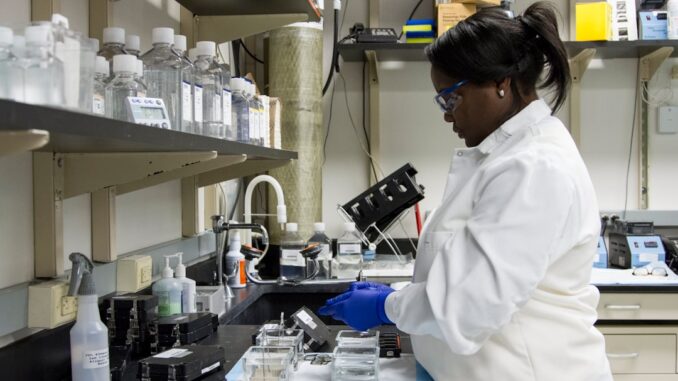
Abstract
Deoxyribonucleic acid (DNA) is the fundamental molecule of heredity, traditionally viewed through the lens of the central dogma: DNA encodes RNA, which encodes protein. While protein-coding sequences constitute a vital part of the genome, they represent only a small fraction of the total DNA. The remaining non-coding DNA (ncDNA), initially dismissed as “junk,” has emerged as a critical player in genome architecture, gene regulation, and cellular function. This report provides a comprehensive overview of DNA, encompassing its structure, organization within the nucleus, and the diverse functionalities of both coding and non-coding regions. We delve into the mechanisms by which ncDNA contributes to gene regulation, including its role in chromatin remodeling, transcription factor binding, and the production of non-coding RNAs. Further, we explore the implications of variations and mutations in ncDNA for complex diseases, with specific examples from cancer, neurodevelopmental disorders, and autoimmune conditions. We conclude by discussing the challenges and future directions in ncDNA research, highlighting the potential for novel therapeutic strategies targeting this previously overlooked genomic landscape.
Many thanks to our sponsor Esdebe who helped us prepare this research report.
1. Introduction
DNA, the carrier of genetic information, has been at the forefront of biological research since its discovery in the late 19th century and the subsequent elucidation of its double helical structure by Watson and Crick in 1953. The initial focus was primarily on the protein-coding regions of the genome, which direct the synthesis of proteins, the workhorses of the cell. However, advances in genomics have revealed that only a small percentage of the human genome, estimated to be around 1-2%, is directly translated into proteins. The remaining portion, ncDNA, was initially regarded as evolutionary baggage, lacking specific function. This view has been radically revised over the past two decades, with accumulating evidence demonstrating the crucial role of ncDNA in a wide array of cellular processes.
NcDNA encompasses a vast landscape of sequences, including introns, intergenic regions, pseudogenes, repetitive elements, and regulatory elements. These sequences play a vital role in maintaining genome integrity, regulating gene expression, and contributing to cellular differentiation and development. Dysregulation of ncDNA function has been implicated in a variety of diseases, including cancer, neurodevelopmental disorders, and autoimmune diseases. This report aims to provide a comprehensive overview of DNA, focusing on the structure, organization, and function of ncDNA, and exploring its implications for human health and disease.
Many thanks to our sponsor Esdebe who helped us prepare this research report.
2. Structure and Organization of DNA
2.1 The Double Helix and its Components
DNA is composed of two polynucleotide strands that wind around each other to form a double helix. Each strand consists of a sugar-phosphate backbone and a series of nitrogenous bases: adenine (A), guanine (G), cytosine (C), and thymine (T). The two strands are held together by hydrogen bonds between complementary base pairs: A pairs with T, and G pairs with C. This specific base pairing is fundamental to DNA replication and transcription, ensuring the accurate transmission of genetic information. The double helix exists in different forms, with the most common being the B-form, a right-handed helix with approximately 10.5 base pairs per turn. Other forms, such as A-DNA and Z-DNA, exist under specific conditions and may play regulatory roles.
2.2 Chromatin Structure and Genome Organization
Within the nucleus, DNA is packaged into a complex structure called chromatin. The basic unit of chromatin is the nucleosome, which consists of approximately 147 base pairs of DNA wrapped around a histone octamer. Histones are highly conserved proteins that play a crucial role in DNA compaction and regulation. Nucleosomes are further organized into higher-order structures, such as 30-nm fibers, which are then arranged into loops and domains. These structures are dynamic and can change in response to cellular signals, allowing access to specific regions of the genome for transcription and other processes.
The organization of the genome within the nucleus is not random. Chromosomes occupy specific territories, and certain regions of the genome are preferentially located near the nuclear periphery or the nuclear interior. This spatial organization can influence gene expression, with genes located in transcriptionally active regions being more accessible to transcription factors and RNA polymerase. Recent advances in chromosome conformation capture (3C) techniques, such as Hi-C, have provided insights into the three-dimensional organization of the genome and its role in gene regulation. These studies have revealed the existence of topologically associating domains (TADs), which are self-interacting genomic regions that limit the spread of regulatory elements. Disruption of TAD boundaries has been implicated in various diseases, including cancer.
2.3 DNA Replication and Repair
DNA replication is the process by which DNA is duplicated, ensuring the accurate transmission of genetic information to daughter cells. This process is catalyzed by DNA polymerase, which uses the existing DNA strand as a template to synthesize a new complementary strand. DNA replication is a highly accurate process, but errors can still occur. To maintain genome integrity, cells have evolved sophisticated DNA repair mechanisms. These mechanisms can detect and repair various types of DNA damage, including base modifications, DNA breaks, and cross-links. Failure of DNA repair mechanisms can lead to the accumulation of mutations, which can contribute to cancer and other diseases. Translesion synthesis is a last resort mechanism to bypass DNA damage that would otherwise stall replication, but it is error-prone, increasing the risk of mutation. The balance between accurate replication, faithful repair, and the potential for adaptive mutation through translesion synthesis is critical for genome stability and cellular adaptation.
Many thanks to our sponsor Esdebe who helped us prepare this research report.
3. The Functional Landscape of Non-Coding DNA
3.1 Regulatory Elements
Regulatory elements are DNA sequences that control gene expression by binding transcription factors (TFs) and other regulatory proteins. These elements include promoters, enhancers, silencers, and insulators. Promoters are located near the transcription start site of a gene and initiate transcription. Enhancers are located at variable distances from the promoter and can increase gene expression. Silencers, conversely, repress gene expression. Insulators define independent regulatory domains, preventing enhancers from activating promoters of neighboring genes. Regulatory elements are often located in ncDNA regions, highlighting the importance of ncDNA in gene regulation. Recent advancements such as CRISPR-based screening have allowed for systematic interrogation of non-coding regions, identifying novel regulatory elements with tissue-specific activity.
3.2 Non-Coding RNAs
NcRNAs are RNA molecules that are not translated into proteins but perform diverse functions in the cell. They are broadly categorized into small ncRNAs (less than 200 nucleotides) and long ncRNAs (lncRNAs, greater than 200 nucleotides).
MicroRNAs (miRNAs) are small ncRNAs that regulate gene expression by binding to messenger RNA (mRNA) molecules, leading to mRNA degradation or translational repression. They are involved in a wide range of biological processes, including development, differentiation, and apoptosis.
Long non-coding RNAs (lncRNAs) are a diverse class of ncRNAs that perform a variety of functions, including regulating gene expression, scaffolding protein complexes, and modulating chromatin structure. LncRNAs can act in cis (affecting genes on the same chromosome) or trans (affecting genes on different chromosomes). They have been implicated in various diseases, including cancer, neurodevelopmental disorders, and cardiovascular disease. Examples include Xist, which plays a critical role in X-chromosome inactivation, and HOTAIR, which regulates gene expression by recruiting chromatin-modifying complexes.
Other ncRNAs include piwi-interacting RNAs (piRNAs), small nucleolar RNAs (snoRNAs), and transfer RNAs (tRNAs), each with specific roles in RNA processing, ribosome biogenesis, and translation.
3.3 Repetitive Elements
Repetitive elements constitute a significant portion of the mammalian genome. These elements include transposons (also known as “jumping genes”), retrotransposons, and simple sequence repeats (SSRs). Transposons are DNA sequences that can move from one location in the genome to another. Retrotransposons are transcribed into RNA, which is then reverse-transcribed into DNA and inserted into a new location in the genome. SSRs are short DNA sequences that are repeated multiple times in tandem. Repetitive elements were initially considered “selfish” DNA, but recent studies have revealed that they play important roles in gene regulation and genome evolution. For example, some transposons contain regulatory elements that can influence the expression of nearby genes. Furthermore, retrotransposons can generate new genes or contribute to gene duplication.
3.4 Introns and Pseudogenes
Introns are non-coding sequences within genes that are transcribed into RNA but are removed by splicing before translation. Introns can contain regulatory elements that influence gene expression. Furthermore, introns can harbor miRNAs and other ncRNAs, adding another layer of complexity to gene regulation. Pseudogenes are non-functional copies of genes that have accumulated mutations that prevent them from being translated into proteins. While pseudogenes were once considered “dead genes,” recent studies have shown that they can play regulatory roles. Some pseudogenes can act as miRNA sponges, sequestering miRNAs and preventing them from binding to their target mRNAs. Others can be transcribed into RNA and regulate the expression of their cognate genes.
Many thanks to our sponsor Esdebe who helped us prepare this research report.
4. Aberrant Non-Coding DNA Function in Disease
4.1 Cancer
Aberrant ncDNA function is a hallmark of cancer. Mutations and epigenetic alterations in regulatory elements can lead to dysregulation of oncogenes and tumor suppressor genes. For example, mutations in the promoter region of the TERT gene, which encodes telomerase reverse transcriptase, can lead to increased telomerase expression and cellular immortalization. Alterations in lncRNA expression have also been implicated in cancer. Some lncRNAs can promote tumor growth and metastasis, while others can suppress tumor formation. For example, the lncRNA MALAT1 is upregulated in many cancers and promotes metastasis. Conversely, the lncRNA GAS5 is downregulated in some cancers and acts as a tumor suppressor. Reactivation of transposable elements has also been reported in several cancer types, driving genomic instability and inflammatory responses. Furthermore, alterations in the three-dimensional organization of the genome, such as disruption of TAD boundaries, can lead to aberrant gene expression and contribute to tumorigenesis. The cancer genome atlas (TCGA) and other large-scale genomic projects have revealed the complexity of ncDNA alterations in cancer and have identified potential therapeutic targets.
4.2 Neurodevelopmental Disorders
Neurodevelopmental disorders, such as autism spectrum disorder (ASD) and schizophrenia, are complex disorders that are thought to arise from a combination of genetic and environmental factors. While mutations in protein-coding genes have been implicated in these disorders, accumulating evidence suggests that alterations in ncDNA also play a significant role. Mutations in regulatory elements can lead to dysregulation of genes involved in brain development and function. For example, deletions and duplications in the 16p11.2 region, which contains several genes involved in neuronal development, are associated with ASD. However, the mechanisms by which these deletions and duplications lead to ASD are not fully understood. It is possible that they disrupt the expression of genes located outside of the deleted or duplicated region, highlighting the importance of non-coding regulatory elements located within this region. Changes in lncRNA expression have also been implicated in neurodevelopmental disorders. For example, the lncRNA MEG3 is dysregulated in ASD and is thought to regulate the expression of genes involved in synaptic function. Epigenetic modifications of ncDNA, such as DNA methylation and histone modifications, can also contribute to neurodevelopmental disorders. These modifications can alter the accessibility of DNA to transcription factors and other regulatory proteins, leading to changes in gene expression. Epigenome-wide association studies (EWAS) have identified several differentially methylated regions (DMRs) in the brains of individuals with neurodevelopmental disorders, suggesting that epigenetic dysregulation plays a critical role in these conditions.
4.3 Autoimmune Diseases
Autoimmune diseases are characterized by an inappropriate immune response against the body’s own tissues. Genetic factors play a significant role in the development of autoimmune diseases, but environmental factors also contribute. While mutations in protein-coding genes have been implicated in autoimmune diseases, accumulating evidence suggests that alterations in ncDNA also play a role. Mutations in regulatory elements can lead to dysregulation of genes involved in immune function. For example, single nucleotide polymorphisms (SNPs) in the promoter region of the IL2RA gene, which encodes the interleukin-2 receptor alpha chain, are associated with an increased risk of multiple sclerosis. These SNPs can alter the binding affinity of transcription factors to the IL2RA promoter, leading to changes in IL2RA expression and immune dysregulation. Alterations in lncRNA expression have also been implicated in autoimmune diseases. For example, the lncRNA NEAT1 is upregulated in systemic lupus erythematosus (SLE) and is thought to promote inflammation. Epigenetic modifications of ncDNA can also contribute to autoimmune diseases. For example, DNA methylation patterns are altered in T cells from individuals with rheumatoid arthritis, suggesting that epigenetic dysregulation plays a role in this condition.
Many thanks to our sponsor Esdebe who helped us prepare this research report.
5. Challenges and Future Directions
Despite the significant advances in our understanding of ncDNA function, several challenges remain. One of the major challenges is the sheer complexity of the ncDNA landscape. The human genome contains a vast number of ncDNA sequences, and it is difficult to determine the function of each sequence. Furthermore, ncDNA functions can be cell-type specific and context-dependent, adding another layer of complexity. Another challenge is the lack of high-throughput methods for studying ncDNA function. While methods such as CRISPR-based screening have been developed, they are still relatively time-consuming and expensive.
Future research should focus on developing new technologies for studying ncDNA function at high resolution and in a cell-type-specific manner. This will require integrating genomics, epigenomics, transcriptomics, and proteomics data to build a comprehensive understanding of ncDNA function. Furthermore, it will be important to develop computational tools for predicting ncDNA function based on sequence features and epigenetic modifications. Another important area of research is the development of therapeutic strategies targeting ncDNA. This could involve designing drugs that target specific ncRNAs or regulatory elements, or developing gene therapy approaches to correct aberrant ncDNA function. For example, antisense oligonucleotides (ASOs) can be used to silence lncRNAs that promote tumor growth. These ASOs bind to the lncRNA and prevent it from interacting with its target molecules. Furthermore, CRISPR-based gene editing can be used to correct mutations in regulatory elements or to insert new regulatory elements into the genome. The challenges are significant, but the potential rewards are enormous. By harnessing the power of ncDNA, we can develop new treatments for a wide range of diseases.
Many thanks to our sponsor Esdebe who helped us prepare this research report.
6. Conclusion
DNA, beyond its role as a template for protein synthesis, possesses a complex and multifaceted functionality largely attributed to its non-coding regions. This ncDNA orchestrates gene regulation, genome architecture, and cellular processes with remarkable precision. Aberrant function of ncDNA is increasingly recognized as a key contributor to a spectrum of human diseases, from cancer to neurodevelopmental and autoimmune disorders. While significant strides have been made in elucidating the roles of ncDNA, many challenges remain in fully understanding its intricate functions and developing effective therapeutic strategies targeting this vast and previously underappreciated genomic landscape. Continued research, driven by technological innovation and integrative approaches, promises to unlock the full potential of ncDNA in understanding and treating complex diseases, marking a significant shift in the paradigm of genetic medicine.
Many thanks to our sponsor Esdebe who helped us prepare this research report.
References
- Mattick, J. S., & Dinger, M. E. (2013). The functions of noncoding RNA. Molecular cell, 42(4), 456-468.
- ENCODE Project Consortium. (2012). An integrated encyclopedia of DNA elements in the human genome. Nature, 489(7414), 57-74.
- Djebali, S., Davis, C. A., Merkel, A., Dobin, A., Lassmann, T., Tanzer, A., … & Wold, B. (2012). Landscape of transcription in human cells. Nature, 489(7414), 101-108.
- Costa, F. F. (2010). Non-coding RNAs: new players in eukaryotic biology. Gene, 468(1-2), 1-10.
- Ponting, C. P., Oliver, P. L., & Reik, W. (2009). Evolution and functions of long noncoding RNAs. Cell, 136(4), 629-641.
- Kapusta, A., Kronenberg, Z., Lynch, V. J., Zhu, Q., Ramsay, R., & Feschotte, C. (2013). Transposable elements are major contributors to the origin, diversification, and regulation of vertebrate long noncoding RNAs. PLoS genetics, 9(5), e1003470.
- Li, W., Notani, D., Rosenfeld, M. G. (2016). Enhancers as non-coding RNA transcription units: perspectives in developmental biology, endocrinology, and cancer. Molecular cell, 59(5), 683-692.
- Rinn, J. L., & Chang, H. Y. (2012). Genome regulation by long noncoding RNAs. Annual review of biochemistry, 81, 145-166.
- Gil, N., & Ulitsky, I. (2017). Regulation of gene expression by pseudogenes. Nature Reviews Genetics, 18(3), 175-189.
- Lander, E. S., Linton, L. M., Birren, B., Nusbaum, C., Zody, M. C., Baldwin, J., … & Waterston, R. H. (2001). Initial sequencing and analysis of the human genome. Nature, 409(6822), 860-921.
- Dekker, J., Rippe, K., Dekker, J., & Rippe, K. (2013). Capturing chromosome conformation. Science, 342(6159), 733-737.
- Dixon, J. R., Selvaraj, S., Yue, F., Kim, A., Li, Y., Shen, Y., … & Ren, B. (2012). Topological domains in mammalian genomes identified by analysis of chromatin interactions. Nature, 485(7398), 376-380.
- Flavahan, W. A., Gaskell, E., & Bernstein, B. E. (2017). Epigenetic plasticity and the hallmarks of cancer. Science, 357(6357), 126-132.
- Esteller, M. (2008). Epigenetics in cancer. New England Journal of Medicine, 358(11), 1148-1159.
- Gibb, E. A., Vucic, E. A., Enfield, K. S., Stewart, G. L., Litherland, S. A., Creighton, C. J., … & Brown, C. J. (2011). Human cancer long non-coding RNA expression profiles identify novel potential biomarkers. PloS one, 6(9), e23982.
- Pauli, A., Norris, M. L., Valen, E., Chew, G. L., & Gagnon, J. A. (2015). Antisense lncRNAs guide mRNA localization by base pairing. Cell, 162(3), 584-595.
- Ebert, M. S., & Sharp, P. A. (2012). Roles for microRNAs in conferring robustness to biological processes. Cell, 149(4), 750-759.
- St Laurent III, G., Wahlestedt, C., & Kapranov, P. (2015). The landscape of long noncoding RNA classification. Trends in Genetics, 31(5), 239-251.
- Consortium, G. T. E. (2015). Genetic effects on gene expression across human tissues. Nature, 526(7575), 648-650.
- Werling, A. M., Geschwind, D. H. (2013). Understanding sex differences in autism. Proceedings of the National Academy of Sciences, 110(13), 4868-4869.
- De Rubeis, S., He, X., Goldberg, A. P., Poultney, C. S., Samocha, K. E., Cicek, A. E., … & Buxbaum, J. D. (2014). Synaptic, transcriptional and chromatin genes disrupted in autism. Nature, 515(7526), 209-215.
- Parikshak, N. N., Luo, Y., Zhang, A., Brandys, B. A., & Geschwind, D. H. (2016). Integrative functional genomic analyses implicate specific molecular pathways and circuits in autism. Cell, 167(3), 792-805. e17.
- Lord, C., Elsabbagh, M., Baird, G., & Veenstra-VanderWeele, J. (2018). Autism spectrum disorder. The Lancet, 392(10146), 508-520.
- International Multiple Sclerosis Genetics Consortium. (2011). Genetic risk and a primary role for cell-mediated immune mechanisms in multiple sclerosis. Nature, 476(7359), 214-219.
- Coornaert, B., Baeten, D., & De Bosscher, K. (2008). Interleukin-10 and its receptors. Cytokine & growth factor reviews, 19(2), 155-168.
- Harley, J. B., Alarcón-Riquelme, M. E., Criswell, L. A., Jacob, C. O., Kimberly, R. P., Korman, B. D., … & Vyse, T. J. (2008). Genome-wide association scan in women with systemic lupus erythematosus identifies susceptibility variants in ITGAM, PXK, KIAA1542 and IRF5. Nature genetics, 40(2), 204-210.
- Ko, K. H., Lee, J., Kim, J. Y., Lee, E. Y., Song, Y. W., & Lee, S. K. (2018). Genome-wide DNA methylation analysis of CD4+ T cells in rheumatoid arthritis reveals altered methylation patterns and potential epigenetic regulation of gene expression. Arthritis research & therapy, 20(1), 1-12.
- Iyer, M. K., Niknafs, Y. S., Malik, R., Singhal, U., Bharadwaj, A., Logan, A. C., … & Chinnaiyan, A. M. (2015). The landscape of long noncoding RNAs in the human transcriptome. Nature genetics, 47(3), 199-208.
- Harrow, J., Frankish, A., Gonzalez, J. M., Tapanari, E., Diekhans, M., Zerbino, D. R., … & Hubbard, T. J. (2012). GENCODE: the reference human genome annotation for The ENCODE Project. Genome research, 22(9), 1760-1774.
- Quinodoz, S. A., Ollikainen, N., Armenise, C., McDonel, P., & Guttman, M. (2021). Long noncoding RNAs encode exonic structures that regulate transcription and splicing. Molecular cell, 81(13), 2765-2780.e8.
Given the complexity of ncDNA and challenges in determining the function of each sequence, what new high-throughput technologies are most promising for studying ncDNA function in a cell-type-specific manner, and how might we integrate the resulting multi-omics data effectively?
That’s a great question! High-throughput technologies like single-cell ATAC-seq and CRISPR screens are promising, especially when combined with advanced bioinformatics. Integrating multi-omics data effectively requires robust computational models and visualization tools to reveal the complex interplay of ncDNA, gene expression, and cellular phenotypes. Looking forward to further discussion on this!
Editor: MedTechNews.Uk
Thank you to our Sponsor Esdebe
“Junk DNA,” eh? Seems like our definition of “junk” just keeps shrinking! I wonder what else we’re overlooking in the vast, unexplored territories of the genome? Perhaps personalized medicine will get a boost from understanding this better?
That’s a fantastic point! The shift in perspective on non-coding DNA is really reshaping how we approach personalized medicine. If we can fully understand how variations in these regions influence gene expression, treatments can be tailored with greater precision. What undiscovered opportunities do you think this opens up?
Editor: MedTechNews.Uk
Thank you to our Sponsor Esdebe