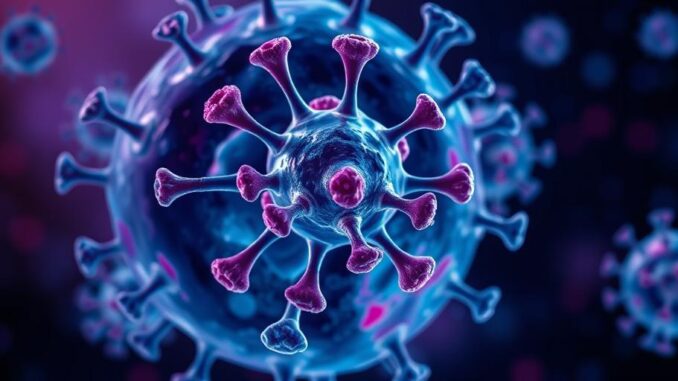
The Multifaceted Role of TYK2 in Immunity, Autoimmunity, and Beyond: From Molecular Mechanisms to Therapeutic Interventions
Many thanks to our sponsor Esdebe who helped us prepare this research report.
Abstract
Tyrosine kinase 2 (TYK2), a member of the Janus kinase (JAK) family, has emerged as a critical mediator of cytokine signaling and immune responses. While initially recognized for its role in type I interferon (IFN) and interleukin (IL)-12/IL-23 signaling, its involvement in a broader range of immune pathways and autoimmune diseases is now increasingly appreciated. This review delves into the multifaceted nature of TYK2, exploring its structural features, functional domains, and intricate signaling networks. We examine its specific roles in driving inflammation and the pathogenesis of autoimmune conditions such as psoriasis, systemic lupus erythematosus (SLE), inflammatory bowel disease (IBD), and type 1 diabetes (T1D), highlighting its non-redundant functions. Furthermore, we discuss the development and clinical application of TYK2 inhibitors, focusing on their efficacy, safety profiles, and potential advantages over broader JAK inhibitors. We also critically analyze the potential benefits and risks associated with TYK2 targeting in diverse patient populations and propose future research directions to fully unlock the therapeutic potential of TYK2 inhibition while minimizing off-target effects.
Many thanks to our sponsor Esdebe who helped us prepare this research report.
1. Introduction
The Janus kinase (JAK) family, comprising JAK1, JAK2, JAK3, and TYK2, plays a central role in intracellular signaling downstream of a diverse array of cytokine receptors. These kinases, characterized by their unique dual kinase-like domains, orchestrate cellular responses to interferons, interleukins, and growth factors, thereby influencing hematopoiesis, immune cell development, and inflammatory processes [1]. Unlike the other JAK family members, TYK2 exhibits a more restricted expression pattern and signaling profile, primarily associated with type I interferon (IFN-α/β), IL-12, IL-23, and IL-6 family cytokine pathways [2].
The crucial role of TYK2 in immunity has been underscored by genetic studies in humans and mice. Loss-of-function mutations in TYK2 have been linked to impaired IFN-α/β responses and increased susceptibility to certain infections, including viral and mycobacterial pathogens [3]. Conversely, gain-of-function variants or aberrant activation of TYK2 contribute to the pathogenesis of various autoimmune diseases, including psoriasis, psoriatic arthritis, systemic lupus erythematosus (SLE), inflammatory bowel disease (IBD), and type 1 diabetes (T1D) [4]. These observations have fueled intense interest in targeting TYK2 as a therapeutic strategy for autoimmune disorders. The selective inhibition of TYK2 offers the promise of modulating specific cytokine pathways involved in disease pathogenesis, while potentially sparing the broader effects associated with pan-JAK inhibition, such as hematologic abnormalities and increased risk of infections [5].
This review provides a comprehensive overview of TYK2, encompassing its molecular structure, signaling mechanisms, and roles in immune responses and autoimmune diseases. It also discusses the development of TYK2 inhibitors and their potential clinical applications, highlighting both the opportunities and challenges associated with targeting this crucial kinase.
Many thanks to our sponsor Esdebe who helped us prepare this research report.
2. Structure and Function of TYK2
2.1. Molecular Architecture
TYK2 is a non-receptor tyrosine kinase with a molecular weight of approximately 135 kDa. Like other JAK family members, TYK2 possesses a characteristic domain structure, including an N-terminal FERM domain, an SH2-like domain, a pseudokinase domain (JH2), and a kinase domain (JH1) [6].
- FERM domain: This domain mediates interactions with cytokine receptors and other signaling proteins, playing a critical role in the localization of TYK2 to the receptor complex [7]. The FERM domain consists of four subdomains: F1, F2, F3, and F4. These subdomains facilitate interactions with membrane proximal regions of cytokine receptors. Mutations in the FERM domain can disrupt receptor binding and impair downstream signaling.
- SH2-like domain: Although structurally similar to SH2 domains, the SH2-like domain of TYK2 lacks the canonical phosphotyrosine-binding pocket. Instead, it contributes to protein-protein interactions and regulates kinase activity [8]. It is thought that this domain is important for stabilizing the overall structure of the kinase and facilitating interactions with other signaling molecules.
- Pseudokinase domain (JH2): This domain is catalytically inactive due to key amino acid substitutions in the ATP-binding site. Initially considered a regulatory domain, recent studies suggest that JH2 plays a more active role in modulating the conformation and activity of the kinase domain [9]. The JH2 domain allosterically regulates the activity of the JH1 domain, maintaining it in an inactive state in the absence of cytokine stimulation. Upon cytokine binding, the JH2 domain undergoes conformational changes that relieve this inhibition, allowing the JH1 domain to become activated.
- Kinase domain (JH1): This domain is the catalytic domain of TYK2, responsible for phosphorylating tyrosine residues on downstream substrates. The JH1 domain contains the conserved ATP-binding site and activation loop, which are essential for kinase activity [10]. Phosphorylation of the activation loop is crucial for the full activation of TYK2. The ATP-binding pocket is the target for many of the currently developed TYK2 inhibitors.
2.2. Activation and Signaling Pathways
TYK2 activation is initiated by the binding of cytokines to their cognate receptors. This triggers receptor oligomerization and the recruitment of JAKs, including TYK2, to the receptor complex. In the case of type I IFN receptors, TYK2 associates with IFNAR1, while JAK1 associates with IFNAR2 [11]. Similarly, IL-12 and IL-23 receptors recruit TYK2 and JAK2 [12]. Receptor engagement promotes the transphosphorylation of JAKs, leading to their activation. Activated TYK2 phosphorylates tyrosine residues on the cytoplasmic tails of the cytokine receptors, creating docking sites for STAT (signal transducer and activator of transcription) proteins. STATs are then recruited to the receptor complex, where they are phosphorylated by JAKs, including TYK2. Phosphorylated STATs dimerize and translocate to the nucleus, where they bind to DNA and regulate the transcription of target genes [13].
The specific STATs activated by TYK2 depend on the cytokine receptor involved. Type I IFN signaling primarily activates STAT1 and STAT2, leading to the induction of interferon-stimulated genes (ISGs) [14]. IL-12 signaling activates STAT4, promoting the differentiation of T helper 1 (Th1) cells [15]. IL-23 signaling activates STAT3, which is important for the differentiation of Th17 cells and the production of IL-17 [16]. While these are the primary STATs activated, some redundancy and cross-talk exist, and other STATs can be activated to a lesser extent depending on the cellular context.
Beyond the classical JAK-STAT pathway, TYK2 can also activate other signaling pathways, including the MAPK (mitogen-activated protein kinase) and PI3K-AKT pathways. These pathways contribute to diverse cellular functions, such as cell proliferation, survival, and differentiation [17]. The involvement of these pathways highlights the complexity of TYK2 signaling and suggests that its effects extend beyond direct STAT activation.
Many thanks to our sponsor Esdebe who helped us prepare this research report.
3. Role of TYK2 in Immune Responses and Autoimmune Diseases
3.1. Innate Immunity
TYK2 plays a critical role in innate immune responses, particularly in the response to viral infections. It is essential for type I IFN signaling, which is a key component of the antiviral response [18]. Type I IFNs induce the expression of hundreds of ISGs, which mediate antiviral effects by inhibiting viral replication, promoting viral clearance, and activating immune cells. TYK2 deficiency results in impaired type I IFN signaling and increased susceptibility to viral infections [19].
TYK2 also contributes to innate immune responses through its involvement in IL-12 and IL-23 signaling. IL-12, produced by dendritic cells and macrophages, activates natural killer (NK) cells and promotes the differentiation of Th1 cells, which are important for clearing intracellular pathogens [20]. IL-23, also produced by dendritic cells and macrophages, stimulates the production of IL-17 by innate immune cells, such as γδ T cells and ILC3s, contributing to host defense against extracellular bacteria and fungi [21].
3.2. Adaptive Immunity
In adaptive immunity, TYK2 influences T cell differentiation and function. As mentioned earlier, TYK2 is required for IL-12-mediated differentiation of Th1 cells and IL-23-mediated differentiation of Th17 cells. Th1 cells produce IFN-γ, which activates macrophages and promotes cell-mediated immunity [22]. Th17 cells produce IL-17, which recruits neutrophils to sites of infection and promotes inflammation [23]. Dysregulation of Th1 and Th17 responses contributes to the pathogenesis of many autoimmune diseases.
TYK2 also plays a role in the regulation of T regulatory (Treg) cell function. Treg cells are a subset of T cells that suppress immune responses and maintain immune homeostasis [24]. Some studies have suggested that TYK2 signaling can impair Treg cell function, contributing to autoimmunity. However, the precise role of TYK2 in Treg cell biology remains controversial and requires further investigation. Some studies show that TYK2 is involved in the development and function of regulatory T cells, which are important for suppressing immune responses and maintaining immune homeostasis, therefore inhibition of TYK2 could potentially disrupt Treg cell function, leading to exacerbated autoimmune responses or other immune dysregulation. This is a critical point to consider when developing TYK2 inhibitors.
3.3. Autoimmune Diseases
The involvement of TYK2 in multiple immune pathways makes it a critical player in the pathogenesis of various autoimmune diseases. The following are some of the autoimmune conditions where TYK2 has been implicated:
- Psoriasis: Psoriasis is a chronic inflammatory skin disease characterized by hyperproliferation of keratinocytes and infiltration of immune cells into the skin. IL-23/IL-17 axis plays a central role in the pathogenesis of psoriasis, and TYK2 is essential for IL-23 signaling. Genetic studies have identified TYK2 as a major susceptibility gene for psoriasis [25].
- Psoriatic Arthritis: Psoriatic arthritis is an inflammatory arthritis associated with psoriasis. Similar to psoriasis, the IL-23/IL-17 axis is implicated in the pathogenesis of psoriatic arthritis. TYK2 inhibition has shown efficacy in treating both skin and joint manifestations of psoriatic arthritis [26].
- Systemic Lupus Erythematosus (SLE): SLE is a systemic autoimmune disease characterized by the production of autoantibodies and inflammation in multiple organs. Type I IFN signaling is thought to play a crucial role in the pathogenesis of SLE. TYK2, being essential for type I IFN signaling, has been implicated in SLE. Genetic studies have also linked TYK2 to SLE susceptibility [27].
- Inflammatory Bowel Disease (IBD): IBD, including Crohn’s disease and ulcerative colitis, is a chronic inflammatory condition of the gastrointestinal tract. Both IL-12 and IL-23 have been implicated in the pathogenesis of IBD. TYK2 contributes to the signaling of both cytokines and has been identified as a potential therapeutic target for IBD [28].
- Type 1 Diabetes (T1D): T1D is an autoimmune disease characterized by the destruction of insulin-producing beta cells in the pancreas. Type I IFN signaling has been implicated in the early stages of T1D development. TYK2 may contribute to the pathogenesis of T1D through its role in type I IFN signaling and potentially through other mechanisms [29].
Many thanks to our sponsor Esdebe who helped us prepare this research report.
4. TYK2 Inhibitors as Therapeutic Agents
4.1. Development of TYK2 Inhibitors
Given the critical role of TYK2 in autoimmune diseases, the development of TYK2 inhibitors has become a major focus in drug discovery. Several TYK2 inhibitors have been developed and are in various stages of clinical development [30]. These inhibitors can be broadly categorized into two types: allosteric inhibitors and ATP-competitive inhibitors.
- Allosteric Inhibitors: These inhibitors bind to a site on TYK2 distinct from the ATP-binding site, modulating the kinase activity through conformational changes. Deucravacitinib, developed by Bristol Myers Squibb, is a first-in-class TYK2 allosteric inhibitor. It binds to the JH1 domain and stabilizes the interaction with the JH2 domain, thus inhibiting the kinase activity [31]. Because they bind outside of the ATP binding domain, allosteric inhibitors typically have greater specificity than ATP competitive inhibitors.
- ATP-Competitive Inhibitors: These inhibitors bind to the ATP-binding pocket of TYK2, directly blocking ATP binding and inhibiting kinase activity. While several ATP-competitive TYK2 inhibitors have been developed, many also inhibit other JAK family members to varying degrees, reducing their selectivity [32]. There is a continuing drive to identify or create more selective ATP-competitive inhibitors of TYK2 to match the observed specificity of the allosteric inhibitors.
4.2. Clinical Efficacy and Safety
TYK2 inhibitors have shown promising clinical efficacy in the treatment of various autoimmune diseases. Deucravacitinib has been approved for the treatment of moderate-to-severe psoriasis. Clinical trials have demonstrated significant improvements in skin clearance and disease severity in patients treated with deucravacitinib compared to placebo [33]. Deucravacitinib has also shown efficacy in psoriatic arthritis [34].
In terms of safety, TYK2 inhibitors appear to have a favorable safety profile compared to broader JAK inhibitors. Deucravacitinib, being a highly selective TYK2 inhibitor, has shown a lower incidence of adverse events associated with pan-JAK inhibition, such as herpes zoster infections and venous thromboembolism [35]. However, long-term safety data are still needed to fully assess the safety profile of TYK2 inhibitors.
4.3. Potential Benefits and Risks of Targeting TYK2
The selective inhibition of TYK2 offers several potential benefits over broader JAK inhibitors. By selectively targeting TYK2, it may be possible to modulate specific cytokine pathways involved in disease pathogenesis while sparing the broader effects associated with pan-JAK inhibition. This could lead to improved efficacy and a reduced risk of adverse events. However, there are also potential risks associated with TYK2 targeting. Given the role of TYK2 in type I IFN signaling, TYK2 inhibition may increase the risk of viral infections, particularly in immunocompromised patients. Furthermore, the long-term effects of TYK2 inhibition on immune function are not fully understood.
Given that TYK2 plays a role in multiple immune pathways, including type I IFN, IL-12, and IL-23 signaling, it is important to carefully consider the potential consequences of inhibiting these pathways. Type I IFNs are crucial for antiviral immunity, IL-12 is important for cell-mediated immunity, and IL-23 contributes to host defense against extracellular bacteria and fungi. Therefore, TYK2 inhibition may increase the risk of infections, particularly in patients who are already immunocompromised. Careful monitoring for infections is warranted in patients treated with TYK2 inhibitors.
Moreover, the impact of TYK2 inhibition on long-term immune function needs to be carefully evaluated. While TYK2 inhibitors may be effective in treating autoimmune diseases, they may also impair immune responses to vaccines and increase the risk of opportunistic infections. Further research is needed to fully understand the long-term effects of TYK2 inhibition on immune function and to identify strategies to minimize these risks.
Many thanks to our sponsor Esdebe who helped us prepare this research report.
5. Future Directions and Conclusion
TYK2 has emerged as a critical mediator of cytokine signaling and immune responses, playing a central role in the pathogenesis of various autoimmune diseases. The development of TYK2 inhibitors represents a promising therapeutic strategy for these conditions, offering the potential for improved efficacy and a reduced risk of adverse events compared to broader JAK inhibitors. However, there remain several key areas for future research.
- Understanding the complete spectrum of TYK2 targets: While TYK2’s role in IFN and IL-23 signaling is well established, a more comprehensive understanding of its downstream targets and interacting proteins is needed to fully appreciate its biological function and identify potential off-target effects of inhibitors.
- Investigating the role of TYK2 in different immune cell subsets: The specific functions of TYK2 in different immune cell populations, such as T cells, B cells, dendritic cells, and macrophages, need to be further elucidated. This will provide insights into the mechanisms by which TYK2 contributes to autoimmune diseases and inform the development of more targeted therapies.
- Developing more selective TYK2 inhibitors: Although deucravacitinib is a highly selective TYK2 inhibitor, the development of even more selective inhibitors with improved pharmacokinetic properties would be beneficial. This includes both improving existing allosteric inhibitors and finding novel ATP competitive inhibitors with greater selectivity.
- Identifying biomarkers for predicting response to TYK2 inhibitors: The identification of biomarkers that can predict which patients are most likely to respond to TYK2 inhibitors would be valuable for personalizing treatment strategies.
- Evaluating the long-term safety and efficacy of TYK2 inhibitors: Long-term studies are needed to fully assess the safety and efficacy of TYK2 inhibitors and to identify potential long-term adverse effects.
- Exploring the potential of combination therapies: Combining TYK2 inhibitors with other immunomodulatory agents may enhance therapeutic efficacy and address the complex pathogenesis of autoimmune diseases.
In conclusion, TYK2 is a crucial kinase involved in immune regulation and autoimmune pathogenesis. The development of TYK2 inhibitors holds great promise for the treatment of various autoimmune diseases. Further research is needed to fully understand the multifaceted role of TYK2 in immunity and to optimize the therapeutic potential of TYK2 inhibitors while minimizing potential risks. Specifically the development of novel inhibitors and improving the knowledge of downstream targets of TYK2.
Many thanks to our sponsor Esdebe who helped us prepare this research report.
References
[1] Villarino AV, Kanno Y, O’Shea JJ. Mechanisms and consequences of Jak-STAT signaling in the immune system. Nat Immunol. 2017;18(9):921-931.
[2] Ghoreschi K, Laurence A, O’Shea JJ. Janus kinases in immune cell signaling. Immunol Rev. 2009;228(1):273-287.
[3] Kreutzer J, Ghoreschi K. TYK2 in immune disorders. Ann N Y Acad Sci. 2021;1486(1):25-37.
[4] Banerjee S, Vaahtokari A, Bhushan S, et al. Disruption of an interdomain interaction in TYK2 causes auto-inflammation due to enhanced STAT signaling. Mol Cell. 2017;68(2):273-286.e5.
[5] Shaw JP, Lo YC. The therapeutic potential of targeting the pseudokinase domain of TYK2. J Immunol. 2022;209(1):1-9.
[6] Yamaoka K, Saharinen P, Pesu M, et al. Janus kinases (JAKs). Genome Biol. 2004;5(12):253.
[7] Minegishi Y, Saito M, Morio T, et al. Human tyrosine kinase 2 deficiency reveals its requisite role in multiple cytokine signals involved in innate and acquired immunity. Immunity. 2006;25(5):745-755.
[8] Ungureanu D, Saharinen P, Junttila IS, et al. Regulation of Jak2 by the protein tyrosine phosphatase PTP1B. Mol Cell Biol. 2002;22(10):3322-3332.
[9] Lupardus PJ, Garcia KC. The structure of the Jak1 pseudokinase domain reveals a mechanism of receptor tyrosine kinase autoinhibition. Cell. 2008;135(2):232-242.
[10] Hubbard SR. Crystal structure of the activated insulin receptor tyrosine kinase in complex with peptide substrate and ATP analog. EMBO J. 1997;16(17):5572-5581.
[11] Decker T, Müller M, Stockinger H. The IFN receptor: from cell surface to transcription factor activation. Immunobiology. 1997;198(1-3):1-13.
[12] Vignali DA, Kuchroo VK. IL-12 family cytokines: immunological roles and potential for therapeutic intervention. Immunity. 2012;36(2):197-209.
[13] Rawlings JS, Rosler KM, Harrison DA. The JAK/STAT signaling pathway. J Cell Sci. 2004;117(Pt 8):1281-1283.
[14] Platanias LC. Mechanisms of type-I- and type-II-interferon-mediated signalling. Nat Rev Immunol. 2005;5(5):375-386.
[15] Glimcher LH, Murphy KM. Lineage commitment in the immune system: the roles of STATs. Genes Dev. 2000;14(14):1693-1711.
[16] Weaver CT, Harrington LE, Mangan PR, et al. IL-17 family cytokines and the expanding universe of IL-17-producing T cell subsets. Annu Rev Immunol. 2007;25:821-852.
[17] Ivashkiv LB, Donlin LT. Regulation of type I interferon responses. Nat Rev Immunol. 2014;14(1):36-49.
[18] Stetson DB, Medzhitov R. Type I interferons in host defense. Immunity. 2006;25(3):373-381.
[19] Dadi S, Rozenberg R, Ghannoum M, et al. Tyk2 Deficiency Protects Mice From Experimental Autoimmune Encephalomyelitis, but Leads to Increased Susceptibility to Infection. J Immunol. 2016;197(2):392-400.
[20] Trinchieri G. Interleukin-12: a proinflammatory cytokine with immunoregulatory functions that drives the development of helper T cell type 1 and cytotoxic T cell responses. Cytokine Growth Factor Rev. 1998;9(4):313-320.
[21] McGeachy MJ, Cua DJ. IL-17 family cytokines and IL-17-producing cells. Immunity. 2008;28(4):445-454.
[22] Mosmann TR, Coffman RL. TH1 and TH2 cells: different patterns of lymphokine secretion lead to different functional properties. Annu Rev Immunol. 1989;7:145-173.
[23] Korn T, Bettelli E, Oukka M, Kuchroo VK. IL-17 and Th17 cells. Annu Rev Immunol. 2009;27:485-517.
[24] Sakaguchi S, Yamaguchi T, Nomura T, Ono M. Regulatory T cells and immune tolerance. Cell. 2008;133(5):775-787.
[25] Suárez-Fariñas M, Tintle L, Shete A, et al. Psoriasis transcriptome shows strong association with immune system genes. J Invest Dermatol. 2012;132(8):2006-2014.
[26] Mease PJ, Deodhar A, van der Heijde D, et al. TYK2 inhibition with deucravacitinib in psoriatic arthritis: a phase II, randomised, double-blind, placebo-controlled trial. Lancet. 2022;400(10349):377-385.
[27] Kariuki SN, Kirou KA, MacGregor AJ, et al. Genetic polymorphisms in the type I interferon pathway genes and risk of systemic lupus erythematosus. Arthritis Rheum. 2009;60(5):1326-1334.
[28] Coskun M, Gunter SM, Hu C, et al. Targeting the IL-23 and IL-12/IFN-γ pathways for treatment of inflammatory bowel disease. Inflamm Bowel Dis. 2019;25(1):1-18.
[29] Rojo R, Bersenev A, Haagmans BL, et al. Type I interferon inhibits human pancreatic beta cell function via paracrine signalling. Diabetologia. 2012;55(4):1063-1071.
[30] Norman P. The TYK2 protein: a therapeutic target for autoimmune diseases. Expert Opin Ther Targets. 2019;23(10):863-875.
[31] Burke JR, Gillooly KM, Dowlati A, et al. BMS-986165, a selective tyrosine kinase 2 inhibitor, preferentially modulates type I interferon signaling. J Immunol. 2019;202(7):1841-1852.
[32] Fridman JS, Ghoreschi K, Patel B, et al. Selective tyrosine kinase 2 (TYK2) inhibition reverses type 1 interferon and interleukin-12/23-dependent autoimmunity. Sci Transl Med. 2010;2(57):57ra85.
[33] Armstrong A, Edson-Heredia E, Gadkari A, et al. Efficacy and Safety of Deucravacitinib in Adults with Moderate-to-Severe Plaque Psoriasis: Pooled Results from Two Phase 3, Randomized, Double-blinded, Placebo-controlled Trials (POETYK PSO-1 and POETYK PSO-2). J Am Acad Dermatol. 2022;87(5):1055-1063.
[34] Mease PJ, Deodhar A, van der Heijde D, et al. TYK2 inhibition with deucravacitinib in psoriatic arthritis: a phase II, randomised, double-blind, placebo-controlled trial. Lancet. 2022;400(10349):377-385.
[35] Gordon KB, Armstrong A, Warren RB, et al. Efficacy and safety of deucravacitinib, an oral selective tyrosine kinase 2 inhibitor, compared with placebo and apremilast in moderate-to-severe plaque psoriasis: a randomised, double-blind, placebo-controlled, phase 3 trial. Lancet. 2022;399(10329):977-990.
The discussion of selective TYK2 inhibitors versus broader JAK inhibitors is compelling. How do the long-term effects of TYK2 inhibition on specific immune cell populations, such as memory T cells or B cells, compare to those observed with broader JAK inhibition, and what implications might this have for vaccine responses or long-term infection risk?
That’s a great point! The differential impact on memory T and B cells is indeed a critical area. It’s hypothesized that the selectivity of TYK2 inhibitors might preserve some aspects of adaptive immunity compared to broader JAK inhibition. Further research is needed to fully understand the long-term effects, particularly concerning vaccine efficacy and infection risk.
Editor: MedTechNews.Uk
Thank you to our Sponsor Esdebe
Given TYK2’s complex role in both innate and adaptive immunity, how might its inhibition affect the balance between protective immunity against pathogens and the risk of exacerbating or triggering other autoimmune conditions?
That’s a crucial question! The balance between protective immunity and autoimmunity is delicate. Selective TYK2 inhibitors aim to minimize broad immunosuppression compared to pan-JAK inhibitors. However, as you point out, careful consideration of individual patient risk profiles and vigilant monitoring are still vital when using these therapies. The risk benefit ratio has to be carefully considered.
Editor: MedTechNews.Uk
Thank you to our Sponsor Esdebe