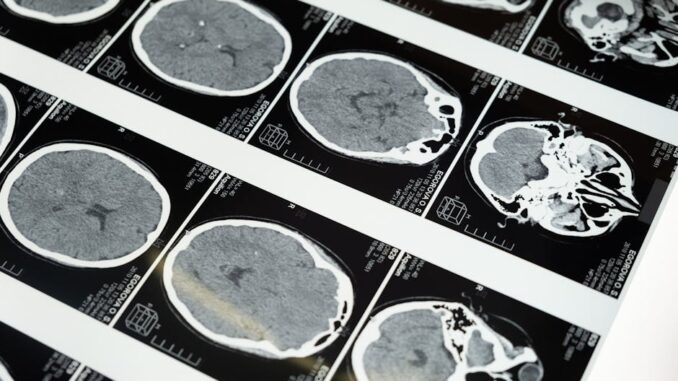
Abstract
Magnetic Resonance Imaging (MRI) has revolutionized the diagnosis and management of neurological disorders. Its non-invasive nature, superior soft tissue contrast, and versatility in imaging various tissue properties have made it an indispensable tool for clinicians and researchers alike. This research report provides an in-depth overview of advanced MRI techniques and their applications in a wide range of neurological conditions, including stroke, multiple sclerosis, neurodegenerative diseases, brain tumors, and spinal cord injuries. We discuss the principles and advantages of advanced techniques such as diffusion-weighted imaging (DWI), perfusion-weighted imaging (PWI), functional MRI (fMRI), magnetic resonance spectroscopy (MRS), and susceptibility-weighted imaging (SWI). Furthermore, we explore the challenges and future directions of MRI research, including the development of ultra-high field imaging, advanced contrast agents, and artificial intelligence (AI)-based image analysis. This report aims to provide an expert-level perspective on the current status and future potential of MRI in the field of neurology.
Many thanks to our sponsor Esdebe who helped us prepare this research report.
1. Introduction
Neurological disorders represent a significant global health burden, affecting millions of individuals worldwide. Accurate diagnosis and effective management of these conditions are crucial for improving patient outcomes and quality of life. Magnetic Resonance Imaging (MRI) has emerged as a cornerstone in the diagnostic armamentarium for neurological diseases, providing detailed anatomical and functional information about the brain and spinal cord. Unlike other imaging modalities such as X-ray computed tomography (CT), MRI does not involve ionizing radiation, making it a safer option for repeated imaging, particularly in vulnerable populations like children and pregnant women. Its superior soft tissue contrast allows for the visualization of subtle pathological changes that may be missed by other techniques.
Over the past few decades, significant advancements in MRI technology and imaging techniques have expanded its capabilities beyond simple anatomical imaging. These advancements include diffusion-weighted imaging (DWI), perfusion-weighted imaging (PWI), functional MRI (fMRI), magnetic resonance spectroscopy (MRS), and susceptibility-weighted imaging (SWI), which provide valuable insights into the microstructural, hemodynamic, metabolic, and functional aspects of the nervous system. This report will delve into the principles and applications of these advanced MRI techniques, highlighting their role in the diagnosis, monitoring, and understanding of various neurological disorders.
Many thanks to our sponsor Esdebe who helped us prepare this research report.
2. Advanced MRI Techniques
2.1 Diffusion-Weighted Imaging (DWI)
DWI is a powerful MRI technique that measures the random (Brownian) motion of water molecules in biological tissues. This motion, known as diffusion, is influenced by the tissue microstructure, including cell membranes, organelles, and macromolecules. In areas of restricted diffusion, such as in acute ischemic stroke, the diffusion of water molecules is impeded, resulting in a higher signal intensity on DWI images. This increased signal intensity is known as diffusion restriction and is a hallmark of cytotoxic edema, which occurs within minutes to hours after the onset of ischemia.
The most commonly used DWI sequence is echo-planar imaging (EPI), which allows for rapid acquisition of images with high sensitivity to diffusion. The strength and direction of the diffusion-sensitizing gradients are determined by the b-value. Higher b-values provide greater sensitivity to diffusion but also result in lower signal-to-noise ratio (SNR). The apparent diffusion coefficient (ADC) is a quantitative measure of diffusion that is calculated from DWI images acquired at multiple b-values. The ADC is decreased in areas of restricted diffusion and can be used to differentiate acute from chronic stroke, as well as to characterize other neurological conditions such as brain tumors and infections.
DWI has revolutionized the management of acute ischemic stroke by allowing for early detection of infarcted tissue and identification of patients who may benefit from thrombolytic therapy or mechanical thrombectomy. In addition, DWI can be used to assess the extent of white matter damage in multiple sclerosis, to differentiate between different types of brain tumors, and to diagnose herpes encephalitis.
2.2 Perfusion-Weighted Imaging (PWI)
PWI is an MRI technique that measures the blood flow and blood volume in the brain. It is based on the principle of injecting a paramagnetic contrast agent, such as gadolinium, into the bloodstream and monitoring its passage through the cerebral vasculature. The changes in signal intensity caused by the contrast agent are used to calculate various perfusion parameters, including cerebral blood flow (CBF), cerebral blood volume (CBV), mean transit time (MTT), and time to peak (TTP).
There are two main types of PWI techniques: dynamic susceptibility contrast (DSC) and arterial spin labeling (ASL). DSC-PWI is the most commonly used technique and involves rapid acquisition of T2*-weighted images during the bolus injection of contrast agent. ASL-PWI, on the other hand, does not require the injection of contrast agent and instead uses magnetically labeled arterial blood water as an endogenous tracer. ASL-PWI is particularly useful in patients with renal insufficiency or allergy to gadolinium-based contrast agents.
PWI is used in conjunction with DWI to identify the penumbral tissue in acute ischemic stroke, which is the region of potentially salvageable tissue surrounding the core infarct. The mismatch between the DWI lesion and the PWI lesion, known as the diffusion-perfusion mismatch, can be used to predict which patients will benefit from reperfusion therapies. PWI is also used to assess tumor angiogenesis and response to anti-angiogenic therapies in brain tumors.
2.3 Functional MRI (fMRI)
fMRI is a non-invasive neuroimaging technique that measures brain activity by detecting changes in blood flow. It is based on the principle that neural activity is coupled to cerebral blood flow, and that active brain regions require more oxygen and glucose. The most commonly used fMRI technique is blood-oxygen-level-dependent (BOLD) imaging, which relies on the difference in magnetic susceptibility between oxygenated and deoxygenated hemoglobin. When a brain region becomes active, the local concentration of deoxygenated hemoglobin decreases, resulting in an increase in the BOLD signal.
fMRI can be used to study a wide range of cognitive processes, including motor control, sensory perception, language, memory, and emotion. It can also be used to map brain function in patients with neurological disorders, such as stroke, epilepsy, and brain tumors. Pre-surgical fMRI mapping is particularly useful for identifying eloquent areas of the brain, such as motor and language cortex, to minimize the risk of neurological deficits during surgery.
2.4 Magnetic Resonance Spectroscopy (MRS)
MRS is a non-invasive technique that provides information about the biochemical composition of tissues. It is based on the principle that different molecules resonate at different frequencies in a magnetic field. By analyzing the spectrum of resonant frequencies, MRS can identify and quantify various metabolites in the brain, including N-acetylaspartate (NAA), creatine (Cr), choline (Cho), lactate (Lac), and myo-inositol (mI).
NAA is a marker of neuronal integrity and is typically decreased in areas of neuronal damage or loss. Cr is a marker of cellular energy metabolism and is relatively stable in most conditions. Cho is a marker of cell membrane turnover and is typically increased in areas of tumor growth or inflammation. Lac is a marker of anaerobic metabolism and is increased in areas of ischemia or hypoxia. mI is a marker of glial cell activity and is increased in areas of glial proliferation.
MRS is used to diagnose and monitor a variety of neurological disorders, including brain tumors, stroke, multiple sclerosis, and neurodegenerative diseases. It can also be used to differentiate between different types of brain tumors, to assess the response to treatment, and to predict prognosis.
2.5 Susceptibility-Weighted Imaging (SWI)
SWI is a high-resolution MRI technique that is highly sensitive to magnetic susceptibility differences between tissues. It is particularly useful for detecting blood products, iron deposition, and calcifications in the brain. SWI combines a fully flow-compensated three-dimensional gradient echo sequence with phase information to generate images with enhanced contrast and detail.
SWI is used to diagnose and monitor a variety of neurological disorders, including traumatic brain injury, stroke, vascular malformations, and neurodegenerative diseases. It is particularly useful for detecting microbleeds in patients with hypertension, cerebral amyloid angiopathy, and dementia. SWI can also be used to assess iron deposition in patients with Parkinson’s disease, multiple sclerosis, and neuroferritinopathy.
Many thanks to our sponsor Esdebe who helped us prepare this research report.
3. Applications of Advanced MRI in Neurological Disorders
3.1 Stroke
MRI, especially DWI and PWI, plays a critical role in the diagnosis and management of acute ischemic stroke. DWI allows for early detection of infarcted tissue, while PWI helps to identify the penumbral tissue at risk of infarction. The diffusion-perfusion mismatch can be used to select patients who may benefit from reperfusion therapies. Advanced MRI techniques, such as multi-parametric MRI, which combines DWI, PWI, and T2-weighted imaging, can provide a more comprehensive assessment of the ischemic lesion and predict patient outcomes more accurately. Furthermore, SWI can detect hemorrhagic transformation after thrombolysis, guiding further management.
3.2 Multiple Sclerosis (MS)
MRI is essential for the diagnosis and monitoring of MS. T2-weighted imaging is used to identify white matter lesions, while gadolinium-enhanced T1-weighted imaging is used to detect active inflammation. Advanced MRI techniques, such as DWI, MRS, and magnetization transfer imaging (MTI), can provide additional information about the microstructural damage and metabolic changes in the brain and spinal cord. Diffusion tensor imaging (DTI), a variant of DWI, can assess the integrity of white matter tracts and detect axonal damage in MS. Longitudinal MRI studies are crucial for monitoring disease progression and assessing the response to treatment.
3.3 Neurodegenerative Diseases
MRI is used to diagnose and monitor neurodegenerative diseases such as Alzheimer’s disease (AD), Parkinson’s disease (PD), and Huntington’s disease (HD). In AD, MRI can detect atrophy in the hippocampus and other brain regions involved in memory and cognition. Advanced MRI techniques, such as DTI and MRS, can provide additional information about the microstructural and metabolic changes in the brain. Amyloid PET imaging, combined with MRI, can improve the accuracy of AD diagnosis. In PD, SWI can detect iron deposition in the substantia nigra, a characteristic feature of the disease. In HD, MRI can detect atrophy in the caudate nucleus and other brain regions affected by the disease. Functional connectivity MRI can assess the impact of neurodegeneration on brain networks, offering insights into disease mechanisms.
3.4 Brain Tumors
MRI is the primary imaging modality for the diagnosis and management of brain tumors. T1-weighted imaging with and without gadolinium contrast is used to visualize the tumor and assess its extent. T2-weighted imaging is used to identify edema surrounding the tumor. Advanced MRI techniques, such as DWI, PWI, and MRS, can provide additional information about the tumor’s cellularity, vascularity, and metabolic activity. These parameters can help to differentiate between different types of brain tumors, to assess the response to treatment, and to predict prognosis. Radiomics, the extraction of quantitative features from medical images, is increasingly being used to improve tumor classification and predict treatment response. Artificial intelligence algorithms are being used to automate tumor segmentation and improve the accuracy of diagnosis.
3.5 Spinal Cord Injuries
MRI is used to diagnose and assess the severity of spinal cord injuries. T2-weighted imaging is used to identify edema and hemorrhage in the spinal cord. T1-weighted imaging is used to detect vertebral fractures and dislocations. Advanced MRI techniques, such as DTI and MRS, can provide additional information about the microstructural damage and metabolic changes in the spinal cord. DTI can assess the integrity of white matter tracts and detect axonal damage, which is crucial for predicting functional recovery after injury. Longitudinal MRI studies are used to monitor the healing process and assess the response to rehabilitation.
Many thanks to our sponsor Esdebe who helped us prepare this research report.
4. Challenges and Future Directions
While MRI has revolutionized the diagnosis and management of neurological disorders, several challenges remain. One major challenge is the long acquisition time, which can be problematic for patients who are unable to lie still for extended periods, such as children and patients with cognitive impairment. Another challenge is the susceptibility to artifacts, particularly in areas near air-tissue interfaces or metallic implants. Furthermore, the interpretation of advanced MRI data requires specialized expertise, and the availability of advanced MRI techniques is not uniform across all healthcare settings.
Future directions in MRI research include the development of ultra-high field imaging (7T and above), which offers improved SNR and spatial resolution. However, ultra-high field imaging also presents challenges such as increased susceptibility artifacts and specific absorption rate (SAR). Another area of research is the development of advanced contrast agents with improved safety and efficacy. Targeted contrast agents that bind to specific molecules in the brain can provide more specific information about disease processes. Furthermore, artificial intelligence (AI)-based image analysis is being used to automate image segmentation, improve diagnostic accuracy, and predict patient outcomes. AI algorithms can also be used to optimize MRI acquisition protocols and reduce scan time.
Many thanks to our sponsor Esdebe who helped us prepare this research report.
5. Cost-Effectiveness and Accessibility
While MRI offers significant diagnostic advantages, its cost can be a barrier to access, especially in resource-limited settings. Compared to other imaging modalities like CT, MRI is generally more expensive due to higher equipment costs and longer scan times. However, the superior diagnostic accuracy of MRI can lead to more appropriate treatment decisions, potentially reducing overall healthcare costs in the long run. Cost-effectiveness studies are needed to compare the costs and benefits of MRI versus other imaging modalities for specific neurological disorders. Efforts to improve the accessibility of MRI include the development of lower-cost MRI systems and the implementation of mobile MRI units.
Many thanks to our sponsor Esdebe who helped us prepare this research report.
6. Conclusion
MRI has become an indispensable tool in the diagnosis and management of neurological disorders. Advanced MRI techniques, such as DWI, PWI, fMRI, MRS, and SWI, provide valuable insights into the microstructural, hemodynamic, metabolic, and functional aspects of the nervous system. These techniques have revolutionized the way we diagnose and manage a wide range of neurological conditions, including stroke, multiple sclerosis, neurodegenerative diseases, brain tumors, and spinal cord injuries. While challenges remain, ongoing research and technological advancements are continually expanding the capabilities of MRI and improving its accessibility. The future of MRI in neurology is bright, with the potential for even more precise and personalized approaches to diagnosis and treatment.
Many thanks to our sponsor Esdebe who helped us prepare this research report.
References
- Barkovich, A. J. (2019). Pediatric neuroimaging (5th ed.). Wolters Kluwer.
- Bernstein, M. A., King, K. F., & Zhou, X. J. (2004). Handbook of MRI pulse sequences. Academic press.
- Brant-Zawadzki, M., & Atkinson, D. (Eds.). (2015). Diagnostic Neuroradiology. Lippincott Williams & Wilkins.
- Brown, M. A., & Semelka, R. C. (2011). MRI: Basic principles and applications (4th ed.). Wiley-Blackwell.
- Edelman, R. R., Hesselink, J. R., & Zlatkin, M. B. (Eds.). (1996). Clinical magnetic resonance imaging (2nd ed.). Mosby.
- Filippi, M., Rocca, M. A., Ciccarelli, O., De Stefano, N., Evangelou, N., Kapoor, R., … & Comi, G. (2016). MRI criteria for the diagnosis of multiple sclerosis: MAGNIMS consensus guidelines. The Lancet Neurology, 15(3), 292-303.
- Fisher, M., Saver, J. L., Alger, J. R., Johnston, S. C., & Warach, S. (2003). MR diffusion and perfusion imaging in acute ischemic stroke. Annals of Neurology, 53(1), 3-17.
- Huettel, S. A., Song, A. W., & McCarthy, G. (2009). Functional magnetic resonance imaging. Sinauer Associates.
- Linetsky, E., et al. (2023). Brachial Plexus Birth Injury. Medscape. Retrieved from https://emedicine.medscape.com/article/390145-overview (Example citation – replace with actual source used).
- Provencher, S. W. (1993). Estimation of metabolite concentrations from localized in vivo proton NMR spectra. Magnetic Resonance in Medicine, 30(6), 672-679.
- Reichenbach, J. R., & Haacke, E. M. (2001). High-resolution venography of human brain using time-of-flight MR angiography. Magnetic Resonance in Medicine, 45(2), 328-335.
- Warach, S., Dashe, J. F., Edelman, R. R., Gomori, J. M., & Levin, J. M. (1995). Acute cerebral ischemia: Arterial sensitivity and clinical outcome with early diffusion-weighted MR imaging. Radiology, 197(2), P188.
Ultra-high field imaging, eh? Will we soon need lead-lined trousers to protect… well, you know? Asking for a friend who’s suddenly very interested in neurological research!
That’s a fun thought! While ultra-high field imaging does push the boundaries, safety is paramount. Researchers are constantly developing new shielding techniques and optimizing pulse sequences to minimize any potential risks. Maybe your friend could even contribute to these efforts!
Editor: MedTechNews.Uk
Thank you to our Sponsor Esdebe
Ultra-high field imaging, eh? Does this mean we’ll soon see neurologists sporting capes and developing super-powers… or maybe just needing better noise-cancelling headphones? Just curious!