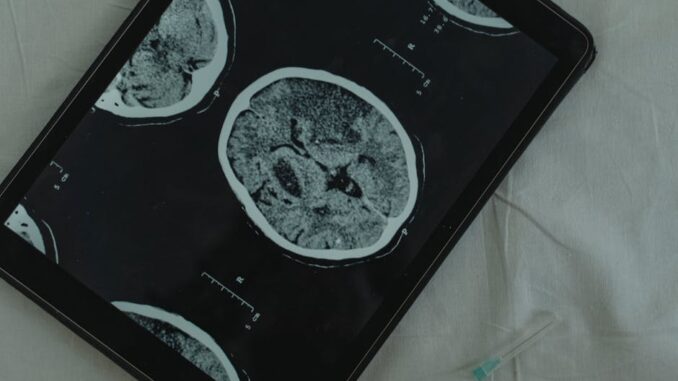
Abstract
Spinal Cord Stimulation (SCS), initially developed for pain management, is experiencing a renaissance as research unveils its broader therapeutic potential. While its efficacy in treating chronic pain, particularly neuropathic pain, is well-established, emerging evidence suggests SCS’s applicability extends beyond analgesia to include motor rehabilitation, cardiovascular regulation, and even modulation of specific neurological disorders. This research report examines the evolving role of SCS, moving beyond its traditional confines. We delve into the mechanisms underpinning SCS’s expanded therapeutic actions, discuss technological advancements enabling more targeted and personalized stimulation, explore novel applications beyond pain, and analyze the challenges and future directions of this dynamic field. We critically evaluate the existing literature, highlight areas where further research is needed, and offer perspectives on optimizing SCS for a wider range of clinical indications.
Many thanks to our sponsor Esdebe who helped us prepare this research report.
1. Introduction: SCS – From Pain Alleviation to Neuromodulation Hub
Spinal Cord Stimulation (SCS) has been a cornerstone in the treatment of chronic pain for decades, offering a viable alternative for patients who have not responded adequately to conservative therapies and/or surgical interventions. The initial gate control theory of pain, proposed by Melzack and Wall (1965), provided the theoretical framework for SCS, positing that stimulation of large-diameter afferent fibers in the dorsal column could inhibit the transmission of nociceptive signals from smaller-diameter fibers. Early SCS systems, therefore, focused on modulating sensory perception to mask pain. However, our understanding of SCS’s mechanisms of action has evolved significantly, recognizing its influence on various neurophysiological processes beyond simple gate control.
The contemporary view acknowledges that SCS exerts its effects through a complex interplay of mechanisms. These include, but are not limited to: modulation of dorsal horn neuronal activity, release of endogenous opioids, alterations in neuroinflammation, and effects on supraspinal structures, including the brainstem and cortex (Linderoth & Foreman, 2015). Consequently, SCS’s therapeutic potential extends beyond mere pain suppression.
This report will explore the expansion of SCS from its traditional role in pain management to a broader platform for neuromodulation. This includes an examination of emerging applications, such as motor rehabilitation post-stroke or spinal cord injury (SCI), management of cardiovascular diseases, and potential treatment of neurological disorders like Parkinson’s disease. The exploration will also include critical discussion on technological advances in device design, waveform delivery, and closed-loop systems that enable more targeted and personalized neuromodulation. Further, we discuss the challenges and ethical considerations associated with expanding the use of SCS and outlining future research directions required to optimize its therapeutic benefits.
Many thanks to our sponsor Esdebe who helped us prepare this research report.
2. Mechanisms of Action: Unveiling the Multifaceted Effects of SCS
The efficacy of SCS in pain management is partially attributed to its influence on spinal cord circuitry. Specifically, SCS can modulate the activity of wide dynamic range (WDR) neurons in the dorsal horn, which play a critical role in transmitting nociceptive information to the brain. By activating inhibitory interneurons and modulating synaptic transmission, SCS can reduce the excitability of WDR neurons and decrease the perception of pain (Song et al., 2009). Moreover, SCS has been shown to promote the release of endogenous opioids, such as enkephalins and endorphins, which act as natural analgesics and further contribute to pain relief (Stanton-Hicks et al., 1996).
Beyond its effects on spinal cord circuitry, SCS also influences supraspinal structures. Studies have shown that SCS can modulate brain activity in regions involved in pain processing, such as the anterior cingulate cortex (ACC), insula, and somatosensory cortex (Garcia-Larrea et al., 1999). These changes in brain activity may contribute to the therapeutic effects of SCS by altering pain perception, emotional responses to pain, and cognitive processing of pain-related information. Furthermore, SCS can influence the autonomic nervous system, potentially through modulation of descending pathways from the brainstem. This can lead to alterations in cardiovascular function, such as reduced blood pressure and heart rate, which may be beneficial in certain clinical conditions.
The specific mechanisms of action of SCS can vary depending on the stimulation parameters used, such as frequency, pulse width, and amplitude. For example, high-frequency SCS (HF10 SCS) has been shown to be more effective than traditional low-frequency SCS in reducing pain in some patients (Kapural et al., 2015). HF10 SCS is thought to work by inhibiting neuronal activity through a different mechanism than low-frequency SCS, potentially involving the activation of GABAergic interneurons (Oakley & Prager, 2012). These differences in mechanism of action highlight the importance of tailoring SCS parameters to the individual patient and clinical condition. It is also important to consider that the interplay of these mechanisms is complex and that a deeper understanding of their relative contributions is required for optimizing therapeutic outcomes. In particular, better understanding of the chronic effects of SCS on neural plasticity is needed.
Many thanks to our sponsor Esdebe who helped us prepare this research report.
3. Technological Advancements in SCS: Paving the Way for Personalized Neuromodulation
The evolution of SCS technology has been rapid, leading to significant improvements in device design, waveform delivery, and programming capabilities. Early SCS systems used simple on/off stimulation with fixed parameters. Modern SCS devices offer a wider range of stimulation parameters, including adjustable frequency, pulse width, amplitude, and polarity. This allows for more precise targeting of specific neural circuits and tailoring of stimulation to individual patient needs.
One significant advancement is the development of lead designs that provide more targeted stimulation. Traditional paddle leads deliver stimulation to a broad area of the spinal cord. Newer lead designs, such as multi-column and segmented leads, allow for more focused stimulation of specific dorsal column fibers (Deer et al., 2017). This can improve the effectiveness of SCS while minimizing unwanted side effects. Another important technological advancement is the development of rechargeable SCS systems. Rechargeable systems eliminate the need for battery replacement surgeries, which can reduce the risk of complications and improve patient satisfaction.
Closed-loop SCS systems represent a promising area of research. These systems use real-time feedback from neural sensors to automatically adjust stimulation parameters based on patient activity and pain levels. Closed-loop systems have the potential to optimize SCS therapy by providing stimulation that is more responsive to individual patient needs and by minimizing the risk of overstimulation or understimulation. Development of reliable and biocompatible neural sensors remains a challenge, but the potential benefits of closed-loop SCS are significant. Furthermore, the integration of artificial intelligence (AI) and machine learning algorithms into SCS systems could further enhance their personalization and efficacy. AI-powered systems could analyze patient data to predict optimal stimulation parameters, detect patterns of pain, and provide personalized recommendations for SCS therapy.
Many thanks to our sponsor Esdebe who helped us prepare this research report.
4. Expanding the Therapeutic Scope: Novel Applications Beyond Pain Management
While SCS is primarily used for pain management, emerging evidence suggests that it may have therapeutic potential in other neurological and cardiovascular conditions. One area of growing interest is the use of SCS for motor rehabilitation after stroke or spinal cord injury (SCI). Studies have shown that SCS can improve motor function in patients with incomplete SCI by enhancing neuronal excitability and promoting neuroplasticity (Gad et al., 2019). SCS may also improve gait and balance in patients with stroke by modulating motor pathways in the brain and spinal cord.
Another potential application of SCS is in the management of cardiovascular diseases. SCS has been shown to reduce angina pectoris (chest pain) in patients with refractory coronary artery disease (Mannheimer et al., 1998). The mechanisms underlying this effect are not fully understood, but may involve modulation of autonomic nervous system activity and reduction of myocardial ischemia. SCS has also been investigated as a potential treatment for heart failure, with some studies suggesting that it may improve cardiac function and reduce symptoms (Borggrefe et al., 2016). These cardiovascular applications of SCS are still under investigation and require further research.
In addition, SCS has shown promise in treating other neurological conditions, such as Parkinson’s disease and epilepsy. Studies have suggested that SCS may improve motor symptoms in patients with Parkinson’s disease, such as rigidity and bradykinesia (slow movement) (Frazzini et al., 2009). SCS may also reduce the frequency of seizures in patients with refractory epilepsy (Van Buyten et al., 2011). While these findings are preliminary, they suggest that SCS may have a broader role in the treatment of neurological disorders. The use of SCS in neurological disorders is still largely experimental and requires rigorous clinical trials to establish its efficacy and safety.
Many thanks to our sponsor Esdebe who helped us prepare this research report.
5. Challenges and Ethical Considerations: Navigating the Future of SCS
Despite the advances in SCS technology and its expanding therapeutic scope, several challenges and ethical considerations must be addressed to ensure its safe and effective use. One challenge is the lack of standardized protocols for patient selection and outcome assessment. Different centers use different criteria for selecting patients for SCS, which can lead to variability in treatment outcomes. Similarly, there is a lack of consensus on the best way to measure the effectiveness of SCS, which can make it difficult to compare results across studies.
Another challenge is the risk of complications associated with SCS implantation and maintenance. Complications can include infection, lead migration, hardware failure, and pain at the implantation site. These complications can require additional surgeries and can negatively impact patient quality of life. Careful patient selection, meticulous surgical technique, and proper device maintenance are essential to minimize the risk of complications.
Ethical considerations related to SCS include the potential for overuse, the cost of the technology, and the impact on patient autonomy. There is a risk that SCS may be used inappropriately in patients who are not good candidates for the therapy, or that it may be used as a substitute for other more conservative treatments. The cost of SCS can be a barrier to access for some patients, particularly in countries with limited healthcare resources. Finally, the use of SCS can raise questions about patient autonomy, particularly in the context of closed-loop systems that automatically adjust stimulation parameters without patient input. It is important to ensure that patients are fully informed about the risks and benefits of SCS and that they have control over their own treatment.
The potential for ‘off-label’ use of SCS also raises ethical concerns. As the potential applications of SCS expand beyond pain management, it is important to ensure that these applications are supported by rigorous scientific evidence and that patients are fully informed about the experimental nature of the treatment. Careful consideration of ethical issues is essential to ensure that SCS is used responsibly and ethically.
Many thanks to our sponsor Esdebe who helped us prepare this research report.
6. Future Directions: Charting a Course for Enhanced SCS Therapy
The future of SCS lies in further refining the technology, expanding its applications, and addressing the challenges and ethical considerations associated with its use. Future research should focus on developing more sophisticated stimulation paradigms that can target specific neural circuits with greater precision. This could involve the use of novel waveforms, such as burst stimulation or patterned stimulation, and the development of new lead designs that allow for more focused stimulation. Improved understanding of the mechanisms of action is crucial for the development of new stimulation paradigms.
Another area of research should focus on developing closed-loop SCS systems that can automatically adjust stimulation parameters based on real-time feedback from neural sensors. This could involve the use of electrophysiological measures, such as local field potentials (LFPs), or biochemical markers to guide stimulation. Closed-loop systems have the potential to optimize SCS therapy by providing stimulation that is more responsive to individual patient needs and by minimizing the risk of overstimulation or understimulation. Furthermore, research is needed to identify biomarkers that can predict which patients are most likely to benefit from SCS and to optimize patient selection criteria.
Finally, more research is needed to evaluate the long-term outcomes of SCS and to identify strategies for managing complications. This could involve the development of new surgical techniques, improved device designs, and better patient education programs. Addressing the ethical considerations associated with SCS is also essential to ensure that it is used responsibly and ethically. This could involve the development of guidelines for patient selection, informed consent, and data privacy. In addition, the development of affordable SCS technologies could improve access to treatment for patients in low-resource settings.
Many thanks to our sponsor Esdebe who helped us prepare this research report.
7. Conclusion
Spinal Cord Stimulation has transcended its original purpose as a pain management tool, evolving into a versatile neuromodulation platform with potential applications across a spectrum of neurological and cardiovascular disorders. Technological advancements, particularly in lead design, waveform delivery, and closed-loop systems, are enabling more targeted and personalized stimulation strategies. As we delve deeper into the mechanisms of action, including spinal and supraspinal effects, we are gaining insights that pave the way for optimized therapies.
However, the expansion of SCS necessitates careful consideration of challenges and ethical implications. Standardizing patient selection, mitigating complications, and addressing concerns surrounding overuse and cost are critical to ensuring the responsible and equitable application of this technology. The future of SCS hinges on continued research, technological innovation, and a commitment to ethical principles, ultimately aiming to unlock its full potential to improve the lives of individuals affected by a wide range of debilitating conditions.
Many thanks to our sponsor Esdebe who helped us prepare this research report.
References
Borggrefe, M., Lurz, P., Gonska, B., Utech, A. T., Volkmer, M., Lennerz, C., … & Butter, C. (2016). Randomized trial of spinal cord stimulation in patients with chronic refractory heart failure. European Journal of Heart Failure, 18(7), 851-859.
Deer, T. R., Hayek, S. M., Narouze, S., Fattouh, M., Wallace, M. S., Webster, L., … & Mekhail, N. (2017). The appropriate use of neurostimulation: spinal cord stimulation and peripheral nerve stimulation for chronic pain. Neuromodulation: Technology at the Neural Interface, 20(6), 559-574.
Frazzini, V., Galati, S., Marchese, R., Barone, P., & Fraioli, L. (2009). Motor effects of spinal cord stimulation in Parkinson’s disease. European Journal of Neurology, 16(1), 81-87.
Gad, P., Kumar, V., Isaacs, R., & Hsieh, J. T. (2019). Spinal cord stimulation for rehabilitation of motor function after spinal cord injury: a systematic review. Neuromodulation: Technology at the Neural Interface, 22(6), 639-648.
Garcia-Larrea, L., Peyron, R., Mertens, P., Gregoire, M. C., Lavenne, F., Bonnefoi, F., … & Mauguière, F. (1999). Electrical stimulation of motor cortex for pain control: a combined PET and TMS study. Pain, 83(3), 259-273.
Kapural, L., Yu, C., Doust, M. W., Gliner, B. E., Vallejo, R., Sitzman, B. T., … & Liss, J. M. (2015). Novel 10-kHz high-frequency therapy is superior to traditional low-frequency spinal cord stimulation for the treatment of chronic back and leg pain: the SENZA-RCT randomized controlled trial. Anesthesiology, 123(4), 851-860.
Linderoth, B., & Foreman, R. D. (2015). Mechanisms of spinal cord stimulation in chronic pain: a review and update. Neuromodulation: Technology at the Neural Interface, 18(4), 313-328.
Mannheimer, C., Eliasson, T., Augustinsson, L. E., Blomstrand, C., Emanuelsson, H., Waagstein, F., & Andersson, B. E. (1998). Electrical stimulation in the treatment of severe angina pectoris: clinical experience over 5 years. Coronary Artery Disease, 9(3-4), 195-202.
Melzack, R., & Wall, P. D. (1965). Pain mechanisms: a new theory. Science, 150(3699), 971-979.
Oakley, J. C., & Prager, J. P. (2012). Spinal cord stimulation with burst technology. Pain Medicine, 13(8), 1047-1052.
Song, Z., Boersma, K., & Linderoth, B. (2009). Spinal cord stimulation: mechanisms of action. Pain, 147(1-3), 1-5.
Stanton-Hicks, M., Rezai, A. R., & Daves, J. E. (1996). Spinal cord stimulation for complex regional pain syndrome. Pain, 67(1), 133-146.
Van Buyten, J. P., Smet, I., Vanacker, P., & van de Kelft, E. (2011). Dorsal column stimulation for refractory epilepsy. Epilepsy & Behavior, 22(2), 368-371.
The exploration of closed-loop systems and AI integration for personalized SCS therapy is particularly exciting. How might these advancements incorporate patient-reported outcomes in real-time to further refine and optimize stimulation parameters?
That’s a great point! Integrating patient-reported outcomes in real-time could revolutionize SCS. Imagine AI algorithms learning from a patient’s daily pain scores and adjusting stimulation to preempt pain spikes, or adapt to changing activity levels. This proactive, personalized approach holds huge potential for improved efficacy and quality of life. It is an exciting development!
Editor: MedTechNews.Uk
Thank you to our Sponsor Esdebe
The discussion of expanding SCS beyond pain management to motor rehabilitation and cardiovascular applications is compelling. Exploring the specific stimulation parameters that optimize outcomes for these diverse conditions represents an important avenue for future research.