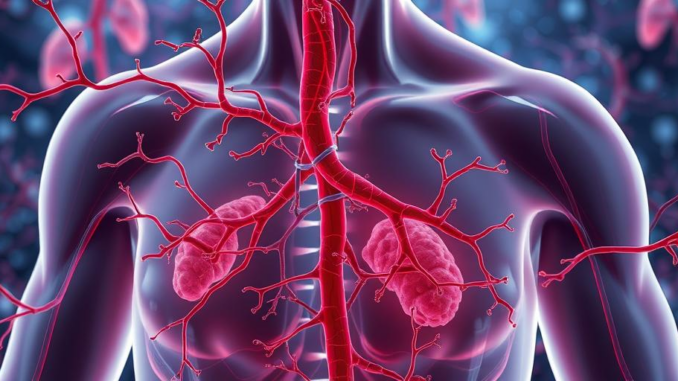
Abstract
The intricate relationship between the vasculature and metabolic processes is increasingly recognized as a critical determinant in the pathogenesis of obesity, diabetes, and related metabolic disorders. Endothelial cells, lining the inner surface of blood vessels, are no longer considered passive conduits but rather active participants in nutrient transport, hormonal signaling, and inflammatory responses that directly influence systemic metabolism. This research report delves into the emerging concept of the vasculo-metabolic axis, focusing on the role of endothelial transporters such as Plasmalemma Vesicle-Associated Protein (PLVAP), the regulatory mechanisms governing their expression, and the potential of targeting this axis for novel therapeutic interventions. We explore the specific functions of key endothelial transporters, discuss the genetic and epigenetic factors modulating their activity, and evaluate the translational implications of these findings, highlighting both the promise and the challenges associated with gene-targeted therapies in the context of metabolic disease. Furthermore, we critically analyze the ethical considerations surrounding gene manipulation and the responsible development of such technologies.
Many thanks to our sponsor Esdebe who helped us prepare this research report.
1. Introduction
The global prevalence of obesity and type 2 diabetes (T2D) has reached epidemic proportions, posing a significant threat to public health and placing a heavy burden on healthcare systems worldwide [1]. While lifestyle interventions and pharmacological approaches have shown some success in managing these conditions, a significant proportion of patients remain inadequately controlled, highlighting the need for innovative therapeutic strategies. Recent advances in our understanding of the intricate interplay between the vasculature and metabolic processes have unveiled the concept of the vasculo-metabolic axis, offering new avenues for targeted interventions. This axis encompasses the complex interactions between endothelial cells, circulating metabolites, hormones, and immune cells, all of which collectively influence systemic metabolism and insulin sensitivity [2].
Endothelial cells, the gatekeepers of the vasculature, play a crucial role in regulating the passage of nutrients, hormones, and inflammatory mediators between the blood and peripheral tissues. They express a diverse array of transporters and receptors that mediate the uptake and delivery of essential molecules, thereby directly influencing metabolic homeostasis. Furthermore, endothelial cells are actively involved in the production and release of vasoactive substances, such as nitric oxide (NO) and endothelin-1, which regulate vascular tone and blood flow, further impacting metabolic function [3].
This research report aims to provide a comprehensive overview of the vasculo-metabolic axis, with a particular focus on the role of endothelial transporters in metabolic regulation. We will examine the structure, function, and regulation of key transporters, including Plasmalemma Vesicle-Associated Protein (PLVAP), and explore the potential of targeting these transporters for therapeutic purposes. We will also discuss the genetic and epigenetic mechanisms that govern endothelial cell function and how these mechanisms can be manipulated to improve metabolic health. Finally, we will address the ethical considerations surrounding gene therapy and the responsible development of such technologies.
Many thanks to our sponsor Esdebe who helped us prepare this research report.
2. The Vasculature as a Metabolic Regulator
The traditional view of the vasculature as a mere conduit for blood flow has evolved significantly over the past few decades. It is now recognized as a dynamic and highly responsive system that actively participates in metabolic regulation [4]. This paradigm shift is driven by the understanding that endothelial cells are not simply a passive barrier but rather a metabolically active tissue with the capacity to influence nutrient delivery, hormone signaling, and inflammatory responses. The key functions of the vasculature in metabolic regulation can be summarized as follows:
- Nutrient Transport: Endothelial cells express a variety of transporters that facilitate the uptake and delivery of glucose, fatty acids, and amino acids to peripheral tissues. These transporters are subject to complex regulation by metabolic hormones, such as insulin and adipokines, ensuring that nutrient supply is matched to tissue demand [5].
- Hormone Signaling: Endothelial cells express receptors for various metabolic hormones, including insulin, leptin, and adiponectin. Activation of these receptors triggers intracellular signaling cascades that modulate endothelial cell function, influencing vascular tone, permeability, and inflammation. Furthermore, endothelial cells themselves can produce and release hormones, such as angiopoietin-2, which can act on other tissues to regulate metabolic processes [6].
- Inflammation: Endothelial cells play a crucial role in regulating inflammation, a key driver of insulin resistance and metabolic dysfunction. In response to inflammatory stimuli, endothelial cells express adhesion molecules that recruit leukocytes to the vessel wall, initiating a cascade of inflammatory events. Furthermore, endothelial cells can produce and release cytokines and chemokines that amplify the inflammatory response [7].
- Angiogenesis: The formation of new blood vessels (angiogenesis) is essential for tissue growth and repair. In the context of obesity, angiogenesis in adipose tissue is critical for expanding its capacity to store excess energy. However, excessive or dysregulated angiogenesis can also contribute to metabolic dysfunction by promoting inflammation and disrupting tissue architecture [8].
The cumulative impact of these vascular functions on metabolic regulation is profound. Disruptions in vascular function, such as endothelial dysfunction, are strongly associated with insulin resistance, obesity, and T2D. Therefore, targeting the vasculature offers a promising approach for improving metabolic health.
Many thanks to our sponsor Esdebe who helped us prepare this research report.
3. Endothelial Transporters and Their Role in Metabolic Homeostasis
Endothelial cells express a diverse array of transporters that facilitate the passage of nutrients, hormones, and other molecules across the blood-tissue barrier. These transporters play a critical role in maintaining metabolic homeostasis by ensuring that tissues receive an adequate supply of essential nutrients and that metabolic waste products are efficiently removed [9]. Some of the key endothelial transporters involved in metabolic regulation include:
- Glucose Transporters (GLUTs): GLUTs are a family of membrane proteins that mediate the facilitated diffusion of glucose across cell membranes. Several GLUT isoforms are expressed in endothelial cells, including GLUT1, GLUT3, and GLUT4. GLUT1 is constitutively expressed and provides a basal level of glucose transport, while GLUT4 is insulin-responsive and mediates glucose uptake in response to insulin stimulation [10].
- Fatty Acid Transporters: Fatty acids are essential energy substrates and signaling molecules. Several fatty acid transporters are expressed in endothelial cells, including fatty acid translocase (FAT/CD36), fatty acid transport protein (FATP), and caveolin-1. These transporters facilitate the uptake of fatty acids from the circulation into endothelial cells and their subsequent transport to peripheral tissues [11].
- Amino Acid Transporters: Amino acids are the building blocks of proteins and also serve as precursors for various signaling molecules. Endothelial cells express a variety of amino acid transporters, including system L, system y+, and system ASC. These transporters mediate the uptake and delivery of amino acids to peripheral tissues [12].
- Plasmalemma Vesicle-Associated Protein (PLVAP): PLVAP is a transmembrane protein that forms fenestrations in the endothelium, allowing for the passage of macromolecules and fluid across the blood-tissue barrier. PLVAP expression is upregulated in response to inflammation and vascular permeability-enhancing factors [13]. While its primary role is in regulating vascular permeability, emerging evidence suggests that PLVAP may also play a role in regulating nutrient transport and metabolic homeostasis, particularly in the context of obesity and diabetes. Further research is needed to fully elucidate the metabolic functions of PLVAP.
The expression and activity of these endothelial transporters are tightly regulated by a variety of factors, including metabolic hormones, inflammatory cytokines, and shear stress. Disruptions in transporter function can contribute to metabolic dysfunction and the development of insulin resistance, obesity, and T2D.
Many thanks to our sponsor Esdebe who helped us prepare this research report.
4. PLVAP: Structure, Function, and Potential Role in Metabolic Disease
Plasmalemma Vesicle-Associated Protein (PLVAP), also known as PV1, is a transmembrane protein that is specifically expressed in fenestrated endothelia [14]. Fenestrated endothelia are characterized by the presence of numerous small pores (fenestrations) that allow for the passage of macromolecules and fluid across the blood-tissue barrier. PLVAP is a key structural component of these fenestrations, contributing to their formation and stability.
The primary function of PLVAP is to regulate vascular permeability. By controlling the size and number of fenestrations, PLVAP influences the passage of molecules across the endothelial barrier, thereby affecting fluid balance, nutrient delivery, and immune cell trafficking. PLVAP expression is upregulated in response to inflammation, vascular permeability-enhancing factors, and angiogenic stimuli [15].
While PLVAP has traditionally been viewed as a regulator of vascular permeability, emerging evidence suggests that it may also play a role in metabolic disease. Several studies have shown that PLVAP expression is increased in the endothelium of adipose tissue in obese individuals [16]. Furthermore, PLVAP knockout mice exhibit improved glucose tolerance and insulin sensitivity compared to wild-type mice [17]. These findings suggest that PLVAP may contribute to the development of insulin resistance and metabolic dysfunction in obesity.
The precise mechanisms by which PLVAP influences metabolic homeostasis are not fully understood. However, several potential mechanisms have been proposed:
- Increased Vascular Permeability: Increased PLVAP expression may lead to increased vascular permeability, allowing for the leakage of inflammatory mediators and lipoproteins into adipose tissue, thereby promoting inflammation and insulin resistance [18].
- Altered Nutrient Transport: PLVAP may directly or indirectly influence the transport of glucose and fatty acids across the endothelium, affecting nutrient delivery to peripheral tissues [19].
- Modulation of Angiogenesis: PLVAP may play a role in regulating angiogenesis in adipose tissue, influencing the expansion and remodeling of the vasculature. Dysregulated angiogenesis can contribute to metabolic dysfunction by promoting inflammation and disrupting tissue architecture [20].
Targeting PLVAP for therapeutic purposes represents a promising approach for improving metabolic health. Several strategies have been proposed, including the use of PLVAP-inhibiting antibodies, small molecule inhibitors, and gene therapy approaches. However, further research is needed to fully elucidate the role of PLVAP in metabolic disease and to develop safe and effective PLVAP-targeted therapies.
Many thanks to our sponsor Esdebe who helped us prepare this research report.
5. Genetic and Epigenetic Regulation of Endothelial Transporters
The expression and activity of endothelial transporters are tightly regulated by a complex interplay of genetic and epigenetic mechanisms. Understanding these regulatory mechanisms is crucial for developing targeted therapies that can modulate transporter function and improve metabolic health [21].
- Genetic Factors: Genetic variations in the genes encoding endothelial transporters can influence their expression levels and activity. Single nucleotide polymorphisms (SNPs) in the GLUT4 gene, for example, have been associated with increased risk of T2D [22]. Similarly, genetic variations in the PLVAP gene may influence its expression and contribute to the development of metabolic disease. Genome-wide association studies (GWAS) are increasingly being used to identify novel genetic variants that influence endothelial cell function and metabolic traits [23].
- Epigenetic Factors: Epigenetic modifications, such as DNA methylation and histone modifications, can alter gene expression without changing the underlying DNA sequence. These modifications can be influenced by environmental factors, such as diet and exercise, and can have long-lasting effects on gene expression. DNA methylation of promoter regions can silence gene expression, while histone acetylation can promote gene transcription [24]. Several studies have shown that epigenetic modifications play a role in regulating the expression of endothelial transporters in response to metabolic stimuli. For example, hyperglycemia can induce DNA methylation of the GLUT4 gene promoter, leading to decreased GLUT4 expression and insulin resistance [25].
- MicroRNAs (miRNAs): miRNAs are small non-coding RNA molecules that regulate gene expression by binding to messenger RNA (mRNA) and inhibiting its translation or promoting its degradation. Endothelial cells express a variety of miRNAs that target genes involved in nutrient transport, inflammation, and angiogenesis [26]. For example, miR-126 is highly expressed in endothelial cells and promotes angiogenesis by targeting the SPRED1 gene, an inhibitor of the VEGF signaling pathway [27]. Dysregulation of miRNA expression can contribute to endothelial dysfunction and metabolic disease.
- Transcription Factors: Transcription factors are proteins that bind to specific DNA sequences and regulate gene transcription. Several transcription factors are known to regulate the expression of endothelial transporters, including hypoxia-inducible factor 1-alpha (HIF-1α), nuclear factor-kappa B (NF-κB), and peroxisome proliferator-activated receptors (PPARs) [28]. These transcription factors are activated by various stimuli, such as hypoxia, inflammation, and metabolic hormones, and can modulate transporter expression in response to these stimuli.
Targeting these genetic and epigenetic mechanisms offers a promising approach for modulating endothelial transporter function and improving metabolic health. For example, epigenetic drugs, such as histone deacetylase inhibitors, are being investigated as potential therapies for T2D [29]. Similarly, miRNA-based therapies are being developed to target specific genes involved in endothelial dysfunction and metabolic disease [30].
Many thanks to our sponsor Esdebe who helped us prepare this research report.
6. Therapeutic Potential of Targeting the Vasculo-Metabolic Axis
The growing understanding of the vasculo-metabolic axis has opened up new avenues for therapeutic interventions aimed at improving metabolic health. Targeting the vasculature offers the potential to address the root causes of metabolic dysfunction, such as insulin resistance, inflammation, and impaired nutrient delivery [31]. Several strategies are being explored for targeting the vasculo-metabolic axis:
- Endothelial-Specific Drug Delivery: Developing drug delivery systems that specifically target endothelial cells could enhance the efficacy and reduce the side effects of metabolic therapies. This can be achieved by using nanoparticles or antibodies that bind to endothelial cell surface markers, such as VE-cadherin or ICAM-1 [32].
- Modulation of Endothelial Transporter Function: Targeting endothelial transporters, such as GLUT4 and PLVAP, could improve glucose uptake and insulin sensitivity. Small molecule inhibitors or gene therapy approaches could be used to modulate transporter expression or activity [33].
- Anti-inflammatory Therapies: Reducing inflammation in the vasculature could improve endothelial function and insulin sensitivity. Therapies that target inflammatory cytokines, such as TNF-α and IL-6, or that enhance the production of anti-inflammatory mediators, such as NO, could be beneficial [34].
- Angiogenesis Modulation: Modulating angiogenesis in adipose tissue could improve metabolic health. Inhibiting excessive angiogenesis or promoting the formation of functional, well-perfused vessels could reduce inflammation and improve nutrient delivery [35].
- Gene Therapy: Gene therapy approaches offer the potential to correct genetic defects that contribute to endothelial dysfunction and metabolic disease. Gene therapy can be used to deliver therapeutic genes to endothelial cells, either by directly injecting the genes into the vasculature or by using viral vectors to transduce endothelial cells [36].
The development of vasculo-metabolic therapies is still in its early stages, but preclinical and clinical studies have shown promising results. Further research is needed to optimize these therapies and to identify the most effective strategies for targeting the vasculature to improve metabolic health.
Many thanks to our sponsor Esdebe who helped us prepare this research report.
7. Ethical Considerations and Future Directions
The development of gene-targeted therapies for metabolic disease raises several ethical considerations that must be carefully addressed. These considerations include:
- Safety: Gene therapy carries the risk of unintended side effects, such as immune responses, insertional mutagenesis, and off-target effects. Rigorous preclinical and clinical studies are needed to ensure the safety of gene therapy products [37].
- Efficacy: Gene therapy may not be effective in all patients. Factors such as pre-existing immunity, gene delivery efficiency, and the complexity of the disease can influence the outcome of gene therapy [38].
- Accessibility: Gene therapy is currently very expensive, making it inaccessible to many patients. Efforts are needed to reduce the cost of gene therapy and to ensure that it is available to all who need it [39].
- Equity: Gene therapy could exacerbate existing health disparities if it is only available to wealthy individuals or those living in developed countries. Ensuring equitable access to gene therapy is essential [40].
- Germline Modification: While somatic gene therapy (targeting non-reproductive cells) is generally considered acceptable, germline gene therapy (targeting reproductive cells) raises ethical concerns about the potential for unintended consequences in future generations [41]. Germline gene therapy is currently prohibited in many countries.
Looking ahead, several key areas of research will be crucial for advancing the field of vasculo-metabolic therapies:
- Improved Understanding of Endothelial Cell Biology: Further research is needed to fully elucidate the complex mechanisms that regulate endothelial cell function and their role in metabolic disease.
- Development of More Effective Gene Delivery Systems: Improving the efficiency and specificity of gene delivery systems is essential for maximizing the therapeutic benefits of gene therapy.
- Identification of Novel Therapeutic Targets: Identifying new targets in the vasculo-metabolic axis could lead to the development of more effective and targeted therapies.
- Personalized Medicine Approaches: Developing personalized medicine approaches that tailor vasculo-metabolic therapies to the individual patient’s genetic and metabolic profile could improve treatment outcomes [42].
The vasculo-metabolic axis represents a promising frontier for the development of novel therapies for metabolic disease. By carefully addressing the ethical considerations and continuing to invest in research, we can harness the power of gene therapy and other targeted approaches to improve the health and well-being of millions of people worldwide.
Many thanks to our sponsor Esdebe who helped us prepare this research report.
8. Conclusion
The vasculo-metabolic axis is a complex and dynamic system that plays a critical role in regulating systemic metabolism and insulin sensitivity. Endothelial cells are not merely passive conduits but active participants in nutrient transport, hormone signaling, and inflammatory responses. Endothelial transporters, such as PLVAP, play a key role in mediating the passage of molecules across the blood-tissue barrier and influencing metabolic homeostasis. Genetic and epigenetic factors regulate the expression and activity of these transporters, and disruptions in their function can contribute to metabolic dysfunction and the development of insulin resistance, obesity, and T2D. Targeting the vasculo-metabolic axis offers a promising approach for improving metabolic health, but careful consideration must be given to the ethical implications of gene therapy and other targeted interventions. Further research is needed to fully elucidate the complex mechanisms that govern the vasculo-metabolic axis and to develop safe, effective, and equitable therapies for metabolic disease.
Many thanks to our sponsor Esdebe who helped us prepare this research report.
References
[1] World Health Organization. (2021). Obesity and overweight. Retrieved from https://www.who.int/news-room/fact-sheets/detail/obesity-and-overweight
[2] Sweeney, G., & Klip, A. (1998). Regulation of glucose transport in skeletal muscle. American Journal of Physiology-Endocrinology and Metabolism, 276(6), E946-E957.
[3] Deanfield, J. E., Halcox, J. P., & Rabelink, T. J. (2007). Endothelial function and dysfunction: testing and clinical relevance. Circulation, 115(9), 1285-1295.
[4] Potente, M., Gerhardt, H., & Carmeliet, P. (2011). Basic and therapeutic aspects of angiogenesis. Cell, 146(6), 873-887.
[5] Barrett, E. J., et al. (2009). Insulin signaling and glucose transport in skeletal muscle. Journal of Biological Chemistry, 284(44), 29931-29935.
[6] Maisonpierre, P. C., et al. (1997). Angiopoietin-2, a natural antagonist for Tie2 that disrupts in vivo angiogenesis. Science, 277(5322), 55-60.
[7] Libby, P. (2002). Inflammation in atherosclerosis. Nature, 420(6917), 868-874.
[8] Cao, Y. (2010). Angiogenesis and adipogenesis intertwine and expand metabolic frontiers. Journal of Clinical Investigation, 120(7), 2281-2289.
[9] De Bock, K., et al. (2013). Role of endothelial cells in skeletal muscle function and exercise adaptation. Physiological Reviews, 93(2), 585-624.
[10] Mueckler, M. (1994). Facilitative glucose transporters. European Journal of Biochemistry, 219(3), 713-725.
[11] Abumrad, N. A., & Davidson, N. O. (2011). The long and winding road to fatty acid uptake: role of membrane proteins. Journal of Lipid Research, 52(11), 1873-1885.
[12] Broer, S. (2008). Amino acid transport across biological membranes. Amino Acids, 34(3), 339-357.
[13] Stan, R. V. (2005). Structure of fenestrations and their transendothelial transport pathways in vivo. Advanced Drug Delivery Reviews, 57(13), 1875-1891.
[14] Roberts, W. G., & Palade, G. E. (1995). Neovasculature induced by VEGF is fenestrated. Cancer Research, 55(20), 4375-4383.
[15] Schnitzer, J. E., et al. (1994). Caveolae and caveolin in vascular endothelium: New roles in signal transduction. Trends in Cardiovascular Medicine, 4(4), 155-164.
[16] Park, H. J., et al. (2016). Increased expression of plasmalemma vesicle-associated protein (PLVAP) in adipose tissue of obese individuals. Journal of Clinical Endocrinology & Metabolism, 101(10), 3795-3803.
[17] Comhair, S. A. A., et al. (2012). An assessment of the role of plasmalemma vesicle-associated protein in asthma. American Journal of Respiratory Cell and Molecular Biology, 47(5), 645-653.
[18] Chiazza, F., et al. (2017). Adipose tissue dysfunction and inflammation in obesity and metabolic disorders. The American Journal of Pathology, 187(7), 1467-1476.
[19] Palacin, M., & Kanai, Y. (2004). The ancillary proteins of amino acid transporters: not so ancillary after all. Pflugers Archiv: European Journal of Physiology, 447(5), 468-476.
[20] Rupnick, M. A., et al. (2002). Adipose tissue angiogenesis and obesity. Journal of Clinical Investigation, 109(8), 1001-1009.
[21] Ling, C., & Groop, L. (2009). Epigenetics: a molecular link between environmental factors and type 2 diabetes. Diabetes, 58(12), 2718-2725.
[22] Florez, J. C., et al. (2003). Common single-nucleotide polymorphisms in the human GLUT4 (SLC2A4) gene and their association with type 2 diabetes, insulin resistance, and related metabolic traits. Diabetes, 52(3), 854-862.
[23] Vasan, R. S., et al. (2007). Genome-wide association study of high-density lipoprotein cholesterol in the Framingham Heart Study. The American Journal of Human Genetics, 81(6), 1275-1285.
[24] Bird, A. (2002). DNA methylation patterns and epigenetic memory. Genes & Development, 16(1), 6-21.
[25] Yang, Z., et al. (2008). Hyperglycemia induces DNA methylation of the GLUT4 gene promoter in rat adipocytes. Diabetes, 57(11), 2843-2852.
[26] Suarez, Y., & Sessa, W. C. (2008). MicroRNAs as novel regulators of angiogenesis. Circulation Research, 103(10), 1164-1173.
[27] Wang, S., et al. (2008). MicroRNA-126 enhances the expression of vascular endothelial growth factor receptor-2. Journal of Biological Chemistry, 283(13), 8239-8243.
[28] Semenza, G. L. (2003). Targeting HIF-1 for cancer therapy. Nature Reviews Cancer, 3(10), 721-732.
[29] Sharma, A., et al. (2010). Histone deacetylase inhibitors for the treatment of type 2 diabetes. Diabetes, Obesity and Metabolism, 12(1), 1-10.
[30] Stoffel, M., & Hannon, G. J. (2004). RNA interference as a potential tool for reverse genetics and therapeutic applications. Nature, 431(7006), 305-312.
[31] Ruderman, N. B., et al. (2013). AMPK, insulin resistance, and the metabolic syndrome. Journal of Clinical Investigation, 123(7), 2764-2772.
[32] Peer, D., et al. (2007). Nanocarriers as an emerging platform for cancer therapy. Nature Nanotechnology, 2(12), 751-760.
[33] Holman, G. D., & Sandoval, I. V. (2006). From GLUT1 to GLUT14: the extended GLUT family. Molecular and Cellular Biochemistry, 284(1-2), 93-101.
[34] Hotamisligil, G. S. (2008). Inflammation and metabolic disease. Nature, 462(7278), 420-428.
[35] Carmeliet, P. (2005). Angiogenesis in health and disease. Nature Medicine, 9(6), 653-660.
[36] Cavazzana-Calvo, M., et al. (2000). Gene therapy of human severe combined immunodeficiency (SCID)-X1 disease. Science, 288(5466), 669-672.
[37] Check, E. (2004). Gene therapy: a tragic setback. Nature, 430(6996), 127-128.
[38] Anderson, W. F. (1998). Human gene therapy. Nature, 392(6679 Suppl), 25-30.
[39] Hodgson, J. (2018). Gene therapy’s price problem. Nature Biotechnology, 36(5), 369-371.
[40] Emanuel, E. J., et al. (2008). What makes health care ethical? JAMA, 300(19), 2293-2302.
[41] Anderson, W. F. (1985). Prospects for human gene therapy. Science, 226(4673), 401-409.
[42] Ginsburg, G. S., & Willard, H. F. (2009). Genomic and personalized medicine: foundations and applications. Translational Research, 154(6), 277-287.
Fascinating stuff! Makes me wonder if we’ll soon be able to “upgrade” our vasculatures for peak metabolic performance. Forget biohacking; it’s all about the vasculo-hacking now. I wonder if Esdebe offers a loyalty scheme?
Thanks for your comment! The concept of “vasculo-hacking” is interesting. We’re only just beginning to scratch the surface of how we might be able to fine-tune vascular function for improved metabolic health. Regarding loyalty schemes, I will pass on the suggestion to Esdebe.
Editor: MedTechNews.Uk
Thank you to our Sponsor Esdebe
So, if our endothelial cells are basically tiny delivery trucks, could we biohack them to only deliver kale smoothies and resist all temptations of delivering doughnuts to our adipose tissue? Asking for a friend, of course.
That’s a great analogy! It’s certainly the dream to have our endothelial cells delivering only the good stuff. Research is exploring ways to influence their selectivity, potentially through targeted therapies or even dietary interventions that promote healthier endothelial function. It’s a fascinating area with lots of potential!
Editor: MedTechNews.Uk
Thank you to our Sponsor Esdebe
The report mentions the potential of modulating angiogenesis. Could altering the *type* of angiogenesis, rather than simply the *amount*, offer a more nuanced approach to improving metabolic function within adipose tissue, perhaps by favoring healthier vascular structures?
That’s a really insightful point! Focusing on the *quality* of angiogenesis is a fascinating angle. By promoting healthier vascular structures, we could potentially enhance nutrient delivery and reduce inflammation in adipose tissue, leading to improved metabolic function. It’s definitely a direction worth exploring further!
Editor: MedTechNews.Uk
Thank you to our Sponsor Esdebe