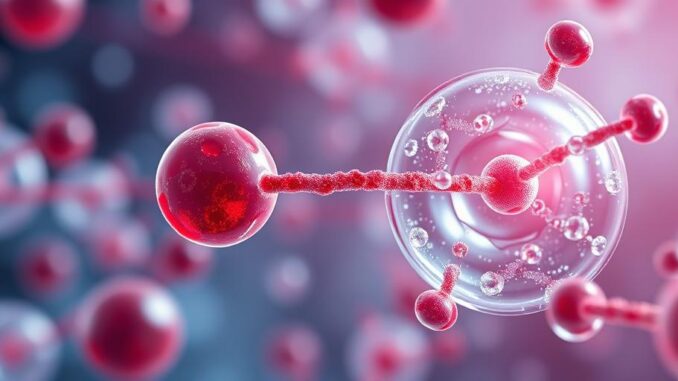
Abstract
Hemoglobin (Hb), a tetrameric metalloprotein residing within erythrocytes, is renowned for its critical role in oxygen (O₂) transport from the lungs to peripheral tissues and the facilitation of carbon dioxide (CO₂) removal. However, the functional significance of Hb extends far beyond its respiratory duties. This report delves into the intricate biochemistry of Hb, exploring its allosteric regulation, the structural dynamics associated with oxygen binding, and the implications of these properties in physiological and pathological contexts. We examine the various Hb derivatives, including oxyhemoglobin (HbO₂), deoxyhemoglobin (Hb), methemoglobin (MetHb), and carboxyhemoglobin (HbCO), highlighting their distinct characteristics and clinical relevance. Furthermore, we discuss the impact of genetic mutations and environmental factors on Hb structure and function, focusing on diseases such as sickle cell anemia and thalassemia. Finally, we explore the emerging role of Hb and its derivatives as biomarkers and therapeutic targets, particularly in the fields of medical imaging, drug delivery, and redox-based therapies. The intricate interplay between Hb structure, function, and its environment underscores its potential as a key player in both understanding and addressing a wide range of human diseases.
Many thanks to our sponsor Esdebe who helped us prepare this research report.
1. Introduction
Hemoglobin (Hb) is arguably one of the most extensively studied proteins in biochemistry and physiology. Its primary function, the reversible binding and transport of oxygen, is essential for aerobic life. However, viewing Hb solely as an oxygen carrier represents a significant underestimation of its biological importance. Hb acts as a sensitive sensor of the cellular microenvironment, modulating its structure and function in response to changes in pH, CO₂, and other factors. This allosteric regulation enables Hb to optimize oxygen delivery to tissues with varying metabolic demands. The complex structure of Hb, composed of four globin subunits (typically two α and two β chains in adult HbA), each containing a heme group with a central iron atom, provides the foundation for its diverse functions. The intricate interplay between the heme iron, the globin protein, and the surrounding environment dictates the oxygen-binding affinity and cooperativity of Hb. Disruptions to Hb structure or function, whether due to genetic mutations, environmental toxins, or disease processes, can have profound clinical consequences. Understanding the multifaceted roles of Hb is crucial for developing effective diagnostic and therapeutic strategies for a wide range of disorders.
Many thanks to our sponsor Esdebe who helped us prepare this research report.
2. Biochemistry and Structure of Hemoglobin
The Hb molecule is a heterotetramer consisting of two α-globin chains (141 amino acids each) and two β-globin chains (146 amino acids each) in adult HbA. Each globin chain is associated with a heme moiety, a protoporphyrin ring complexed with a ferrous iron (Fe²⁺) atom. The heme group is responsible for binding oxygen. The iron atom binds oxygen reversibly, without undergoing oxidation to the ferric state (Fe³⁺), which would render it incapable of oxygen binding. The globin protein environment plays a critical role in maintaining the iron in the ferrous state and modulating its oxygen-binding affinity.
The three-dimensional structure of Hb is crucial for its function. The α and β subunits have similar tertiary structures, each consisting of eight α-helices arranged in a characteristic globin fold. The heme group resides in a hydrophobic pocket within the globin chain, shielded from solvent exposure. The iron atom is coordinated by four nitrogen atoms from the porphyrin ring and a nitrogen atom from the histidine residue at the proximal position (His F8). The sixth coordination site of the iron atom is available for binding oxygen. Upon oxygen binding, the iron atom moves slightly into the plane of the porphyrin ring, triggering a conformational change in the globin subunit. This conformational change is transmitted to the other subunits, influencing their oxygen-binding affinity, a phenomenon known as cooperativity.
Cooperativity is a key feature of Hb function, allowing it to efficiently load oxygen in the lungs, where oxygen tension is high, and unload oxygen in the tissues, where oxygen tension is low. The oxygen-binding curve of Hb is sigmoidal, reflecting the cooperative binding of oxygen. The sigmoidal curve is more efficient at delivering oxygen to tissues than a hyperbolic curve would be. The molecular basis of cooperativity involves allosteric transitions between two major conformational states: the T (tense) state and the R (relaxed) state. The T state has a low affinity for oxygen, while the R state has a high affinity. Oxygen binding promotes the transition from the T state to the R state, increasing the oxygen-binding affinity of the remaining subunits. Several factors influence the allosteric equilibrium between the T and R states, including pH, CO₂, and 2,3-bisphosphoglycerate (2,3-BPG).
Many thanks to our sponsor Esdebe who helped us prepare this research report.
3. Hemoglobin Derivatives and Their Clinical Significance
Several Hb derivatives exist, each with distinct properties and clinical implications:
-
Oxyhemoglobin (HbO₂): The oxygen-bound form of Hb. It is responsible for carrying oxygen from the lungs to the tissues. The color of HbO₂ is bright red, giving arterial blood its characteristic appearance.
-
Deoxyhemoglobin (Hb): The oxygen-unbound form of Hb. It is the form of Hb that returns to the lungs after delivering oxygen to the tissues. The color of deoxyhemoglobin is dark purple.
-
Methemoglobin (MetHb): Hb in which the iron atom is in the ferric (Fe³⁺) state. MetHb cannot bind oxygen. Elevated levels of MetHb can lead to tissue hypoxia. Methemoglobinemia can be caused by genetic mutations, exposure to oxidizing agents (e.g., nitrates, certain drugs), or congenital enzyme deficiencies.
-
Carboxyhemoglobin (HbCO): Hb bound to carbon monoxide (CO). CO binds to Hb with an affinity approximately 200-250 times greater than that of oxygen. HbCO is unable to bind oxygen, effectively blocking oxygen transport. Carbon monoxide poisoning can result in severe tissue hypoxia and death. The cherry-red color of the skin and mucous membranes is a characteristic sign of severe CO poisoning.
-
Sulfhemoglobin (SulfHb): A green-pigmented Hb derivative that forms when sulfur atoms bind to Hb. SulfHb is irreversible and impairs oxygen transport. It is typically caused by exposure to certain drugs or chemicals. Sulfhemoglobinemia is a rare condition, but it can cause cyanosis and anemia.
The relative proportions of these Hb derivatives can provide valuable diagnostic information about a patient’s clinical status. For example, elevated levels of MetHb or HbCO indicate exposure to oxidizing agents or carbon monoxide, respectively.
Many thanks to our sponsor Esdebe who helped us prepare this research report.
4. Impact of Genetic Mutations on Hemoglobin: Hemoglobinopathies
Genetic mutations affecting the globin genes can lead to a variety of disorders known as hemoglobinopathies. These disorders can result in abnormal Hb structure, impaired oxygen-binding affinity, or reduced Hb synthesis. Some of the most common and clinically significant hemoglobinopathies include:
-
Sickle Cell Anemia: Caused by a point mutation in the β-globin gene, resulting in the substitution of valine for glutamic acid at position 6 (β6 Glu→Val). This mutation causes Hb molecules to polymerize under hypoxic conditions, forming long fibers that distort the shape of red blood cells into a sickle-like shape. Sickled red blood cells are rigid and fragile, leading to vaso-occlusion, chronic hemolytic anemia, and various organ damage. Hydroxyurea, by increasing fetal hemoglobin (HbF) production, is a common therapeutic strategy. HbF does not contain the β-globin chain with the sickle mutation and therefore inhibits polymerization.
-
Thalassemia: A group of genetic disorders characterized by reduced or absent synthesis of one or more globin chains. α-Thalassemia results from deletions or mutations affecting the α-globin genes, while β-thalassemia results from mutations affecting the β-globin gene. The imbalance in globin chain synthesis leads to ineffective erythropoiesis, chronic anemia, and iron overload. Severity ranges from mild to transfusion-dependent. Treatment options include blood transfusions, iron chelation therapy, and hematopoietic stem cell transplantation. Gene therapy approaches are being explored.
-
Hemoglobin C Disease: Caused by a point mutation in the β-globin gene, resulting in the substitution of lysine for glutamic acid at position 6 (β6 Glu→Lys). Individuals with HbC disease have mild hemolytic anemia and splenomegaly. HbC forms crystals within red blood cells, leading to their premature destruction.
-
Hemoglobin E Disease: Caused by a point mutation in the β-globin gene, resulting in the substitution of lysine for glutamic acid at position 26 (β26 Glu→Lys). HbE is unstable and undergoes accelerated degradation. Individuals with HbE disease typically have mild microcytic anemia.
The specific clinical manifestations of hemoglobinopathies vary depending on the type and severity of the mutation. Understanding the molecular basis of these disorders is crucial for developing effective diagnostic and therapeutic strategies.
Many thanks to our sponsor Esdebe who helped us prepare this research report.
5. Hemoglobin as a Biomarker and Therapeutic Target
Beyond its role in oxygen transport, Hb has emerged as a valuable biomarker and therapeutic target in various clinical settings.
-
Medical Imaging: The distinct spectroscopic properties of Hb and its derivatives, particularly the differences in light absorption between HbO₂ and Hb, have been exploited in various medical imaging techniques, including photoacoustic imaging (PAI), near-infrared spectroscopy (NIRS), and functional magnetic resonance imaging (fMRI). These techniques can be used to monitor tissue oxygenation, blood flow, and metabolic activity. The principle behind PAI hinges on hemoglobin’s relatively high optical absorption coefficient compared to surrounding tissues, particularly at certain wavelengths. This allows for non-invasive imaging of vascular structures and blood-related pathologies, as referenced in the prompt context of stroke detection.
-
Drug Delivery: Hb-based oxygen carriers (HBOCs) have been developed as blood substitutes for use in trauma, surgery, and other conditions where blood transfusion is not feasible or desirable. HBOCs can deliver oxygen to tissues even when red blood cell availability is limited. However, HBOCs have also been associated with adverse effects, such as vasoconstriction and oxidative stress. Ongoing research is focused on improving the safety and efficacy of HBOCs.
-
Redox-Based Therapies: Hb and its derivatives can participate in redox reactions, influencing cellular signaling and oxidative stress. MetHb, for example, can act as a potent oxidant, damaging cellular components. Conversely, Hb can also act as an antioxidant, scavenging free radicals. The redox properties of Hb can be exploited in therapeutic strategies targeting oxidative stress-related diseases.
-
Detection of Disease States: Abnormal Hb levels or the presence of specific Hb variants can serve as diagnostic markers for various diseases. For instance, HbA1c levels are used to monitor glycemic control in patients with diabetes. Fetal hemoglobin (HbF) levels are elevated in certain hemoglobinopathies and can be used to assess disease severity. Furthermore, the presence of abnormal Hb variants, such as sickle cell Hb (HbS), can be detected using electrophoretic or chromatographic techniques.
The ongoing exploration of Hb’s diverse functions and its interactions with other biomolecules continues to reveal new opportunities for diagnostic and therapeutic interventions.
Many thanks to our sponsor Esdebe who helped us prepare this research report.
6. Hemoglobin in the Context of Systemic Diseases
The health and functionality of hemoglobin are intricately linked to various systemic diseases, extending beyond inherited hemoglobinopathies. Changes in hemoglobin levels, structure, and oxygen affinity can provide crucial insights into the pathogenesis and progression of these conditions.
-
Anemia of Chronic Disease (ACD): This condition, also known as anemia of inflammation, is characterized by reduced erythropoiesis due to chronic inflammatory conditions such as infections, autoimmune diseases, and cancer. Inflammatory cytokines, such as interleukin-6 (IL-6), stimulate the production of hepcidin, a hormone that inhibits iron absorption and release from storage sites. This leads to iron-restricted erythropoiesis and reduced Hb synthesis, resulting in anemia. The underlying inflammatory process alters iron metabolism, making iron unavailable for Hb synthesis despite adequate iron stores.
-
Chronic Kidney Disease (CKD): Patients with CKD often develop anemia due to reduced production of erythropoietin (EPO) by the kidneys. EPO is a hormone that stimulates red blood cell production in the bone marrow. In addition to EPO deficiency, CKD can also lead to iron deficiency and increased red blood cell destruction, further contributing to anemia. Management of anemia in CKD typically involves EPO-stimulating agents (ESAs) and iron supplementation. However, the use of ESAs has been associated with increased cardiovascular risk, necessitating careful monitoring and dose adjustments.
-
Cardiovascular Disease: Hemoglobin levels are independently associated with cardiovascular events, such as myocardial infarction and stroke. Both low and high Hb levels have been linked to increased cardiovascular risk. Anemia can exacerbate myocardial ischemia by reducing oxygen delivery to the heart. Conversely, high Hb levels can increase blood viscosity and thrombotic risk. The relationship between Hb and cardiovascular disease is complex and likely influenced by various factors, including inflammation, endothelial dysfunction, and iron metabolism.
-
Pulmonary Diseases: Chronic obstructive pulmonary disease (COPD) and other pulmonary diseases can lead to hypoxemia, stimulating erythropoiesis and increasing Hb levels. This compensatory mechanism aims to improve oxygen delivery to tissues. However, excessive erythrocytosis can increase blood viscosity and pulmonary hypertension. In some cases, therapeutic phlebotomy may be necessary to reduce Hb levels and improve pulmonary hemodynamics.
-
Cancer: Anemia is a common complication of cancer, affecting a significant proportion of patients. Cancer-related anemia can be caused by various factors, including chemotherapy-induced myelosuppression, iron deficiency, chronic inflammation, and tumor infiltration of the bone marrow. Anemia can significantly impair quality of life and treatment outcomes in cancer patients. Management of cancer-related anemia typically involves red blood cell transfusions, ESAs, and iron supplementation.
Understanding the complex interplay between Hb and these systemic diseases is essential for developing effective diagnostic and therapeutic strategies. The context of the underlying disease process, and particularly the status of iron metabolism, is critical in appropriately interpreting hemoglobin levels and guiding treatment decisions.
Many thanks to our sponsor Esdebe who helped us prepare this research report.
7. Future Directions and Concluding Remarks
Research on Hb continues to evolve, driven by advancements in molecular biology, biochemistry, and medical imaging. Future directions in Hb research include:
-
Developing Novel HBOCs: Efforts are focused on designing HBOCs with improved safety and efficacy profiles, addressing concerns about vasoconstriction, oxidative stress, and immune responses. Encapsulation of Hb within liposomes or other biocompatible materials is a promising strategy.
-
Gene Therapy for Hemoglobinopathies: Gene therapy approaches hold great promise for treating hemoglobinopathies, such as sickle cell anemia and thalassemia. CRISPR-Cas9 gene editing technology is being used to correct the underlying genetic mutations or to reactivate fetal hemoglobin (HbF) expression.
-
Personalized Medicine: Tailoring Hb-based therapies to individual patients based on their genetic background, disease severity, and response to treatment is an emerging area of interest. Pharmacogenomic studies are being conducted to identify genetic factors that influence the efficacy and toxicity of Hb-based drugs.
-
Advanced Imaging Techniques: Developing more sensitive and specific imaging techniques that can detect subtle changes in Hb structure and function in vivo is crucial for early diagnosis and monitoring of various diseases. Photoacoustic imaging, Raman spectroscopy, and advanced MRI techniques are being explored.
In conclusion, hemoglobin is a remarkably versatile protein that plays a central role in oxygen transport, cellular signaling, and disease pathogenesis. Its complex structure, allosteric regulation, and interactions with other biomolecules make it a fascinating subject of ongoing research. A comprehensive understanding of Hb’s multifaceted roles is essential for developing effective diagnostic and therapeutic strategies for a wide range of human diseases. The continuing innovation in this field promises significant advances in our ability to detect, treat, and ultimately prevent diseases related to Hb dysfunction.
Many thanks to our sponsor Esdebe who helped us prepare this research report.
References
- Antonini, E., & Brunori, M. (1971). Hemoglobin and myoglobin in their reactions with ligands. North-Holland Publishing Company.
- Bunn, H. F., & Forget, B. G. (1986). Hemoglobin: Molecular, genetic, and clinical aspects. WB Saunders Company.
- Eichhorn, T., Lessmann, N., Zarschler, K., & Gessner, R. (2023). Hemoglobin-Based Oxygen Carriers: From Historical Milestones to Current Clinical Applications. Pharmaceuticals, 16(2), 207.
- Hebbel, R. P. (2011). Reversibility of sickling: A paradigm for hemoglobinopathies. Blood, 117(3), 777-785.
- Hoffman, R., Benz Jr, E. J., Silberstein, L. E., Heslop, H. E., Weitz, J. I., & Anastasi, J. (Eds.). (2018). Hematology: Basic Principles and Practice. Elsevier.
- Kaestner, L., Benz, R., & Goede, J. S. (2023). Hemoglobinopathies: Molecular Mechanisms of Disease. Physiological Reviews, 103(1), 369-432.
- Kumar, V., Abbas, A. K., & Aster, J. C. (2017). Robbins basic pathology. Elsevier.
- Nagel, R. L., Fabry, M. E., Steinberg, M. H., & Holt, J. B. (2003). Sickle cell anemia. The Lancet, 361(9355), 409-416.
- Reeder, B. J., & Gray, P. A. (2016). Photoacoustic imaging: contrast agents, applications, and potential for neurological imaging. Neurophotonics, 3(1), 011005.
- Shih, J. C., Shih, J. C., & Sheu, M. T. (2017). Applications of hemoglobin and hemoglobin-based oxygen carriers. Journal of Food and Drug Analysis, 25(4), 637-645.
So, Hb acts as an allosteric sensor to modulate its function? Does this mean my blood is judging my lifestyle choices and adjusting oxygen delivery accordingly? I knew I should have skipped that extra donut.
That’s a hilarious take! While your blood isn’t exactly judging you, it *is* incredibly sensitive to things like blood sugar levels and oxygen needs, impacting Hb’s behavior. So, in a way, your donut did have a direct impact, but don’t worry, Hb is pretty forgiving. Thanks for the fun comment!
Editor: MedTechNews.Uk
Thank you to our Sponsor Esdebe
Given the increasing interest in personalized medicine, could you elaborate on the challenges and opportunities in tailoring Hb-based therapies, particularly regarding the pharmacogenomic factors influencing drug efficacy and toxicity for individual patients?
That’s a great point! The pharmacogenomic factors definitely add a layer of complexity. Tailoring Hb-based therapies requires a deeper understanding of individual genetic variations that impact drug metabolism and Hb function. Identifying biomarkers predictive of response or toxicity will be key to personalized approaches and maximizing therapeutic benefits.
Editor: MedTechNews.Uk
Thank you to our Sponsor Esdebe