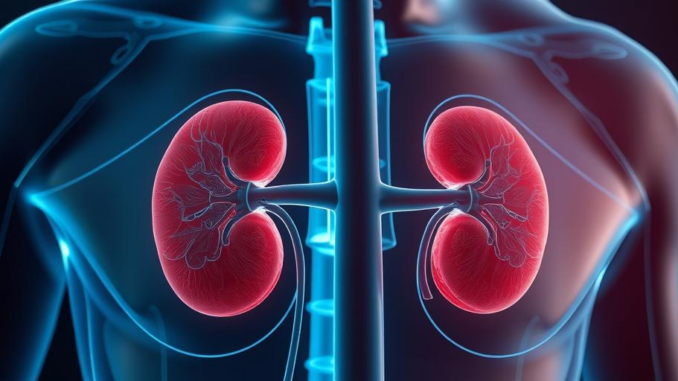
Abstract
Intrarenal pressure (IRP) is a critical physiological parameter influencing kidney function. Disruptions in IRP homeostasis, stemming from factors such as elevated fluid viscosity, outflow obstruction, or external compression, can initiate a cascade of detrimental effects, culminating in renal dysfunction and potential irreversible damage. This research report provides a comprehensive overview of IRP dynamics, encompassing its regulation, the underlying pathophysiological mechanisms leading to pressure dysregulation, the resultant physiological consequences, and current clinical strategies for monitoring and managing IRP, particularly in the context of urological interventions. Furthermore, it delves into the long-term effects of sustained IRP elevation on renal health, highlighting knowledge gaps and potential avenues for future research aimed at improving diagnostic and therapeutic approaches for pressure-related kidney pathologies. The review critically assesses the limitations of current clinical practices and emphasizes the necessity for developing more sophisticated, non-invasive IRP monitoring techniques and targeted interventions to mitigate the adverse effects of abnormal renal pressure.
Many thanks to our sponsor Esdebe who helped us prepare this research report.
1. Introduction
The kidney, a highly vascularized and metabolically active organ, maintains fluid and electrolyte balance, regulates blood pressure, and eliminates waste products. Optimal renal function is critically dependent on the intricate interplay of hemodynamic forces and intrarenal pressure (IRP). IRP, representing the pressure within the renal parenchyma, collecting system, and vasculature, dictates the efficiency of glomerular filtration, tubular reabsorption, and overall renal perfusion. Dysregulation of IRP, characterized by either significant elevation or reduction, can have profound consequences, ranging from acute kidney injury (AKI) to chronic kidney disease (CKD). While elevated IRP is the primary focus of this review due to its more frequently implicated role in renal pathology, the importance of maintaining adequate IRP for proper renal function should not be understated.
This report will explore the multifaceted aspects of IRP, starting with a detailed examination of the physiological mechanisms governing its regulation. We will then delve into the various factors that contribute to increased IRP, including, but not limited to, increased fluid viscosity (as exemplified by the scenario mentioned in the prompt), outflow obstruction (such as ureteral stones or strictures), and external compression. Subsequently, the physiological effects of elevated IRP on renal function, cellular integrity, and the development of long-term kidney damage will be discussed. Finally, we will review current methods for monitoring and managing IRP during urological procedures and explore potential future directions for improving patient outcomes.
Many thanks to our sponsor Esdebe who helped us prepare this research report.
2. Physiological Regulation of Intrarenal Pressure
IRP is not a static entity but rather a dynamic variable tightly regulated by a complex interplay of intrinsic and extrinsic mechanisms. These mechanisms operate to maintain IRP within a narrow physiological range, ensuring optimal renal perfusion and function.
2.1. Intrinsic Mechanisms
-
Autoregulation of Renal Blood Flow: The kidney possesses a remarkable capacity to maintain a relatively constant renal blood flow (RBF) and glomerular filtration rate (GFR) despite fluctuations in systemic blood pressure. This autoregulation is primarily mediated by two mechanisms: myogenic response and tubuloglomerular feedback (TGF).
- Myogenic Response: This intrinsic mechanism involves the constriction or dilation of afferent arterioles in response to changes in intraluminal pressure. Increased pressure causes afferent arteriolar constriction, reducing RBF and preventing excessive glomerular pressure. Conversely, decreased pressure leads to afferent arteriolar dilation, maintaining RBF and GFR.
- Tubuloglomerular Feedback (TGF): TGF is a negative feedback loop that links changes in tubular fluid composition in the distal tubule to afferent arteriolar tone. The macula densa, a specialized group of cells in the distal tubule, senses changes in NaCl concentration in the tubular fluid. Increased NaCl concentration signals a reduced GFR and RBF, triggering the release of vasoactive substances (e.g., adenosine) that cause afferent arteriolar constriction, reducing RBF and GFR back to normal. Conversely, decreased NaCl concentration leads to afferent arteriolar dilation.
-
Intrarenal Angiotensin II (Ang II) System: The kidney possesses its own local Ang II system, independent of the systemic renin-angiotensin-aldosterone system (RAAS). Ang II, produced locally in the kidney, exerts potent vasoconstrictor effects on both afferent and efferent arterioles. However, its effect is more pronounced on efferent arterioles, leading to an increase in glomerular pressure and GFR. The intrarenal Ang II system plays a crucial role in maintaining glomerular filtration, especially in situations of reduced RBF or systemic hypotension.
2.2. Extrinsic Mechanisms
-
Sympathetic Nervous System: The sympathetic nervous system exerts significant influence on renal hemodynamics and IRP. Sympathetic activation causes renal vasoconstriction, primarily via α1-adrenergic receptors on renal blood vessels. This leads to a decrease in RBF and GFR, and consequently, a reduction in IRP. Furthermore, sympathetic activation stimulates renin release from the juxtaglomerular cells, leading to increased Ang II production and further vasoconstriction.
-
Hormonal Regulation: Several hormones, including atrial natriuretic peptide (ANP), endothelin-1 (ET-1), and nitric oxide (NO), influence renal hemodynamics and IRP. ANP, released from the heart in response to atrial stretch, promotes vasodilation and natriuresis, leading to a decrease in blood volume and blood pressure. ET-1, produced by endothelial cells, is a potent vasoconstrictor that increases renal vascular resistance and IRP. NO, also produced by endothelial cells, promotes vasodilation and protects against vasoconstriction, contributing to the maintenance of RBF and GFR.
Many thanks to our sponsor Esdebe who helped us prepare this research report.
3. Factors Contributing to Increased Intrarenal Pressure
Elevated IRP can arise from a variety of factors that disrupt the delicate balance of fluid dynamics within the kidney. These factors can be broadly categorized as those affecting afferent flow, intrarenal obstruction, outflow obstruction, and external compression.
3.1. Increased Afferent Flow or Pressure
While less commonly a direct cause of sustained elevated IRP, conditions that acutely increase afferent arteriolar pressure without adequate compensatory mechanisms can transiently increase IRP. This might occur, for example, with rapid infusion of large volumes of intravenous fluids, particularly in individuals with pre-existing renal dysfunction.
3.2. Increased Fluid Viscosity
As alluded to in the prompt, increased viscosity of the tubular fluid can significantly elevate IRP. The resistance to flow increases linearly with viscosity, thus even moderate changes in fluid viscosity can greatly increase the required pressure gradient to maintain flow. This is especially true when combined with other factors, such as mild obstruction. Examples include:
-
Contrast-Induced Nephropathy (CIN): The administration of iodinated contrast media during radiological procedures can increase the viscosity of tubular fluid, particularly in patients with pre-existing renal impairment or dehydration. This increased viscosity, coupled with potential direct toxic effects of the contrast agent on tubular cells, can contribute to AKI.
-
Hyperuricemia/Uric Acid Nephropathy: High levels of uric acid in the tubular fluid can lead to the precipitation of uric acid crystals, increasing fluid viscosity and causing tubular obstruction and inflammation.
-
Myeloma Cast Nephropathy: In patients with multiple myeloma, the overproduction of monoclonal light chains can lead to the formation of proteinaceous casts in the distal tubules, increasing fluid viscosity and obstructing tubular flow.
3.3. Intrarenal Obstruction
Obstruction within the renal parenchyma, such as tubular obstruction or interstitial inflammation, can impede fluid flow and elevate IRP.
-
Acute Tubular Necrosis (ATN): ATN, caused by ischemia or nephrotoxins, leads to tubular cell damage and sloughing, resulting in tubular obstruction and increased IRP.
-
Interstitial Nephritis: Inflammation of the renal interstitium, caused by infections, drugs, or autoimmune disorders, can lead to edema and compression of renal tubules, increasing IRP.
3.4. Outflow Obstruction
Obstruction of the urinary outflow tract, at any level from the renal pelvis to the urethra, is a common cause of elevated IRP.
-
Ureteral Obstruction: Ureteral stones, strictures, tumors, or external compression can obstruct urine flow from the kidney to the bladder, leading to hydronephrosis and increased IRP. The degree of IRP elevation depends on the severity and duration of the obstruction. Acute, complete ureteral obstruction can lead to rapid and significant increases in IRP, potentially causing irreversible renal damage. Chronic, partial obstruction may result in a more gradual increase in IRP, with compensatory mechanisms initially mitigating the effects.
-
Bladder Outlet Obstruction (BOO): BOO, caused by prostatic enlargement, urethral strictures, or neurogenic bladder dysfunction, can impede urine flow from the bladder, leading to increased intravesical pressure and backpressure on the kidneys, resulting in hydronephrosis and elevated IRP. It is worth noting that chronic backpressure will, over time, damage the renal pelvis and ureter as well. This can lead to a loss of peristalsis and can hinder the kidney’s ability to remove fluid, even after the original obstruction is resolved.
3.5. External Compression
External compression of the kidney can also lead to increased IRP.
-
Abdominal Compartment Syndrome (ACS): ACS, characterized by increased intra-abdominal pressure (IAP), can compress the kidneys and renal vasculature, leading to decreased RBF and GFR, and increased IRP. ACS can occur in critically ill patients with trauma, burns, or pancreatitis.
-
Retroperitoneal Fibrosis: Retroperitoneal fibrosis, a rare condition characterized by the formation of fibrous tissue in the retroperitoneum, can encase and compress the ureters, leading to hydronephrosis and increased IRP.
Many thanks to our sponsor Esdebe who helped us prepare this research report.
4. Physiological Effects of Elevated Intrarenal Pressure
Elevated IRP exerts a multitude of adverse effects on renal function and structure, contributing to both acute and chronic kidney diseases. The severity and nature of these effects depend on the magnitude and duration of IRP elevation.
4.1. Effects on Renal Hemodynamics
Elevated IRP directly impacts renal hemodynamics by compressing renal blood vessels, increasing renal vascular resistance, and reducing RBF. This reduction in RBF leads to decreased GFR and impaired renal function. The autoregulatory mechanisms of the kidney may initially compensate for the increased IRP by dilating afferent arterioles, but these mechanisms are eventually overwhelmed by sustained pressure elevation.
4.2. Effects on Tubular Function
Increased IRP can disrupt tubular function by compressing renal tubules, impairing tubular reabsorption and secretion. This can lead to electrolyte imbalances, acid-base disturbances, and impaired concentration ability.
4.3. Effects on Renal Oxygenation
Elevated IRP reduces RBF and can lead to renal ischemia and hypoxia. Hypoxia can damage tubular cells, contributing to ATN and further exacerbating renal dysfunction. The outer medulla, which is already relatively hypoxic due to its high metabolic demand and relatively low blood flow, is particularly vulnerable to the effects of increased IRP.
4.4. Effects on Renal Inflammation and Fibrosis
Chronic elevation of IRP can trigger inflammatory pathways and promote renal fibrosis, leading to irreversible renal damage. Increased IRP can activate inflammatory cells, such as macrophages, and stimulate the production of pro-inflammatory cytokines and growth factors, such as transforming growth factor-β (TGF-β). TGF-β promotes the deposition of extracellular matrix (ECM) proteins, leading to fibrosis and scarring of the renal parenchyma. This fibrosis disrupts the normal renal architecture and impairs renal function.
4.5. Effects on Cellular Integrity
Sustained increases in IRP can lead to cellular damage and apoptosis in various renal cell types, including tubular cells, glomerular cells, and interstitial cells. The mechanical stress imposed by elevated IRP can activate intracellular signaling pathways that promote cell death. Furthermore, hypoxia induced by increased IRP can also contribute to cellular damage and apoptosis.
Many thanks to our sponsor Esdebe who helped us prepare this research report.
5. Monitoring and Management of Intrarenal Pressure
The ability to monitor and manage IRP is crucial for preventing and mitigating the adverse effects of elevated renal pressure, particularly during urological procedures. However, direct measurement of IRP is challenging and not routinely performed in clinical practice.
5.1. Current Monitoring Techniques
-
Invasive Measurement: The gold standard for measuring IRP is direct cannulation of the renal pelvis or ureter with a pressure transducer. However, this method is invasive and carries the risk of complications, such as infection, bleeding, and ureteral injury. Therefore, it is typically reserved for research purposes or specific clinical scenarios where accurate IRP measurement is essential.
-
Indirect Assessment: In clinical practice, IRP is typically assessed indirectly through imaging techniques and functional markers.
- Ultrasound: Ultrasound can detect hydronephrosis, a sign of increased pressure in the renal collecting system. However, ultrasound is not quantitative and cannot directly measure IRP.
- Computed Tomography (CT) and Magnetic Resonance Imaging (MRI): CT and MRI can provide detailed anatomical information about the kidney and urinary tract, including the degree of hydronephrosis and the presence of any obstruction. However, like ultrasound, these techniques do not directly measure IRP.
- Renal Scintigraphy: Renal scintigraphy can assess renal function and drainage. Delayed drainage can suggest increased pressure in the renal collecting system.
- Serum Creatinine and Estimated Glomerular Filtration Rate (eGFR): Serum creatinine and eGFR are markers of overall renal function. A decrease in eGFR can indicate renal injury caused by elevated IRP. However, these markers are not specific for IRP and can be affected by other factors.
5.2. Management Strategies
Management strategies for elevated IRP focus on addressing the underlying cause and reducing the pressure within the kidney.
-
Relieving Obstruction: If elevated IRP is caused by urinary outflow obstruction, the primary goal is to relieve the obstruction. This may involve the placement of a ureteral stent, nephrostomy tube, or surgical intervention.
-
Fluid Management: In cases of increased fluid viscosity or volume overload, fluid management strategies, such as intravenous fluids or diuretics, may be employed to reduce fluid volume and pressure.
-
Medical Management: Depending on the underlying cause of increased IRP, medical management may include antibiotics for infections, immunosuppressants for inflammatory conditions, or medications to lower uric acid levels.
-
Minimally Invasive Urological Procedures: Many urological procedures, such as ureteroscopy and percutaneous nephrolithotomy (PCNL), can be performed minimally invasively to relieve obstruction and reduce IRP. However, these procedures can also increase IRP if the irrigation pressure is too high. Therefore, it is important to monitor IRP during these procedures and maintain the lowest possible irrigation pressure.
5.3 Future Directions in IRP Monitoring and Management
Several avenues for future research and development hold promise for improving IRP monitoring and management.
-
Non-Invasive IRP Monitoring: The development of non-invasive IRP monitoring techniques would revolutionize the management of patients at risk for elevated renal pressure. Potential approaches include the use of novel imaging techniques, such as ultrasound elastography or MRI-based pressure mapping, or the development of wearable sensors that can detect changes in renal blood flow or oxygenation.
-
Targeted Therapies: The development of targeted therapies that can specifically reduce IRP without affecting systemic blood pressure would be a significant advance. Potential targets include intrarenal Ang II system, endothelin-1 pathway, and inflammatory pathways.
-
Personalized Management Strategies: Tailoring IRP management strategies to the individual patient based on their underlying condition, renal function, and risk factors would optimize outcomes and minimize complications. This requires further research into the pathophysiology of IRP dysregulation and the development of predictive models that can identify patients at high risk for developing elevated IRP.
Many thanks to our sponsor Esdebe who helped us prepare this research report.
6. Long-Term Damage Caused by Pressure Increases
Chronic elevation of IRP, even if seemingly mild, can lead to significant long-term damage to the kidney. The duration and magnitude of pressure elevation are critical determinants of the extent of injury. The progressive nature of the damage often goes unnoticed until significant renal dysfunction is present.
-
Chronic Kidney Disease (CKD): The most significant long-term consequence of chronic IRP elevation is the development of CKD. The persistent inflammation, fibrosis, and cellular damage caused by elevated IRP lead to a progressive decline in renal function.
-
Hypertension: CKD resulting from increased IRP can exacerbate or even initiate hypertension. The damaged kidneys are less able to regulate sodium and water balance, contributing to increased blood volume and blood pressure. Additionally, the kidneys may inappropriately activate the renin-angiotensin-aldosterone system (RAAS), further contributing to hypertension.
-
End-Stage Renal Disease (ESRD): In severe cases, chronic IRP elevation can lead to ESRD, requiring dialysis or kidney transplantation for survival. ESRD is associated with significant morbidity and mortality, highlighting the importance of preventing and managing elevated IRP to preserve renal function.
-
Increased Cardiovascular Risk: CKD and ESRD are both strong independent risk factors for cardiovascular disease. The chronic inflammation, oxidative stress, and electrolyte imbalances associated with kidney disease contribute to accelerated atherosclerosis and increased risk of heart attack, stroke, and heart failure.
Many thanks to our sponsor Esdebe who helped us prepare this research report.
7. Conclusion
Intrarenal pressure dynamics play a vital role in maintaining renal function. Disruptions in IRP homeostasis, primarily elevation, can lead to a cascade of detrimental effects, culminating in renal dysfunction and irreversible damage. A multitude of factors, including increased fluid viscosity, outflow obstruction, and external compression, can contribute to elevated IRP. Monitoring and managing IRP, particularly during urological procedures, is crucial for preventing and mitigating these adverse effects. Current monitoring techniques are limited, highlighting the need for developing more sophisticated, non-invasive methods. Management strategies focus on addressing the underlying cause and reducing the pressure within the kidney. Further research is needed to improve our understanding of the pathophysiology of IRP dysregulation and to develop targeted therapies and personalized management strategies to optimize patient outcomes. The long-term consequences of chronic IRP elevation, including CKD, hypertension, and increased cardiovascular risk, underscore the importance of preventing and managing this condition to preserve renal function and overall health.
Many thanks to our sponsor Esdebe who helped us prepare this research report.
References
- Rennke HG, Denker BM. Renal pathophysiology: essential concepts. Lippincott Williams & Wilkins; 2007.
- Rose BD, Post TW. Clinical physiology of acid-base and electrolyte disorders. McGraw-Hill Professional; 2001.
- Levinsky NG. Pathophysiology of acute renal failure. N Engl J Med. 1977;296(26):1453-1458.
- Klahr S. Obstructive nephropathy. Kidney Int. 1983;23(4):71-84.
- Chevalier RL. Obstructive nephropathy: from experimental models to clinical relevance. Kidney Int. 2006;69(8):1327-1338.
- Okegawa T, Pong RC, Nutile R, et al. Effect of intrarenal pelvic pressure on renal function. J Urol. 1999;161(4):1315-1318.
- Wilcox CS. New insights into the role of the kidney in hypertension. Am J Kidney Dis. 2005;45(6):1095-1111.
- Lameire N, Van Biesen W, Vanholder R. Acute kidney injury. Lancet. 2005;365(9457):417-430.
- Andreucci M, Faga A, Sabbatini M, et al. Pathophysiology of crystal-induced acute kidney injury. Kidney Int. 2016;90(2):225-236.
- Kellum JA, Lameire N, Aspelin P, et al. Kidney Disease: Improving Global Outcomes (KDIGO) acute kidney injury work group. KDIGO clinical practice guideline for acute kidney injury. Kidney Int Suppl. 2012;2(1):1-138.
- Harris RC, Neilson EG. Mechanism of tubulointerstitial fibrosis. Kidney Int. 1991;39(5):1051-1061.
- Woolf AS, Winyard PJ, Rosser EM, et al. Congenital obstructive nephropathy: cellular and molecular consequences. Kidney Int. 2000;57(4):1245-1255.
- Erlichman J, Pettit JW, Klahr S. Effect of increased intrapelvic pressure on proximal tubular reabsorption in the rat. Am J Physiol. 1988;254(3 Pt 2):F362-F367.
- Leger KJ, Loiselle TD, Chee KG, et al. Intrarenal pressure during flexible ureteroscopy: a randomized prospective study. J Urol. 2013;189(5):1783-1787.
- Bader MJ, Eisner B, Porpiglia F, et al. Contemporary management of ureteric stones. Eur Urol. 2012;61(4):642-652.
- Ganpule AP, Bhattu AS, Desai MR. Management of renal stones – Recent advances. Indian J Urol. 2012;28(1):2-9.
- Taylor AL, Muto G, Pearle MS. Contemporary techniques for upper tract imaging: ultrasound, computed tomography, and magnetic resonance imaging. Urol Clin North Am. 2007;34(3):319-333.
- Hoenig DM, Shalhav AL, Elbahnasy AM, et al. Lower pole percutaneous nephrolithotomy: comparison of prone and supine approaches. J Endourol. 2004;18(4):305-309.
- Manohar T, Autorino R, Hameed BMZ, et al. Clinical impact of intrarenal pressure during flexible ureteroscopy: systematic review. J Endourol. 2021;35(11):1551-1565.
- Desai M, Kukreja R, Desai M. The renal access: getting it right. J Endourol. 2010;24(11):1729-1733.
Be the first to comment