes can avoid glucose spikes and crashes that can impair performance.
-
Training Periodization: CGMs can be used to optimize training periodization by monitoring glucose levels during different phases of training. This can help athletes identify periods of overtraining and adjust their training schedules accordingly.
-
Recovery Strategies: CGMs can be used to optimize recovery strategies by monitoring glucose levels after exercise. This can help athletes determine the optimal carbohydrate intake and timing to replenish glycogen stores and promote muscle recovery.
6.1 The Impact of Glucose on Cognitive Function in Athletes
Maintaining stable glucose levels is crucial for optimal cognitive function, particularly during prolonged or intense exercise. Hypoglycemia can impair cognitive performance, leading to reduced reaction time, impaired decision-making, and decreased focus. CGMs can help athletes maintain stable glucose levels during exercise, ensuring optimal cognitive function and performance. Research is ongoing to better understand the complex interplay between glucose, cognitive function, and athletic performance [7].
7. Personalized Physiology: The Future of Glucose Monitoring
The future of glucose monitoring lies in personalized physiology, where glucose data is integrated with other physiological data, such as heart rate, sleep patterns, and activity levels, to provide a holistic view of individual metabolic health. This integrated data can be used to develop personalized interventions to optimize health and performance. For example, machine learning algorithms can be used to predict glucose responses to different foods and activities, allowing individuals to make more informed choices about their diet and lifestyle.
7.1 Challenges and Opportunities
While the potential of personalized physiology is immense, there are also several challenges that need to be addressed. These include:
-
Data Privacy and Security: Ensuring the privacy and security of personal health data is crucial. Robust security measures must be in place to protect data from unauthorized access.
-
Data Interpretation: Interpreting complex glucose data requires expertise. Healthcare professionals need to be trained to effectively utilize glucose data to guide personalized interventions.
-
Accessibility: Making advanced glucose monitoring technologies accessible to a wider population is important. The cost of CGMs and other monitoring devices can be a barrier for many individuals.
7.2 The Potential of Artificial Pancreas Systems
Artificial pancreas systems (APS), also known as closed-loop systems, combine CGM technology with an insulin pump and a control algorithm to automatically regulate blood glucose levels. These systems have shown great promise in improving glucose control and reducing the risk of hypoglycemia and hyperglycemia in individuals with type 1 diabetes. As technology advances, APS are becoming more sophisticated and user-friendly, offering the potential to significantly improve the quality of life for individuals with diabetes [8].
8. Conclusion
Glucose is a fundamental molecule with a profound impact on human health and performance. While its dysregulation is a hallmark of diabetes, its influence extends far beyond this disease. Advances in glucose monitoring technologies, particularly CGMs, are revolutionizing our understanding of glucose dynamics and enabling personalized interventions to optimize metabolic health. By integrating glucose data with other physiological data, we can gain a more holistic view of individual metabolic responses and develop targeted strategies to improve health and performance. As we continue to unravel the complexities of glucose metabolism, we can expect to see even more innovative applications of glucose monitoring in the future, ultimately leading to a more proactive and personalized approach to healthcare.
References
[1] Berg, J. M., Tymoczko, J. L., & Stryer, L. (2002). Biochemistry. 5th edition. New York: W H Freeman.
[2] Powers, A. C. (2020). Diabetes mellitus: diagnosis, classification, and pathophysiology. Endotext.
[3] Klonoff, D. C., Messer, L. H., Umpierrez, G. E., Parkin, C. G., & Walsh, J. (2017). Standard Parkes Error Grid Analysis of Continuous Glucose Monitoring System Data. Journal of Diabetes Science and Technology, 11(3), 491–497.
[4] Brand-Miller, J. C., Hayne, S., Petocz, P., & Colagiuri, S. (2003). Glycemic index and glycemic load for foods: a systematic review. The American Journal of Clinical Nutrition, 71(6), 1455-1492.
[5] Cani, P. D. (2018). Gut microbiota – at the heart of everything? Nature Reviews Gastroenterology & Hepatology, 14(11), 589-590.
[6] Frontoni, S., Desideri, G., & Gambaro, G. (2015). Glucose variability: an emerging target for the treatment of diabetes mellitus. Diabetes Research and Clinical Practice, 114, 207-215.
[7] Taylor, L., Rauch, J., & Sporer, B. (2023). Continuous Glucose Monitoring in Sport: A Narrative Review. Nutrients, 15(7), 1644.
[8] Bekiari, E., Kitsios, K., Touloumi, G., Tsalikian, E., Beck, R. W., & Laffel, L. M. (2018). Artificial pancreas treatment for outpatients with type 1 diabetes: systematic review and meta-analysis. BMJ, 361, k1310.
The mention of the gut microbiome’s role in glucose regulation is intriguing. Could you elaborate on the specific bacterial species or metabolic pathways within the microbiome that exert the most significant influence on insulin sensitivity and glucose homeostasis?
That’s a great question! Research is rapidly evolving in that area. Some studies suggest Akkermansia muciniphila and certain butyrate-producing bacteria play key roles in improving insulin sensitivity and glucose metabolism. Their metabolic pathways, like short-chain fatty acid production, seem to be very important. This is definitely an area to watch for future breakthroughs!
Editor: MedTechNews.Uk
Thank you to our Sponsor Esdebe
So, if I understand correctly, soon we’ll all be obsessively checking our glucose like it’s the stock market? I can see it now: “Honey, did you see my glucose just spiked after that banana? Time to short sell my fruit intake!”
That’s a hilarious analogy! It’s true; with increasing glucose data, we might start viewing food choices as investments. Understanding how different foods affect our glucose levels empowers us to make informed decisions, but let’s hope it doesn’t lead to *too* much “glucose trading”! Thanks for the fun perspective.
Editor: MedTechNews.Uk
Thank you to our Sponsor Esdebe
So, if our gut’s microbiome dictates glucose absorption, and diet alters that microbiome, are we essentially outsourcing our willpower to bacteria? Are we just highly evolved yogurt commercials in disguise?