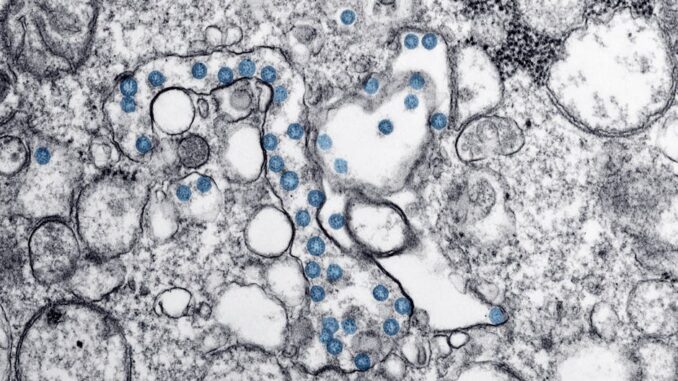
Abstract
Neurodegenerative diseases, including Alzheimer’s disease (AD), Parkinson’s disease (PD), and multiple sclerosis (MS), represent a significant and growing global health burden. While neuronal dysfunction and loss are central to the pathology of these disorders, the contribution of glial cells has emerged as a critical area of investigation. This review provides a comprehensive overview of the diverse roles of glial cells – astrocytes, microglia, oligodendrocytes, and NG2-glia – in the pathogenesis of neurodegenerative diseases, extending beyond their well-established involvement in inflammation and plaque formation. We examine the complex interplay between different glial cell types and their influence on neuronal survival, synaptic plasticity, and disease progression. Furthermore, we delve into the molecular mechanisms underlying glial dysfunction and highlight emerging therapeutic strategies targeting glial cells for the treatment of neurodegenerative diseases. We will discuss the challenges and future directions in glial cell research with an emphasis on the need for a deeper understanding of glial heterogeneity and cell-specific responses to pathological stimuli to develop effective therapies.
Many thanks to our sponsor Esdebe who helped us prepare this research report.
1. Introduction
For many years, neurons held the central stage in neuroscience research, while glial cells were often relegated to a supportive role, providing structural integrity and metabolic sustenance. However, this view has dramatically shifted over the past two decades. It is now widely recognized that glial cells, comprising approximately half of the brain’s cellular population, are not merely passive bystanders but active participants in virtually all aspects of brain function, from neural development and synaptic transmission to neuroinflammation and neurodegeneration. These intricate roles are executed by a diverse cast of glial cell types, each with unique morphologies, functions, and responses to various stimuli. The focus of this review is neurodegenerative diseases which place a heavy burden on society and families.
In the context of neurodegenerative diseases, such as AD, PD, Huntington’s disease (HD), and amyotrophic lateral sclerosis (ALS), the traditional neuron-centric view has proven insufficient to fully explain the complex pathophysiology of these disorders. While neuronal dysfunction and death are undeniably central to the clinical manifestations of these diseases, emerging evidence implicates glial cells as key drivers of disease progression. Specifically, glial cells are now known to contribute to neuroinflammation, oxidative stress, excitotoxicity, and the disruption of neuronal circuits, all of which contribute to neuronal damage and cognitive decline.
Beyond their established roles in inflammation and plaque formation in AD, glial cells exhibit a range of diverse and often context-dependent functions that can either exacerbate or ameliorate neurodegeneration. For instance, astrocytes can provide neurotrophic support and clear glutamate from the synaptic cleft, thereby protecting neurons from excitotoxicity. However, under pathological conditions, astrocytes can become reactive and release pro-inflammatory cytokines, contributing to neuroinflammation and neuronal damage. Similarly, microglia, the resident immune cells of the brain, can phagocytose cellular debris and release neurotrophic factors, promoting tissue repair. However, chronic activation of microglia can lead to the sustained release of pro-inflammatory mediators, exacerbating neurodegeneration.
Therefore, a comprehensive understanding of the multifaceted roles of glial cells in neurodegenerative diseases is essential for developing effective therapeutic strategies. This review aims to provide an overview of the diverse functions of glial cells in the pathogenesis of neurodegenerative diseases, focusing on the complex interplay between different glial cell types and their influence on neuronal survival, synaptic plasticity, and disease progression. We will also discuss the challenges and future directions in glial cell research, with an emphasis on the need for a deeper understanding of glial heterogeneity and cell-specific responses to pathological stimuli to develop targeted therapies.
Many thanks to our sponsor Esdebe who helped us prepare this research report.
2. Glial Cell Types and their Basic Functions
The central nervous system (CNS) is populated by several distinct types of glial cells, each with specialized functions that contribute to the overall health and function of the brain and spinal cord. The major types of glial cells include astrocytes, microglia, oligodendrocytes, and NG2-glia (also known as oligodendrocyte precursor cells). A brief overview of their basic functions is outlined below:
2.1 Astrocytes: Astrocytes are the most abundant glial cell type in the brain and play a critical role in maintaining homeostasis, supporting neuronal function, and modulating synaptic transmission. Key functions of astrocytes include:
- Structural Support: Astrocytes provide structural support to neurons by forming a network of processes that surround neurons and blood vessels.
- Metabolic Support: Astrocytes provide neurons with essential nutrients, such as glucose and lactate, and remove metabolic waste products.
- Regulation of Extracellular Environment: Astrocytes regulate the extracellular concentration of ions, neurotransmitters, and other molecules, maintaining optimal conditions for neuronal function. Astrocytes express high levels of glutamate transporters, playing a crucial role in preventing excitotoxicity.
- Blood-Brain Barrier (BBB) Maintenance: Astrocytes contribute to the formation and maintenance of the BBB, a selective barrier that protects the brain from harmful substances in the bloodstream. Astrocytic end-feet surround blood vessels and secrete factors that promote the tight junctions between endothelial cells.
- Synaptic Modulation: Astrocytes participate in synaptic transmission by releasing gliotransmitters, such as glutamate, ATP, and D-serine, which can modulate neuronal activity. They also ensheath synapses, influencing synaptic plasticity and communication.
2.2 Microglia: Microglia are the resident immune cells of the CNS and play a crucial role in immune surveillance, phagocytosis, and inflammation. Key functions of microglia include:
- Immune Surveillance: Microglia constantly survey the brain for signs of injury, infection, or abnormal protein aggregates.
- Phagocytosis: Microglia engulf and remove cellular debris, pathogens, and protein aggregates, promoting tissue repair and preventing the spread of inflammation.
- Inflammation: Microglia release pro-inflammatory cytokines and chemokines, which can activate other immune cells and contribute to neuroinflammation. However, microglia can also release anti-inflammatory cytokines, promoting resolution of inflammation and tissue repair.
- Synaptic Pruning: Microglia participate in synaptic pruning, a process by which unnecessary or weak synapses are eliminated during development and in response to injury or disease.
2.3 Oligodendrocytes: Oligodendrocytes are responsible for myelinating axons in the CNS, a process that is essential for rapid and efficient nerve impulse conduction. Key functions of oligodendrocytes include:
- Myelination: Oligodendrocytes wrap their processes around axons, forming a myelin sheath that insulates the axon and increases the speed of action potential propagation.
- Trophic Support: Oligodendrocytes provide trophic support to axons, promoting neuronal survival and function.
2.4 NG2-glia (Oligodendrocyte Precursor Cells): NG2-glia are a population of glial cells that can differentiate into oligodendrocytes and contribute to remyelination after injury. Key functions of NG2-glia include:
- Proliferation and Migration: NG2-glia proliferate and migrate to areas of injury or demyelination.
- Differentiation: NG2-glia differentiate into mature oligodendrocytes and contribute to remyelination.
- Synaptic Modulation: NG2-glia receive synaptic input from neurons and can modulate neuronal activity.
In summary, each type of glial cell has distinct functions that are essential for maintaining brain homeostasis, supporting neuronal function, and responding to injury or disease. Understanding the complex interplay between different glial cell types is crucial for developing effective therapeutic strategies for neurodegenerative diseases.
Many thanks to our sponsor Esdebe who helped us prepare this research report.
3. Glial Cell Dysfunction in Alzheimer’s Disease
Alzheimer’s disease (AD) is characterized by the progressive accumulation of amyloid-beta (Aβ) plaques and neurofibrillary tangles (NFTs) in the brain, leading to neuronal dysfunction and cognitive decline. While Aβ and NFTs have long been considered the primary drivers of AD pathogenesis, emerging evidence highlights the critical role of glial cells in the development and progression of the disease. Specifically, astrocytes and microglia exhibit profound changes in their morphology, function, and gene expression in response to Aβ and NFTs, contributing to neuroinflammation, synaptic dysfunction, and neuronal loss.
3.1 Astrogliosis in AD: Astrogliosis, characterized by the hypertrophy and proliferation of astrocytes, is a prominent feature of AD pathology. Reactive astrocytes surrounding Aβ plaques and NFTs exhibit increased expression of glial fibrillary acidic protein (GFAP), a marker of astrocyte activation. Reactive astrocytes can contribute to both beneficial and detrimental effects in AD.
- Beneficial Effects: Astrocytes can promote Aβ clearance by secreting enzymes that degrade Aβ and by facilitating Aβ transport across the BBB. Astrocytes can also provide neurotrophic support to neurons and protect them from excitotoxicity by clearing glutamate from the synaptic cleft.
- Detrimental Effects: Reactive astrocytes can release pro-inflammatory cytokines, such as IL-1β and TNF-α, contributing to neuroinflammation and neuronal damage. Astrocytes can also contribute to the formation of Aβ plaques by secreting Aβ and by promoting the aggregation of Aβ. Furthermore, reactive astrocytes can impair synaptic transmission by disrupting the formation and maintenance of synapses.
3.2 Microglial Activation in AD: Microglial activation is another prominent feature of AD pathology. Microglia surrounding Aβ plaques and NFTs exhibit increased expression of activation markers, such as CD68 and Iba-1, and release pro-inflammatory cytokines. Microglia can also contribute to both beneficial and detrimental effects in AD.
- Beneficial Effects: Microglia can phagocytose Aβ plaques and cellular debris, promoting Aβ clearance and tissue repair. Microglia can also release neurotrophic factors, such as BDNF and NGF, which promote neuronal survival and function.
- Detrimental Effects: Chronic activation of microglia can lead to the sustained release of pro-inflammatory cytokines, such as IL-1β, TNF-α, and IL-6, exacerbating neuroinflammation and neuronal damage. Microglia can also release reactive oxygen species (ROS) and reactive nitrogen species (RNS), which can cause oxidative stress and neuronal damage. Furthermore, microglia can contribute to synaptic loss by phagocytosing synapses.
3.3 Oligodendrocytes in AD: Whilst traditionally less studied in AD, emerging evidence suggests that oligodendrocytes and myelin are significantly affected in AD, contributing to cognitive decline. Myelin deficits may impair neuronal communication and exacerbate neurodegeneration.
- Myelin Degradation: Studies have shown myelin degradation and oligodendrocyte dysfunction in AD brains, potentially impairing axonal integrity and neuronal communication.
- Oligodendrocyte Loss: Some evidence suggests oligodendrocyte loss occurs in AD, exacerbating the impact on myelin integrity.
In summary, glial cell dysfunction plays a critical role in the pathogenesis of AD, contributing to neuroinflammation, synaptic dysfunction, and neuronal loss. Understanding the complex interplay between different glial cell types and their responses to Aβ and NFTs is essential for developing effective therapeutic strategies for AD. Targeting glial cells to reduce neuroinflammation, promote Aβ clearance, and protect synapses may represent a promising avenue for AD therapy.
Many thanks to our sponsor Esdebe who helped us prepare this research report.
4. Glial Cell Involvement in Parkinson’s Disease
Parkinson’s disease (PD) is a neurodegenerative disorder characterized by the progressive loss of dopaminergic neurons in the substantia nigra pars compacta (SNpc), leading to motor deficits, such as tremor, rigidity, and bradykinesia. While neuronal loss is the hallmark of PD, emerging evidence suggests that glial cells, particularly astrocytes and microglia, play a significant role in the pathogenesis of the disease. Specifically, glial cells contribute to neuroinflammation, oxidative stress, and the accumulation of α-synuclein, a protein that forms Lewy bodies, the pathological hallmark of PD.
4.1 Astrogliosis in PD: Similar to AD, astrogliosis is a prominent feature of PD pathology. Reactive astrocytes in the SNpc and other brain regions exhibit increased expression of GFAP and release pro-inflammatory cytokines. Reactive astrocytes can contribute to both beneficial and detrimental effects in PD.
- Beneficial Effects: Astrocytes can provide neurotrophic support to dopaminergic neurons and protect them from oxidative stress by releasing antioxidants, such as glutathione. Astrocytes can also clear glutamate from the synaptic cleft, preventing excitotoxicity.
- Detrimental Effects: Reactive astrocytes can release pro-inflammatory cytokines, such as IL-1β and TNF-α, contributing to neuroinflammation and neuronal damage. Astrocytes can also contribute to the aggregation of α-synuclein by secreting α-synuclein and by promoting the misfolding of α-synuclein. Furthermore, reactive astrocytes can impair dopaminergic neurotransmission by disrupting the synthesis, release, and reuptake of dopamine.
4.2 Microglial Activation in PD: Microglial activation is also a prominent feature of PD pathology. Microglia in the SNpc and other brain regions exhibit increased expression of activation markers and release pro-inflammatory cytokines. Microglia can also contribute to both beneficial and detrimental effects in PD.
- Beneficial Effects: Microglia can phagocytose α-synuclein aggregates and cellular debris, promoting tissue repair and preventing the spread of α-synuclein pathology. Microglia can also release neurotrophic factors, such as GDNF and BDNF, which promote dopaminergic neuron survival and function.
- Detrimental Effects: Chronic activation of microglia can lead to the sustained release of pro-inflammatory cytokines, exacerbating neuroinflammation and neuronal damage. Microglia can also release ROS and RNS, causing oxidative stress and neuronal damage. Furthermore, microglia can contribute to the spread of α-synuclein pathology by releasing exosomes containing α-synuclein.
4.3 Oligodendrocytes in PD: Emerging studies point to a role for oligodendrocytes and myelin deficits in the pathophysiology of PD. These deficits are not only present in motor circuits but also in non-motor areas affected in PD, suggesting a broader impact of oligodendrocyte dysfunction on disease progression.
- Myelin Degradation: Studies have shown myelin degradation in PD brains. This can impair neural network communication, especially in motor circuits, further impacting motor function.
- Oligodendrocyte Loss: Some studies suggest oligodendrocyte loss in PD, which can contribute to the overall degradation of myelin integrity.
In summary, glial cell dysfunction plays a critical role in the pathogenesis of PD, contributing to neuroinflammation, oxidative stress, and the accumulation of α-synuclein. Targeting glial cells to reduce neuroinflammation, promote α-synuclein clearance, and protect dopaminergic neurons may represent a promising avenue for PD therapy. Furthermore, assessing oligodendrocyte function and myelin integrity could reveal new therapeutic targets for PD.
Many thanks to our sponsor Esdebe who helped us prepare this research report.
5. Glial Cells in Multiple Sclerosis
Multiple sclerosis (MS) is a chronic inflammatory and neurodegenerative disease of the CNS characterized by demyelination, axonal damage, and neuronal loss. In MS, the immune system attacks myelin, the protective sheath surrounding nerve fibers, leading to a wide range of neurological symptoms, including motor dysfunction, sensory disturbances, and cognitive impairment. Glial cells, particularly oligodendrocytes, astrocytes, and microglia, play a central role in the pathogenesis of MS.
5.1 Oligodendrocyte Dysfunction in MS: Oligodendrocyte dysfunction and demyelination are the pathological hallmarks of MS. In MS, the immune system attacks oligodendrocytes, leading to their destruction and the subsequent loss of myelin. Demyelination impairs nerve impulse conduction, leading to neurological dysfunction.
- Immune-mediated Damage: Autoimmune responses directly target oligodendrocytes, resulting in cell death and myelin breakdown. The precise mechanisms underlying oligodendrocyte susceptibility to immune attack are still under investigation.
- Remyelination Failure: In MS, the capacity for remyelination is often impaired, leading to persistent demyelination and axonal damage. Factors that contribute to remyelination failure include inflammation, oxidative stress, and the lack of sufficient oligodendrocyte precursor cells.
5.2 Astrogliosis in MS: Astrogliosis is a prominent feature of MS pathology, particularly in areas of demyelination. Reactive astrocytes in MS lesions exhibit increased expression of GFAP and release pro-inflammatory cytokines. Reactive astrocytes can contribute to both beneficial and detrimental effects in MS.
- Beneficial Effects: Astrocytes can promote remyelination by releasing growth factors and by clearing debris from the site of injury. Astrocytes can also provide neurotrophic support to neurons and protect them from excitotoxicity.
- Detrimental Effects: Reactive astrocytes can release pro-inflammatory cytokines, exacerbating inflammation and neuronal damage. Astrocytes can also form a glial scar, which can inhibit remyelination and axonal regeneration.
5.3 Microglial Activation in MS: Microglial activation is also a prominent feature of MS pathology. Microglia in MS lesions exhibit increased expression of activation markers and release pro-inflammatory cytokines. Microglia can also contribute to both beneficial and detrimental effects in MS.
- Beneficial Effects: Microglia can phagocytose myelin debris and cellular debris, promoting tissue repair and preventing the spread of inflammation. Microglia can also release neurotrophic factors, which promote oligodendrocyte survival and remyelination.
- Detrimental Effects: Chronic activation of microglia can lead to the sustained release of pro-inflammatory cytokines, exacerbating inflammation and neuronal damage. Microglia can also release ROS and RNS, causing oxidative stress and axonal damage. Furthermore, microglia can contribute to demyelination by directly attacking oligodendrocytes and myelin.
In summary, glial cell dysfunction plays a critical role in the pathogenesis of MS, contributing to demyelination, axonal damage, and neuronal loss. Targeting glial cells to promote remyelination, reduce neuroinflammation, and protect axons may represent a promising avenue for MS therapy.
Many thanks to our sponsor Esdebe who helped us prepare this research report.
6. Emerging Therapeutic Strategies Targeting Glial Cells
The growing recognition of the crucial roles of glial cells in neurodegenerative diseases has fueled the development of novel therapeutic strategies targeting these cells. These strategies aim to modulate glial cell activity to reduce neuroinflammation, promote neuroprotection, and enhance tissue repair. Several promising therapeutic approaches are currently under investigation:
6.1 Modulation of Microglial Activation: Targeting microglia to reduce neuroinflammation has emerged as a promising therapeutic strategy for neurodegenerative diseases. Several approaches are being explored:
- Inhibition of Pro-inflammatory Cytokine Production: Inhibiting the production or release of pro-inflammatory cytokines, such as IL-1β, TNF-α, and IL-6, can reduce neuroinflammation and protect neurons from damage. Several drugs that target these cytokines are currently in clinical trials for neurodegenerative diseases.
- Promotion of Microglial Phagocytosis: Enhancing the phagocytic activity of microglia can promote the clearance of Aβ plaques, α-synuclein aggregates, and myelin debris, reducing the burden of pathological protein aggregates and promoting tissue repair. Strategies to enhance phagocytosis include stimulating microglial receptors, such as TREM2, and using antibodies to opsonize pathological proteins.
- Repolarization of Microglia: Repolarizing microglia from a pro-inflammatory (M1) phenotype to an anti-inflammatory (M2) phenotype can promote resolution of inflammation and tissue repair. Strategies to repolarize microglia include using anti-inflammatory cytokines, such as IL-4 and IL-10, and using drugs that inhibit pro-inflammatory signaling pathways.
6.2 Enhancement of Astrocyte Function: Targeting astrocytes to enhance their neuroprotective functions is another promising therapeutic strategy for neurodegenerative diseases. Several approaches are being explored:
- Promotion of Neurotrophic Factor Release: Enhancing the release of neurotrophic factors, such as BDNF and GDNF, from astrocytes can promote neuronal survival and function. Strategies to enhance neurotrophic factor release include stimulating astrocyte receptors, such as TrkB and GFRα1, and using drugs that increase neurotrophic factor expression.
- Regulation of Glutamate Homeostasis: Restoring glutamate homeostasis can protect neurons from excitotoxicity. Strategies to regulate glutamate homeostasis include enhancing glutamate transporter expression in astrocytes and using drugs that block glutamate receptors.
- Modulation of Astrocytic Inflammatory Signaling: Targeting inflammatory pathways within astrocytes can reduce neuroinflammation and protect neurons from damage. This can involve inhibiting the release of pro-inflammatory cytokines from astrocytes.
6.3 Promotion of Remyelination: Promoting remyelination is a key therapeutic goal for MS. Several approaches are being explored:
- Enhancement of Oligodendrocyte Precursor Cell (OPC) Differentiation: Stimulating the differentiation of OPCs into mature oligodendrocytes can promote remyelination and restore nerve impulse conduction. Strategies to enhance OPC differentiation include using growth factors, such as PDGF and FGF, and using drugs that inhibit pathways that suppress OPC differentiation.
- Inhibition of Remyelination Inhibitors: Blocking the activity of remyelination inhibitors, such as Nogo-A and LINGO-1, can promote remyelination and restore nerve impulse conduction. Several antibodies that target these inhibitors are currently in clinical trials for MS.
- Stem Cell Therapy: Transplantation of stem cells that can differentiate into oligodendrocytes can provide a source of new oligodendrocytes for remyelination. Several clinical trials are currently evaluating the safety and efficacy of stem cell therapy for MS.
6.4 Gene Therapy: Delivering genes that encode therapeutic proteins directly into glial cells can provide a sustained and targeted approach to treating neurodegenerative diseases. Gene therapy can be used to express neurotrophic factors, anti-inflammatory cytokines, or enzymes that degrade pathological proteins.
In summary, emerging therapeutic strategies targeting glial cells hold great promise for the treatment of neurodegenerative diseases. These strategies aim to modulate glial cell activity to reduce neuroinflammation, promote neuroprotection, and enhance tissue repair. Further research is needed to optimize these approaches and to identify the most effective glial cell targets for specific neurodegenerative diseases.
Many thanks to our sponsor Esdebe who helped us prepare this research report.
7. Challenges and Future Directions
Despite the significant progress in understanding the roles of glial cells in neurodegenerative diseases, several challenges remain. Overcoming these challenges is essential for developing effective glial-targeted therapies.
7.1 Glial Heterogeneity: Glial cells are a heterogeneous population, with distinct subtypes exhibiting different functions and responses to pathological stimuli. Understanding the specific roles of different glial subtypes in neurodegenerative diseases is crucial for developing targeted therapies. Single-cell RNA sequencing and other high-throughput technologies are being used to characterize glial heterogeneity and to identify subtype-specific markers.
7.2 Complex Glial-Neuronal Interactions: Glial cells interact with neurons in complex ways, and these interactions can be altered in neurodegenerative diseases. Understanding the mechanisms underlying glial-neuronal interactions is crucial for developing therapies that restore normal glial-neuronal communication. Co-culture systems and in vivo imaging techniques are being used to study glial-neuronal interactions.
7.3 Temporal Dynamics of Glial Activation: Glial activation is a dynamic process that changes over time in neurodegenerative diseases. Understanding the temporal dynamics of glial activation is crucial for developing therapies that target the appropriate glial responses at different stages of disease progression. Longitudinal studies and advanced imaging techniques are being used to track glial activation over time.
7.4 Translation from Preclinical Models to Clinical Trials: Many glial-targeted therapies have shown promise in preclinical models but have failed to translate to clinical trials. This may be due to differences between preclinical models and human disease, as well as the complexity of human neurodegenerative diseases. Developing more relevant preclinical models and using biomarkers to identify patients who are most likely to respond to glial-targeted therapies are crucial for improving translation.
7.5 Delivery of Therapeutics to Glial Cells: Delivering therapeutics specifically to glial cells can be challenging due to the BBB and the difficulty of targeting specific glial subtypes. Developing novel drug delivery systems that can cross the BBB and target specific glial subtypes is crucial for improving the efficacy of glial-targeted therapies. Nanoparticles and viral vectors are being explored as potential drug delivery systems.
In conclusion, glial cell research is a rapidly evolving field with great potential to improve the treatment of neurodegenerative diseases. Addressing the challenges outlined above and continuing to invest in glial cell research is essential for translating basic science discoveries into effective therapies for these devastating disorders.
Many thanks to our sponsor Esdebe who helped us prepare this research report.
8. Conclusion
This review has highlighted the multifaceted roles of glial cells in neurodegenerative diseases, extending beyond their established involvement in inflammation and plaque formation. We have examined the diverse functions of astrocytes, microglia, oligodendrocytes, and NG2-glia in the pathogenesis of AD, PD, and MS, emphasizing the complex interplay between different glial cell types and their influence on neuronal survival, synaptic plasticity, and disease progression. Furthermore, we have discussed emerging therapeutic strategies targeting glial cells, including modulation of microglial activation, enhancement of astrocyte function, and promotion of remyelination. Finally, we have addressed the challenges and future directions in glial cell research, emphasizing the need for a deeper understanding of glial heterogeneity, complex glial-neuronal interactions, temporal dynamics of glial activation, translation from preclinical models to clinical trials, and delivery of therapeutics to glial cells.
Targeting glial cells represents a promising avenue for the development of novel therapies for neurodegenerative diseases. However, further research is needed to optimize these approaches and to identify the most effective glial cell targets for specific diseases. By addressing the challenges outlined in this review, we can pave the way for the development of effective glial-targeted therapies that improve the lives of patients suffering from neurodegenerative diseases.
Many thanks to our sponsor Esdebe who helped us prepare this research report.
References
[Please note that the following references are representative examples and a comprehensive list would be much longer.]
- Barres, B. A. (2008). The mystery and magic of glia: a perspective on their reactivity and role in disease. Neuron, 60(3), 430-447.
- Sofroniew, M. V. (2015). Astrocyte reactivity: subtypes, states, and functions in the healthy and diseased brain. Trends in neurosciences, 38(1), 40-55.
- Ransohoff, R. M., & El Khoury, J. (2015). Microglia in health and disease. Cold Spring Harbor perspectives in biology, 8(1), a020560.
- Nave, K. A., & Werner, H. B. (2014). Myelination of the nervous system: structure, function, and pathology. Cold Spring Harbor perspectives in biology, 6(4), a018029.
- Heneka, M. T., Golenbock, D. T., Latz, E., Morgan, D., Brown, R., McCoy, M. G., … & Kummer, M. P. (2015). The inflammasome in Alzheimer’s disease. Nature, 526(7573), 47.
- Chung, W. S., Welsh, C. A., Barres, B. A. (2015). Astrocyte-secreted proteins signal via neuronal EGFR to promote synapse maturation. Neuron, 87(2), 454.
- Subramaniam, S. R., & Federoff, H. J. (2017). Targeting Neuroinflammation in Parkinson’s Disease. Experimental Neurology, 298, 1-16.
- Jha, M. K., Choi, D. H., & Kim, T. D. (2019). Microglia-Mediated Neuroinflammation: Role in Neurodegenerative Diseases. Antioxidants, 8(6), 204.
- Franklin, R. J., & Ffrench-Constant, C. (2008). Remyelination in the CNS: from biology to therapy. Nature Reviews Neuroscience, 9(11), 839-855.
- Gacias, M., Szyndrowski, K., Ivanovic, A., Kijewska, M., LeBlond, C., & Kossut, M. (2021). Oligodendrocyte dysfunction in Alzheimer’s disease. Brain Communications, 3(3), fcab120.
- Bradl, M., & Hohlfeld, R. (2003). The role of astrocytes in inflammatory neurodegenerative diseases. Journal of Neurology, 250(6), 649-657.
This is a great review highlighting the critical and complex roles of glial cells in neurodegenerative diseases. The discussion around the temporal dynamics of glial activation is particularly interesting, and further research here could reveal key therapeutic time windows.
Thank you for your insightful comment! We agree that understanding the temporal dynamics of glial activation is crucial. Pinpointing these therapeutic windows could revolutionize treatment strategies. Exploring how glial responses evolve over time in different neurodegenerative diseases is definitely a key focus for future research.
Editor: MedTechNews.Uk
Thank you to our Sponsor Esdebe
The discussion of glial heterogeneity is fascinating. Could further research into astrocyte subtypes, particularly their diverse responses to pathological stimuli, lead to more targeted and effective therapeutic interventions in neurodegenerative conditions?