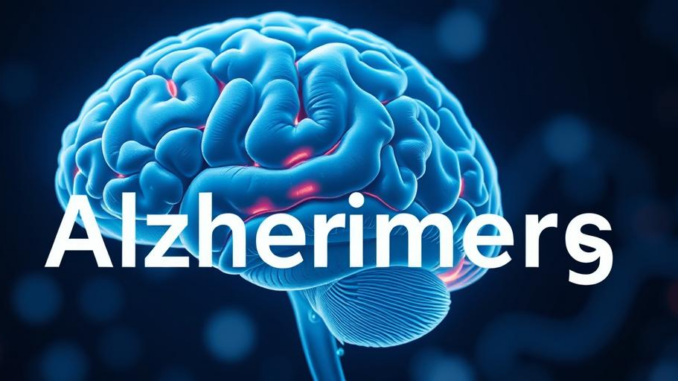
Abstract
Alzheimer’s disease (AD) represents a formidable challenge to global health, characterized by progressive cognitive decline and neurodegeneration. While significant advancements have been made in understanding the underlying pathophysiology of AD, effective disease-modifying therapies remain elusive. This review provides a comprehensive overview of AD, encompassing its complex etiology, evolving diagnostic criteria, and the latest developments in therapeutic strategies. We delve into the key pathological hallmarks, including amyloid-beta plaques and neurofibrillary tangles, and explore the intricate interplay of genetic and environmental risk factors. Furthermore, we critically analyze the current state of diagnostic modalities, highlighting the potential of novel biomarkers and neuroimaging techniques for early and accurate detection. Finally, we discuss emerging therapeutic approaches, ranging from immunotherapies targeting amyloid-beta to innovative strategies aimed at modulating tau pathology and neuroinflammation, while considering the role of lifestyle interventions in mitigating AD risk and progression. We also examine the potential of artificial intelligence (AI) powered methods to enhance the diagnosis and treatment of this disease.
Many thanks to our sponsor Esdebe who helped us prepare this research report.
1. Introduction
Alzheimer’s disease (AD) is the most prevalent form of dementia, a neurodegenerative disorder that progressively impairs cognitive function, ultimately leading to significant functional decline and dependence. The global burden of AD is substantial and continues to rise with the aging population, posing significant social and economic challenges. Understanding the complexities of AD pathogenesis is crucial for developing effective prevention and treatment strategies. This review aims to provide a comprehensive overview of AD, from its underlying pathology to current diagnostic and therapeutic approaches, as well as future directions in the field. Given the multi-faceted nature of this disease, it is important to consider a wide range of potential therapeutic interventions from lifestyle changes, such as physical activity, to emerging pharmaceutical treatments.
Many thanks to our sponsor Esdebe who helped us prepare this research report.
2. Pathophysiology of Alzheimer’s Disease
The pathophysiology of AD is characterized by a complex interplay of molecular and cellular events that ultimately lead to neuronal dysfunction and death. The two primary pathological hallmarks of AD are:
- Amyloid-beta (Aβ) plaques: These extracellular deposits are composed primarily of Aβ peptides, which are derived from the amyloid precursor protein (APP) through sequential cleavage by β-secretase (BACE1) and γ-secretase. The accumulation of Aβ, particularly the Aβ42 isoform, leads to the formation of oligomers, fibrils, and ultimately, plaques. The amyloid cascade hypothesis posits that Aβ accumulation is the initiating event in AD pathogenesis, triggering downstream events such as tau hyperphosphorylation and neuroinflammation. While widely accepted, recent evidence suggests that the oligomeric forms of Aβ, rather than the plaques themselves, are the primary drivers of neurotoxicity.
- Neurofibrillary tangles (NFTs): These intracellular aggregates are composed of hyperphosphorylated tau protein. In healthy neurons, tau protein stabilizes microtubules, which are essential for axonal transport. In AD, tau becomes abnormally phosphorylated, leading to its detachment from microtubules and self-aggregation into paired helical filaments (PHFs), which then accumulate as NFTs. The presence of NFTs correlates strongly with cognitive decline and neuronal loss.
Beyond Aβ and tau, other pathological processes contribute to AD pathogenesis, including:
- Neuroinflammation: Chronic inflammation in the brain is a prominent feature of AD. Activated microglia and astrocytes release pro-inflammatory cytokines and chemokines, which contribute to neuronal damage and exacerbate Aβ and tau pathology. The role of neuroinflammation is complex, as it can also be neuroprotective in certain contexts by clearing Aβ and debris. However, chronic and dysregulated inflammation is detrimental to neuronal function.
- Synaptic dysfunction: Synaptic loss is a critical event in AD, occurring early in the disease process and correlating strongly with cognitive decline. Aβ oligomers and tau pathology impair synaptic plasticity, disrupt neurotransmitter signaling, and ultimately lead to synaptic degeneration.
- Oxidative stress: Increased oxidative stress, resulting from an imbalance between the production and removal of reactive oxygen species (ROS), contributes to neuronal damage and dysfunction in AD. Oxidative stress can damage proteins, lipids, and DNA, further exacerbating the pathological cascade.
- Mitochondrial dysfunction: Mitochondria are essential for cellular energy production and play a critical role in neuronal function. Mitochondrial dysfunction, including impaired oxidative phosphorylation and increased ROS production, is observed in AD and contributes to neuronal vulnerability.
- Vascular factors: Cerebrovascular dysfunction, including reduced cerebral blood flow and impaired blood-brain barrier integrity, can contribute to AD pathogenesis. Vascular risk factors such as hypertension, diabetes, and hyperlipidemia are associated with an increased risk of AD. The link between cardiovascular health and Alzheimer’s risk is increasingly recognized. Subtle changes in heart rate variability or cardiac function, potentially detectable through AI analysis of ECGs, could offer early indicators of AD.
The interplay of these pathological processes creates a vicious cycle of neurodegeneration, ultimately leading to the clinical manifestations of AD.
Many thanks to our sponsor Esdebe who helped us prepare this research report.
3. Genetic and Environmental Risk Factors
AD is a complex disease with both genetic and environmental risk factors. The most significant genetic risk factor for late-onset AD is the apolipoprotein E (APOE) ε4 allele. APOE is a cholesterol transport protein that plays a role in Aβ clearance and neuronal repair. Individuals carrying the APOE ε4 allele have an increased risk of developing AD and tend to have an earlier onset of the disease. The APOE ε2 allele, on the other hand, is associated with a reduced risk of AD.
Rare genetic mutations in genes encoding APP, presenilin 1 (PSEN1), and presenilin 2 (PSEN2) are responsible for early-onset familial AD (EOAD), which typically occurs before the age of 65. These mutations lead to increased production of Aβ42, promoting Aβ aggregation and plaque formation.
In addition to genetic factors, several environmental risk factors have been implicated in AD, including:
- Age: Age is the strongest risk factor for AD. The prevalence of AD increases exponentially with age.
- Family history: Individuals with a family history of AD are at a higher risk of developing the disease.
- Cardiovascular risk factors: Hypertension, diabetes, hyperlipidemia, obesity, and smoking are associated with an increased risk of AD. Maintaining cardiovascular health through lifestyle modifications and medical management can help reduce AD risk. As mentioned earlier, the potential of AI-powered ECG analysis to detect subtle cardiovascular changes linked to AD could prove valuable.
- Traumatic brain injury (TBI): A history of TBI, particularly repeated TBIs, is associated with an increased risk of AD.
- Education: Higher levels of education are associated with a reduced risk of AD. This may be due to increased cognitive reserve, which refers to the brain’s ability to cope with damage and maintain function.
- Lifestyle factors: Physical inactivity, poor diet, social isolation, and lack of cognitive stimulation may increase the risk of AD. Engaging in regular physical activity, maintaining a healthy diet, staying socially connected, and participating in mentally stimulating activities may help reduce AD risk.
The interplay of genetic and environmental factors determines an individual’s risk of developing AD. While genetic predisposition cannot be modified, lifestyle interventions targeting modifiable risk factors can play a crucial role in preventing or delaying the onset of the disease.
Many thanks to our sponsor Esdebe who helped us prepare this research report.
4. Diagnostic Methods
Accurate and early diagnosis of AD is essential for providing appropriate care and potentially intervening with disease-modifying therapies. The diagnosis of AD is based on a combination of clinical assessment, neuropsychological testing, and biomarker analysis.
- Clinical assessment: A thorough medical history and physical examination are essential to rule out other potential causes of cognitive impairment. Cognitive assessments, such as the Mini-Mental State Examination (MMSE) and the Montreal Cognitive Assessment (MoCA), are used to evaluate cognitive function. The Clinical Dementia Rating (CDR) scale is used to assess the severity of dementia.
- Neuropsychological testing: Comprehensive neuropsychological testing provides a detailed assessment of cognitive domains, including memory, language, executive function, and visuospatial abilities. This testing can help identify specific cognitive deficits and track changes in cognitive function over time.
- Biomarker analysis: Biomarkers are increasingly used to support the diagnosis of AD and to identify individuals at risk of developing the disease. The two main types of AD biomarkers are:
- Cerebrospinal fluid (CSF) biomarkers: CSF biomarkers include Aβ42, total tau (t-tau), and phosphorylated tau (p-tau). In AD, Aβ42 levels are typically decreased in CSF due to its deposition in plaques, while t-tau and p-tau levels are increased due to neuronal damage and tau pathology.
- Amyloid and tau PET imaging: Positron emission tomography (PET) imaging with radioligands that bind to Aβ plaques and NFTs allows for the visualization and quantification of these pathological hallmarks in the brain. Amyloid PET imaging can detect Aβ plaques even before the onset of clinical symptoms, while tau PET imaging correlates more closely with cognitive decline.
Newer diagnostic techniques include:
- Blood-based biomarkers: Recent advances have led to the development of blood-based biomarkers for AD, which are less invasive and more accessible than CSF biomarkers and PET imaging. Several blood-based biomarkers, including plasma Aβ42/Aβ40 ratio, p-tau181, and neurofilament light chain (NfL), have shown promise in detecting AD pathology and predicting cognitive decline. Further validation and standardization are needed before these biomarkers can be widely used in clinical practice.
- MRI: Magnetic resonance imaging (MRI) techniques can identify structural changes in the brain, such as hippocampal atrophy and cortical thinning, which are characteristic of AD. MRI can also be used to assess white matter integrity and cerebral blood flow.
The use of biomarkers in AD diagnosis has led to the development of new diagnostic criteria, such as the National Institute on Aging-Alzheimer’s Association (NIA-AA) research framework, which defines AD based on the presence of Aβ plaques and tau pathology, regardless of clinical symptoms. This framework allows for the identification of individuals in the preclinical stages of AD, who may be at risk of developing clinical symptoms in the future. The use of AI to analyze neuroimaging data has significantly improved the speed and accuracy of detection of AD.
Many thanks to our sponsor Esdebe who helped us prepare this research report.
5. Current and Emerging Therapeutic Strategies
Despite significant advances in understanding the pathophysiology of AD, effective disease-modifying therapies remain elusive. Current treatments for AD primarily focus on symptomatic relief, rather than addressing the underlying disease process.
- Cholinesterase inhibitors: These drugs, such as donepezil, rivastigmine, and galantamine, increase the levels of acetylcholine in the brain by inhibiting the enzyme cholinesterase, which breaks down acetylcholine. Cholinesterase inhibitors can improve cognitive function and reduce behavioral symptoms in some individuals with AD, but their effects are modest and temporary.
- Memantine: This drug is an NMDA receptor antagonist that is thought to protect neurons from excitotoxicity caused by excessive glutamate stimulation. Memantine can improve cognitive function and reduce behavioral symptoms in some individuals with moderate to severe AD.
Emerging therapeutic strategies for AD target the underlying pathological processes, including Aβ and tau pathology, neuroinflammation, and synaptic dysfunction. Some of the most promising approaches include:
- Immunotherapies targeting Aβ: These therapies involve the use of antibodies to clear Aβ plaques from the brain. Several anti-Aβ antibodies, such as aducanumab, lecanemab and donanemab, have shown promise in clinical trials, demonstrating a reduction in Aβ plaques and a slowing of cognitive decline in some individuals with early AD. However, these therapies are associated with side effects, such as amyloid-related imaging abnormalities (ARIA), which can cause brain swelling or bleeding. Lecanemab and Donanemab have shown some efficacy and been approved by the FDA.
- BACE inhibitors: These drugs inhibit the enzyme BACE1, which is involved in the production of Aβ. Several BACE inhibitors have been tested in clinical trials, but most have failed due to lack of efficacy or adverse effects.
- Tau-targeted therapies: These therapies aim to reduce tau pathology by inhibiting tau phosphorylation, preventing tau aggregation, or clearing tau aggregates from the brain. Several tau-targeted therapies are currently being tested in clinical trials.
- Anti-inflammatory therapies: These therapies aim to reduce neuroinflammation by targeting inflammatory cytokines or immune cells. Several anti-inflammatory drugs are being tested in clinical trials.
- Synaptic therapies: These therapies aim to protect and restore synaptic function by enhancing neurotransmitter signaling, promoting synaptic plasticity, or preventing synaptic degeneration.
In addition to pharmacological therapies, lifestyle interventions can play a crucial role in mitigating AD risk and progression. These interventions include:
- Physical activity: Regular physical activity has been shown to improve cognitive function and reduce the risk of AD.
- Healthy diet: A Mediterranean-style diet, rich in fruits, vegetables, whole grains, and healthy fats, has been associated with a reduced risk of AD.
- Cognitive stimulation: Engaging in mentally stimulating activities, such as reading, puzzles, and social interactions, can help maintain cognitive function.
- Social engagement: Maintaining social connections and participating in social activities can help reduce the risk of AD.
Combination therapies, targeting multiple pathological processes and incorporating lifestyle interventions, may be the most effective approach to treating AD. It is important to note that early detection, possibly improved by AI-driven methods, is crucial to enhancing the effect of therapeutic approaches.
Many thanks to our sponsor Esdebe who helped us prepare this research report.
6. The Potential of AI in Alzheimer’s Disease Research and Treatment
Artificial intelligence (AI) is revolutionizing various fields of medicine, and Alzheimer’s disease research is no exception. AI offers a powerful toolkit for addressing the complexities of AD, from early diagnosis to personalized treatment strategies.
- Enhanced Diagnosis: AI algorithms can analyze vast amounts of data from various sources, including neuroimaging scans (MRI, PET), genetic data, clinical records, and cognitive assessments, to identify subtle patterns and biomarkers that may be indicative of early AD. For instance, deep learning models can be trained to detect subtle changes in brain structure or activity on MRI scans that are not readily apparent to the human eye. As highlighted in the introduction, AI-powered ECG analysis could detect early cardiovascular indicators associated with AD.
- Drug Discovery and Development: AI can accelerate the drug discovery process by identifying potential drug targets, predicting drug efficacy, and optimizing clinical trial design. Machine learning algorithms can analyze large datasets of molecular structures and biological activity to identify promising drug candidates that are more likely to succeed in clinical trials.
- Personalized Treatment: AI can help tailor treatment strategies to individual patients based on their unique genetic profiles, medical history, and lifestyle factors. By analyzing these data, AI algorithms can predict an individual’s response to different treatments and recommend the most effective course of action.
- Predictive Modeling: AI can develop predictive models to estimate an individual’s risk of developing AD based on their genetic and lifestyle factors. These models can help identify individuals who are at high risk of developing AD, allowing for early interventions to delay or prevent the onset of the disease.
- Care Optimization: AI-powered tools can assist in providing personalized care for individuals with AD. For example, AI-powered robots can provide companionship, reminders, and assistance with daily tasks. AI-driven sensors can monitor patients’ movements and vital signs to detect falls or other health emergencies.
AI’s role in Alzheimer’s disease research and treatment is still evolving, but its potential to transform the field is immense. As AI technology continues to advance, it is likely to play an increasingly important role in the fight against this devastating disease.
Many thanks to our sponsor Esdebe who helped us prepare this research report.
7. Future Directions and Challenges
Despite significant progress in understanding AD, many challenges remain. Future research efforts should focus on:
- Identifying novel drug targets: A better understanding of the underlying pathological processes is needed to identify new drug targets that can effectively modify the disease course.
- Developing more effective therapies: Current therapies have limited efficacy and are associated with side effects. The development of more effective and well-tolerated therapies is a major priority.
- Improving early detection: Early detection of AD is crucial for maximizing the benefits of therapeutic interventions. Further research is needed to develop more sensitive and specific biomarkers for early detection.
- Understanding the role of genetics and environment: Further research is needed to elucidate the complex interplay of genetic and environmental factors in AD pathogenesis.
- Developing personalized treatment strategies: Tailoring treatment strategies to individual patients based on their unique characteristics may improve treatment outcomes.
- Addressing health disparities: AD disproportionately affects certain populations, such as African Americans and Hispanics. Research is needed to understand the factors contributing to these disparities and to develop interventions to address them.
- Improving care for people living with AD: Comprehensive care, including medical, social, and emotional support, is essential for people living with AD and their caregivers. Further research is needed to develop and implement effective care models.
The development of effective disease-modifying therapies for AD remains a significant challenge. However, with continued research efforts and a collaborative approach, progress is being made towards the goal of preventing and treating this devastating disease.
Many thanks to our sponsor Esdebe who helped us prepare this research report.
8. Conclusion
Alzheimer’s disease is a complex and devastating neurodegenerative disorder that poses a significant challenge to global health. While significant progress has been made in understanding the underlying pathophysiology of AD, effective disease-modifying therapies remain elusive. This review has provided a comprehensive overview of AD, encompassing its etiology, pathology, diagnosis, and treatment. Emerging therapeutic strategies targeting Aβ and tau pathology, neuroinflammation, and synaptic dysfunction hold promise for the future. The integration of AI technologies offers the potential to revolutionize diagnosis, drug discovery, and personalized treatment approaches. Early detection and intervention are crucial for maximizing the benefits of therapeutic interventions. Future research efforts should focus on identifying novel drug targets, developing more effective therapies, improving early detection, understanding the role of genetics and environment, developing personalized treatment strategies, and addressing health disparities. Only through a concerted and collaborative effort can we hope to conquer this formidable disease.
Many thanks to our sponsor Esdebe who helped us prepare this research report.
References
- Alzheimer’s Association. (2023). 2023 Alzheimer’s Disease Facts and Figures. Alzheimer’s & Dementia, 19(4), 1598-1695.
- Bloom, G. S. (2014). Amyloid-β and tau: the trigger and bullet in Alzheimer disease pathogenesis. JAMA neurology, 71(4), 505-508.
- Hardy, J. A., & Higgins, G. A. (1992). Alzheimer’s disease: the amyloid cascade hypothesis. Science, 256(5054), 184-185.
- De Strooper, B., & Karran, E. (2016). The cellular phase of Alzheimer’s disease. Cell, 164(4), 603-615.
- Goedert, M., Eisenberg, D., & Crowther, R. A. (2017). Propagation of prions and prion-like proteins in neurodegenerative diseases. Annual review of neuroscience, 40, 63-83.
- Heneka, M. T., Carson, M. J., Khoury, J. E., Landreth, G. E., Brosseron, F., Feinstein, D. L., … & Kummer, M. P. (2015). Neuroinflammation in Alzheimer’s disease. The Lancet Neurology, 14(4), 388-405.
- Selkoe, D. J. (2002). Alzheimer’s disease is a synaptic failure. Science, 298(5594), 789-791.
- Butterfield, D. A., & Halliwell, B. (2019). Oxidative stress, dysfunctional glucose metabolism and Alzheimer’s disease. Nature Reviews Neuroscience, 20(3), 148-167.
- Swerdlow, R. H., Burns, J. M., & Khan, S. M. (2014). The Alzheimer’s disease mitochondrial cascade hypothesis. Journal of Alzheimer’s Disease, 20(s2), S103-S116.
- de la Torre, J. C. (2004). Is Alzheimer’s disease a neurodegenerative disorder of the microcirculation?. Annals of the New York Academy of Sciences, 1019(1), 1-7.
- Strittmatter, W. J., Saunders, A. M., Schmechel, D., Pericak-Vance, M., Enghild, J., Salvesen, G. S., & Roses, A. D. (1993). Apolipoprotein E: high-avidity binding to beta-amyloid and increased frequency of type 4 allele in late-onset familial Alzheimer disease. Proceedings of the National Academy of Sciences, 90(5), 1977-1981.
- Goate, A., Chartier-Harlin, M. C., Mullan, M., Brown, J., Crawford, F., Fidani, L., … & Hardy, J. (1991). Segregation of a missense mutation in the amyloid precursor protein gene with familial Alzheimer’s disease. Nature, 349(6311), 704-706.
- Price, J. L., McKeel Jr, D. W., Buckles, V. D., Morris, J. C. (2009). Neuropathology of nondemented aging: Presumptive evidence for preclinical Alzheimer disease. Neurobiol Aging. 30(7): 1026–1036.
- Jack, C. R., Jr, Bennett, D. A., Blennow, K., Carrillo, M. C., Dunn, B., Haeberlein, S. B., Holtzman, D. M., Jagust, W., Jessen, F., Karlawish, J., Liu, E., Molinuevo, J. L., Montine, T. J., Phelps, C. H., Rankin, K. P., Rowe, C. C., Scheltens, P., Siemers, E., Strooper, B. D., Trojanowski, J. Q., & the NIA-AA Research Framework. (2018). A research framework for Alzheimer’s disease: Integrating new biological findings into an updated definition of AD. Alzheimer’s & Dementia, 14(4), 535–562.
- Cummings, J. L., Morstorf, J., & Zhong, K. (2014). Alzheimer’s disease drug-development pipeline: few candidates, frequent failures. Alzheimer’s research & therapy, 6(4), 37.
- van Dyck, C. H., Swanson, C. J., Aisen, P., Bateman, R. J., Chen, C., Gee, M., Kanekiyo, M., Li, D., Veitch, S., Gauthier, S., Katayama, S., Essioux, L., Barakos, J., Henley, D., Frost, J., Raghavan, N., Budd, H. A., Koyama, A., Kramer, L. D., & Pfeifer, L. A. (2023). Lecanemab in Early Alzheimer’s Disease. New England Journal of Medicine, 388(1), 9-21.
- Swanson CJ, et al. Donanemab in Early Symptomatic Alzheimer’s Disease. N Engl J Med. 2023;388:48-61.
- Long, J. M., & Holtzman, D. M. (2011). Alzheimer disease: an update on pathobiology and treatment strategies. Cell, 147(4), 759-769.
- Livingston, G., Sommerlad, A., Orgeta, V., Costafreda, S. G., Huntley, J., Ames, D., … & Cooper, C. (2020). Dementia prevention, intervention, and care: 2020 report of the Lancet Commission. The Lancet, 396(10248), 413-446.
- Hampel H, Vergallo A, Siniatchkin M, et al. Artificial intelligence in Alzheimer’s disease: state-of-the-art and future directions. Nat Rev Neurol. 2023;19(4):195-208.
- Costa AS, Serrano R, Duarte-Silva S, Alves V, Santana I. Artificial Intelligence in Alzheimer’s Disease: A Systematic Review. Front Neurosci. 2020;14:562.
So, if AI can detect AD via ECG, does that mean my smartwatch is now qualified to give me unsolicited medical advice *and* nag me about exercise? I’m simultaneously impressed and terrified! Will I need to start arguing with my wrist?
That’s a really interesting point! The prospect of proactive health monitoring through smartwatches certainly raises questions about autonomy and unsolicited advice. Maybe future devices will offer adjustable sensitivity settings, allowing users to define their comfort level with health alerts. This could balance early detection with personal preferences.
Editor: MedTechNews.Uk
Thank you to our Sponsor Esdebe
The review highlights the potential of AI in analysing neuroimaging data. Could AI also play a role in integrating diverse data types, such as genetic information, lifestyle factors, and cognitive test results, to create more holistic and personalized risk assessments for Alzheimer’s?
That’s an excellent point! AI’s ability to integrate diverse data streams is incredibly promising. Combining neuroimaging with genetic predispositions, lifestyle factors, and cognitive performance could revolutionize personalized risk assessment and treatment strategies for Alzheimer’s. It would be fascinating to see more research in this area!
Editor: MedTechNews.Uk
Thank you to our Sponsor Esdebe
The review mentions AI’s potential in enhancing AD diagnosis through neuroimaging analysis. Could AI also be leveraged to predict individual cognitive decline trajectories based on longitudinal data, informing more targeted interventions?
That’s a great question! Exploring individual cognitive decline trajectories using AI and longitudinal data is crucial. This could allow for personalized interventions based on predicted needs, maximizing treatment effectiveness and potentially slowing down the progression of Alzheimer’s. Continuous monitoring and adaptation of care plans is definitely an area ripe for exploration!
Editor: MedTechNews.Uk
Thank you to our Sponsor Esdebe