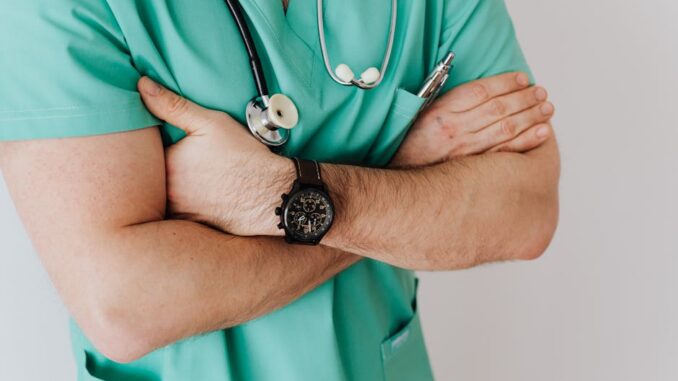
Abstract
Heart failure (HF) remains a significant global health challenge, characterized by the heart’s inability to pump blood effectively to meet the body’s needs. Despite advancements in medical and device therapies, mortality and morbidity associated with HF remain high, underscoring the need for novel approaches to prevention, diagnosis, and treatment. This research report provides a comprehensive overview of the current understanding of HF, encompassing its pathophysiology, diagnostic modalities, and therapeutic strategies, with a particular focus on recent breakthroughs and emerging concepts. We delve into the intricate molecular mechanisms underlying HF development and progression, including genetic predispositions, cellular remodeling processes, and the role of non-coding RNAs. Furthermore, we explore the potential of cutting-edge diagnostic techniques, such as advanced cardiac imaging and biomarker analysis, in facilitating early detection and risk stratification. The report also critically evaluates the efficacy and limitations of current pharmacological and device-based therapies, highlighting the need for personalized medicine approaches tailored to individual patient profiles. Finally, we discuss promising novel therapeutic strategies, including gene therapy, regenerative medicine, and targeted therapies, that hold the potential to revolutionize HF management and improve patient outcomes. This review aims to provide an in-depth analysis of the current state-of-the-art and future directions in HF research and clinical practice, catering to the interests of experts in the field.
Many thanks to our sponsor Esdebe who helped us prepare this research report.
1. Introduction
Heart failure (HF) is a complex clinical syndrome that arises when the heart is unable to pump sufficient blood to meet the body’s metabolic demands, or can only do so at elevated filling pressures. This condition represents a significant burden on healthcare systems worldwide, affecting millions of individuals and contributing substantially to hospitalizations, disability, and mortality [1]. The prevalence of HF is projected to increase in the coming decades, driven by the aging population, the rising incidence of cardiovascular risk factors such as hypertension and diabetes, and improved survival rates following acute myocardial infarction [2]. HF is not a single disease entity but rather a heterogeneous syndrome with diverse etiologies, including coronary artery disease, hypertension, valvular heart disease, cardiomyopathy, and congenital heart defects. Furthermore, HF can manifest in different forms, characterized by either reduced ejection fraction (HFrEF), preserved ejection fraction (HFpEF), or mid-range ejection fraction (HFmrEF), each exhibiting distinct pathophysiological mechanisms and clinical outcomes [3].
Traditionally, HF management has focused on alleviating symptoms, improving quality of life, and reducing mortality through pharmacological interventions, device therapies, and lifestyle modifications. However, despite these advancements, a significant proportion of patients continue to experience disease progression, recurrent hospitalizations, and premature death, highlighting the limitations of current treatment strategies [4]. This necessitates a deeper understanding of the underlying molecular mechanisms driving HF development and progression, as well as the identification of novel therapeutic targets and personalized approaches to management. The purpose of this research report is to provide a comprehensive overview of the current state-of-the-art in HF research and clinical practice, encompassing the latest advancements in understanding the pathophysiology, diagnosis, and treatment of this complex syndrome. The report will delve into the intricacies of molecular mechanisms, explore the potential of cutting-edge diagnostic techniques, critically evaluate the efficacy of current therapies, and discuss promising novel therapeutic strategies that hold the potential to revolutionize HF management and improve patient outcomes. This review is intended to cater to the interests of experts in the field, providing an in-depth analysis of the current challenges and future directions in HF research and clinical practice.
Many thanks to our sponsor Esdebe who helped us prepare this research report.
2. Pathophysiology of Heart Failure: Molecular Mechanisms and Cellular Remodeling
The pathophysiology of HF is a complex interplay of molecular, cellular, and systemic factors that ultimately lead to impaired cardiac function. At the molecular level, dysregulation of gene expression, protein synthesis, and intracellular signaling pathways contribute to cardiomyocyte dysfunction, hypertrophy, and apoptosis. Cellular remodeling processes, such as fibrosis and inflammation, further exacerbate the decline in cardiac performance. Understanding these intricate mechanisms is crucial for identifying potential therapeutic targets and developing more effective treatments for HF.
2.1. Genetic Predisposition and Inherited Cardiomyopathies
A significant proportion of HF cases have a genetic component, with mutations in genes encoding for sarcomeric proteins, ion channels, and cytoskeletal proteins predisposing individuals to dilated cardiomyopathy (DCM), hypertrophic cardiomyopathy (HCM), and other inherited cardiomyopathies [5]. These genetic mutations can disrupt cardiomyocyte structure and function, leading to contractile dysfunction, arrhythmias, and ultimately HF. For example, mutations in genes encoding for myosin heavy chain (MYH7) and cardiac troponin T (TNNT2) are commonly found in HCM, while mutations in genes encoding for dystrophin (DMD) and lamin A/C (LMNA) are associated with DCM [6]. Advances in genetic sequencing technologies have enabled the identification of novel disease-causing genes and the development of personalized risk assessment tools for individuals with a family history of HF. However, genetic testing remains costly and complex, and the interpretation of genetic variants requires expertise and caution. Furthermore, the penetrance and expressivity of genetic mutations can vary widely, making it challenging to predict the clinical course of HF in affected individuals.
2.2. Myocardial Hypertrophy and Remodeling
Myocardial hypertrophy is an adaptive response to increased hemodynamic stress, such as hypertension or aortic stenosis. Initially, hypertrophy can compensate for the increased workload by increasing myocardial mass and contractile force. However, prolonged hypertrophy can lead to maladaptive remodeling, characterized by cardiomyocyte apoptosis, fibrosis, and changes in gene expression. These structural and functional changes ultimately impair cardiac performance and contribute to the development of HF [7]. Several signaling pathways, including the renin-angiotensin-aldosterone system (RAAS), the sympathetic nervous system (SNS), and the mitogen-activated protein kinase (MAPK) pathway, play a critical role in mediating myocardial hypertrophy and remodeling [8]. Pharmacological interventions that target these pathways, such as angiotensin-converting enzyme inhibitors (ACEIs), angiotensin receptor blockers (ARBs), and beta-blockers, have been shown to reduce myocardial hypertrophy and improve outcomes in patients with HF.
2.3. Fibrosis and Extracellular Matrix Remodeling
Cardiac fibrosis is characterized by the excessive accumulation of extracellular matrix (ECM) components, such as collagen, in the myocardial interstitium. Fibrosis disrupts the normal architecture of the heart, impairs cardiomyocyte contractility, and increases myocardial stiffness, ultimately leading to diastolic dysfunction and HF [9]. Several factors contribute to cardiac fibrosis, including inflammation, oxidative stress, and activation of fibroblasts. Transforming growth factor-beta (TGF-β) is a key profibrotic cytokine that stimulates collagen synthesis and inhibits collagen degradation [10]. Emerging therapeutic strategies aimed at inhibiting TGF-β signaling or targeting other profibrotic pathways are being investigated as potential treatments for HF.
2.4. Inflammation and Immune Response
Inflammation plays a complex and often detrimental role in the pathogenesis of HF. Pro-inflammatory cytokines, such as tumor necrosis factor-alpha (TNF-α) and interleukin-1β (IL-1β), are elevated in patients with HF and contribute to cardiomyocyte dysfunction, apoptosis, and remodeling [11]. The immune system is also activated in HF, with infiltration of macrophages and other immune cells into the myocardium. While the initial inflammatory response may be protective, chronic inflammation can perpetuate cardiac damage and accelerate disease progression. Targeting inflammatory pathways with anti-inflammatory agents has shown promise in preclinical studies, but clinical trials have yielded mixed results. Further research is needed to better understand the role of inflammation in HF and to identify effective anti-inflammatory therapies.
2.5. Role of Non-coding RNAs
Non-coding RNAs, such as microRNAs (miRNAs) and long non-coding RNAs (lncRNAs), are emerging as important regulators of gene expression and cellular function in HF [12]. miRNAs are small RNA molecules that bind to messenger RNAs (mRNAs) and inhibit their translation or promote their degradation. LncRNAs are longer RNA molecules that can regulate gene expression by interacting with DNA, RNA, or proteins. Dysregulation of miRNAs and lncRNAs has been implicated in the pathogenesis of HF, with some miRNAs promoting cardiomyocyte hypertrophy, fibrosis, and apoptosis, while others exert protective effects. Identifying specific miRNAs and lncRNAs that are dysregulated in HF and developing targeted therapies that modulate their expression hold promise as novel therapeutic strategies.
Many thanks to our sponsor Esdebe who helped us prepare this research report.
3. Advanced Diagnostic Techniques for Early Detection and Risk Stratification
Early detection and accurate risk stratification are crucial for improving outcomes in patients with HF. Traditional diagnostic methods, such as echocardiography and electrocardiography, have limitations in detecting subtle changes in cardiac function and predicting disease progression. Advanced cardiac imaging techniques and biomarker analysis offer the potential to overcome these limitations and facilitate earlier and more precise diagnosis and risk assessment.
3.1. Advanced Cardiac Imaging
3.1.1. Cardiac Magnetic Resonance (CMR)
CMR is a non-invasive imaging modality that provides detailed anatomical and functional information about the heart [13]. CMR can assess left ventricular (LV) and right ventricular (RV) volumes, ejection fraction, and myocardial mass with high accuracy. Furthermore, CMR can detect myocardial fibrosis, edema, and inflammation, providing valuable insights into the underlying pathophysiology of HF. Late gadolinium enhancement (LGE) imaging, a specific CMR technique, is used to identify areas of myocardial scar or fibrosis, which are associated with increased risk of adverse events. Stress CMR, performed during exercise or pharmacological stress, can detect myocardial ischemia and assess coronary artery disease in patients with HF.
3.1.2. Cardiac Computed Tomography (CCT)
CCT is another non-invasive imaging modality that can provide detailed anatomical information about the heart and coronary arteries [14]. CCT can be used to assess coronary artery calcium (CAC) score, which is a marker of coronary artery disease burden. Coronary computed tomography angiography (CCTA) can visualize the coronary arteries and detect stenosis or plaque formation. CCT is particularly useful for evaluating patients with suspected coronary artery disease who are not suitable for invasive angiography. However, CCT involves exposure to ionizing radiation and may not be suitable for patients with renal impairment.
3.1.3. Three-Dimensional Echocardiography (3DE)
3DE is an advanced echocardiographic technique that provides a three-dimensional reconstruction of the heart [15]. 3DE can accurately assess LV and RV volumes, ejection fraction, and myocardial mass, overcoming some of the limitations of traditional two-dimensional echocardiography. 3DE is also useful for evaluating valvular heart disease and congenital heart defects. However, 3DE image quality can be affected by patient body habitus and lung disease.
3.2. Biomarker Analysis
3.2.1. Natriuretic Peptides
B-type natriuretic peptide (BNP) and N-terminal pro-BNP (NT-proBNP) are cardiac hormones that are released in response to increased myocardial wall stress [16]. BNP and NT-proBNP are widely used as diagnostic and prognostic biomarkers in HF. Elevated levels of BNP and NT-proBNP are indicative of HF and are associated with increased risk of hospitalization and death. However, BNP and NT-proBNP levels can be affected by other factors, such as age, renal function, and obesity, which can limit their diagnostic accuracy.
3.2.2. High-Sensitivity Troponin
High-sensitivity troponin (hs-troponin) assays can detect very low levels of cardiac troponin, a marker of myocardial injury [17]. Elevated hs-troponin levels are indicative of myocardial damage and are associated with increased risk of adverse events in patients with HF. Hs-troponin can be used to identify patients with subclinical myocardial injury who may benefit from early intervention.
3.2.3. Novel Biomarkers
Several novel biomarkers are being investigated as potential diagnostic and prognostic tools in HF. These include markers of inflammation, oxidative stress, myocardial fibrosis, and cardiomyocyte remodeling [18]. For example, galectin-3 is a marker of myocardial fibrosis, soluble ST2 is a marker of inflammation, and growth differentiation factor-15 (GDF-15) is a marker of cellular stress. These novel biomarkers may provide additional information about the underlying pathophysiology of HF and help to identify patients who are at high risk of adverse events.
Many thanks to our sponsor Esdebe who helped us prepare this research report.
4. Current and Emerging Therapeutic Strategies
The management of HF has evolved significantly over the past few decades, with the introduction of new pharmacological agents and device therapies that have improved patient outcomes. However, despite these advancements, HF remains a challenging condition to treat, and a significant proportion of patients continue to experience disease progression and adverse events. This necessitates the development of novel therapeutic strategies that target the underlying pathophysiology of HF and address the unmet needs of patients.
4.1. Pharmacological Therapies
4.1.1. RAAS Inhibitors
ACEIs and ARBs are cornerstone therapies for HFrEF [19]. They block the RAAS, reducing vasoconstriction, sodium retention, and myocardial remodeling. Angiotensin receptor-neprilysin inhibitors (ARNIs), such as sacubitril/valsartan, combine ARB activity with neprilysin inhibition, further enhancing natriuresis and vasodilation [20]. ARNIs have demonstrated superior efficacy compared to ACEIs in reducing hospitalization and mortality in patients with HFrEF.
4.1.2. Beta-Blockers
Beta-blockers are also recommended for HFrEF, as they block the effects of the SNS, reducing heart rate, blood pressure, and myocardial oxygen demand [21]. Beta-blockers have been shown to improve LV function and reduce mortality in patients with HFrEF. However, beta-blockers should be initiated at low doses and titrated slowly to avoid worsening HF symptoms.
4.1.3. Mineralocorticoid Receptor Antagonists (MRAs)
MRAs, such as spironolactone and eplerenone, block the effects of aldosterone, reducing sodium retention, potassium loss, and myocardial fibrosis [22]. MRAs have been shown to improve outcomes in patients with HFrEF and should be considered in patients who remain symptomatic despite treatment with ACEIs/ARBs, beta-blockers, and diuretics.
4.1.4. Sodium-Glucose Cotransporter 2 (SGLT2) Inhibitors
SGLT2 inhibitors, such as empagliflozin, dapagliflozin, and canagliflozin, are a newer class of drugs that were initially developed for the treatment of diabetes [23]. However, clinical trials have shown that SGLT2 inhibitors also improve outcomes in patients with HF, regardless of diabetes status. SGLT2 inhibitors reduce hospitalization and mortality in patients with HFrEF and HFpEF. The mechanism of action of SGLT2 inhibitors in HF is not fully understood but may involve improvements in myocardial energetics, inflammation, and fibrosis.
4.1.5. Novel Pharmacological Agents
Several novel pharmacological agents are being developed for the treatment of HF. These include selective cardiac myosin activators, soluble guanylate cyclase stimulators, and inhibitors of inflammation and fibrosis [24]. These novel agents may offer new hope for patients with HF who do not respond to conventional therapies.
4.2. Device Therapies
4.2.1. Implantable Cardioverter-Defibrillators (ICDs)
ICDs are implanted devices that can deliver electrical shocks to terminate life-threatening ventricular arrhythmias [25]. ICDs are recommended for patients with HFrEF who are at high risk of sudden cardiac death. However, ICDs do not prevent HF progression and may be associated with complications, such as inappropriate shocks and lead-related problems.
4.2.2. Cardiac Resynchronization Therapy (CRT)
CRT is a pacing therapy that synchronizes the contraction of the left and right ventricles [26]. CRT is recommended for patients with HFrEF and left bundle branch block who are symptomatic despite optimal medical therapy. CRT has been shown to improve LV function, reduce hospitalization, and improve survival in selected patients with HFrEF.
4.2.3. Mechanical Circulatory Support (MCS)
MCS devices, such as left ventricular assist devices (LVADs), are implanted pumps that assist the failing heart in pumping blood [27]. LVADs are used as a bridge to transplantation in patients with advanced HF who are awaiting a heart transplant. LVADs are also used as destination therapy in patients with advanced HF who are not eligible for heart transplantation. LVADs can improve symptoms, quality of life, and survival in patients with advanced HF, but are associated with significant complications, such as bleeding, infection, and stroke.
4.3. Gene Therapy and Regenerative Medicine
Gene therapy and regenerative medicine approaches hold promise for repairing damaged myocardium and restoring cardiac function in patients with HF [28]. Gene therapy involves delivering genes into the heart to correct genetic defects or enhance cardiac function. Regenerative medicine involves using stem cells or other biological materials to regenerate damaged myocardium. Several gene therapy and regenerative medicine strategies are being investigated in preclinical and clinical studies, including delivery of genes encoding for sarcoplasmic reticulum calcium ATPase (SERCA2a), vascular endothelial growth factor (VEGF), and stromal cell-derived factor-1 (SDF-1), as well as transplantation of mesenchymal stem cells, cardiac progenitor cells, and induced pluripotent stem cell-derived cardiomyocytes. These approaches are still in early stages of development, but offer the potential to revolutionize the treatment of HF.
4.4. Personalized Medicine
Personalized medicine involves tailoring treatment strategies to individual patient characteristics, such as genetic profile, biomarker levels, and clinical phenotype [29]. Personalized medicine approaches have the potential to improve outcomes in patients with HF by identifying the most effective treatment strategies for each individual patient. For example, genetic testing can identify patients who are likely to respond to specific medications, while biomarker analysis can identify patients who are at high risk of adverse events. Personalized medicine approaches are becoming increasingly feasible with the development of new technologies, such as genomic sequencing and high-throughput biomarker analysis. However, the implementation of personalized medicine in HF requires further research and development.
Many thanks to our sponsor Esdebe who helped us prepare this research report.
5. Challenges and Future Directions
Despite significant advances in understanding and managing HF, several challenges remain. HF is a complex and heterogeneous syndrome with diverse etiologies and clinical manifestations. This makes it challenging to develop effective therapies that work for all patients. Furthermore, HF is often associated with other comorbidities, such as diabetes, hypertension, and renal disease, which can complicate management. The aging population and the rising incidence of cardiovascular risk factors are contributing to the increasing prevalence of HF, placing a growing burden on healthcare systems. Addressing these challenges requires a multi-faceted approach that includes:
- Improved understanding of HF pathophysiology: Further research is needed to elucidate the complex molecular mechanisms underlying HF development and progression. This will help to identify novel therapeutic targets and develop more effective treatments.
- Development of new diagnostic tools: New diagnostic tools are needed to detect HF earlier and to identify patients who are at high risk of adverse events. This will allow for earlier intervention and more personalized management.
- Development of new therapies: New therapies are needed to target the underlying pathophysiology of HF and to address the unmet needs of patients. This includes pharmacological agents, device therapies, gene therapy, and regenerative medicine approaches.
- Implementation of personalized medicine: Personalized medicine approaches have the potential to improve outcomes in patients with HF by tailoring treatment strategies to individual patient characteristics. This requires further research and development.
- Improved prevention strategies: Preventing HF is the most effective way to reduce its burden. This requires addressing modifiable risk factors, such as hypertension, diabetes, and obesity.
Many thanks to our sponsor Esdebe who helped us prepare this research report.
6. Conclusion
Heart failure remains a significant global health challenge, but substantial progress has been made in understanding its pathophysiology, improving diagnostic techniques, and developing new therapeutic strategies. Advancements in molecular biology have provided insights into the genetic and cellular mechanisms underlying HF, while advanced cardiac imaging and biomarker analysis have improved early detection and risk stratification. Novel pharmacological agents, device therapies, gene therapy, and regenerative medicine approaches hold promise for revolutionizing the treatment of HF. Personalized medicine approaches offer the potential to tailor treatment strategies to individual patient characteristics, further improving outcomes. Addressing the challenges that remain requires a continued commitment to research, innovation, and collaboration. By working together, we can improve the lives of millions of individuals affected by heart failure.
Many thanks to our sponsor Esdebe who helped us prepare this research report.
References
[1] Roger VL. Epidemiology of heart failure. Circ Res. 2013;113(6):646-59.
[2] Benjamin EJ, Muntner P, Alonso A, et al. Heart disease and stroke statistics—2019 update: a report from the American Heart Association. Circulation. 2019;139(10):e56-e528.
[3] McDonagh TA, Metra M, Adamo M, et al. 2021 ESC Guidelines for the diagnosis and treatment of acute and chronic heart failure. Eur Heart J. 2021;42(36):3599-726.
[4] Braunwald E. Heart failure. J Am Coll Cardiol. 2013;62(17):1606-18.
[5] Towbin JA, Jefferies JL. Cardiomyopathies due to genetic mutations: Prevalence, diagnosis, and management. Circulation. 2017;136(5):492-508.
[6] Elliott PM, Anastasakis A, Borger MA, et al. 2014 ESC Guidelines on diagnosis and management of hypertrophic cardiomyopathy: the Task Force for the Diagnosis and Management of Hypertrophic Cardiomyopathy of the European Society of Cardiology (ESC). Eur Heart J. 2014;35(39):2739-79.
[7] Mann DL. Mechanisms and models in heart failure: a combinatorial approach. Circulation. 1999;100(9):999-1008.
[8] Cohn JN, Ferrari R, Sharpe N. Cardiac remodeling–concepts and clinical implications: a consensus paper from an international forum on cardiac remodeling. J Am Coll Cardiol. 2000;35(3):568-82.
[9] Weber KT. Cardiac interstitium in health and disease: the fibrillar collagen network. J Am Coll Cardiol. 1989;13(4):880-6.
[10] Khalil N, Smith E, Nickerson-Nutter C, Blaxall BC, Goldstein RH. Increased production of transforming growth factor-beta 1 and collagen in bleomycin-induced pulmonary fibrosis in hamsters. Am J Respir Cell Mol Biol. 1991;5(2):155-62.
[11] Mann DL. Inflammatory mediators and the failing heart: past, present, and future. Circ Res. 2002;91(11):988-98.
[12] van Rooij E, Olson EN. MicroRNAs: novel regulators of cardiovascular disease. Circ Res. 2007;100(8):1169-80.
[13] Hundley WG, Bluemke DA, Finn JP, et al. ACCF/ACR/AHA/NASCI/SCMR 2010 expert consensus document on cardiovascular magnetic resonance: a report of the American College of Cardiology Foundation Task Force on Expert Consensus Documents. J Am Coll Cardiol. 2010;55(23):2614-62.
[14] Budoff MJ, Achenbach S, Blumenthal RS, et al. Assessment of coronary artery disease by cardiac computed tomography: a scientific statement from the American Heart Association. Circulation. 2006;114(16):1761-91.
[15] Mor-Avi V, Lang RM, Badano LP, et al. Current and evolving echocardiographic techniques for the quantitative evaluation of cardiac mechanics: ASE/EAE consensus statement on methodology. J Am Soc Echocardiogr. 2011;24(3):277-313.
[16] Daniels LB, Maisel AS. Natriuretic peptides. J Am Coll Cardiol. 2007;50(23):2357-68.
[17] Thygesen K, Alpert JS, Jaffe AS, et al. Fourth universal definition of myocardial infarction (2018). J Am Coll Cardiol. 2018;72(22):2231-64.
[18] Bayes-Genis A, Barallat J, Domingo M, et al. Novel biomarkers in heart failure. Rev Esp Cardiol (Engl Ed). 2010;63(8):959-67.
[19] Yusuf S, Pitt B, Davis R, et al. Effect of enalapril on survival in patients with chronic heart failure. N Engl J Med. 1991;325(5):293-302.
[20] McMurray JJV, Packer M, Desai AS, et al. Angiotensin-Neprilysin Inhibition versus Enalapril in Heart Failure. N Engl J Med. 2014;371(11):993-1004.
[21] Packer M, Bristow MR, Cohn JN, et al. The effect of carvedilol on morbidity and mortality in patients with chronic heart failure. N Engl J Med. 2001;344(18):1349-58.
[22] Pitt B, Zannad F, Remme WJ, et al. The effect of spironolactone on morbidity and mortality in patients with severe heart failure. N Engl J Med. 1999;341(10):709-17.
[23] Packer M, Anker SD, Butler J, et al. Cardiovascular and Renal Outcomes with Empagliflozin in Heart Failure. N Engl J Med. 2020;383(15):1413-24.
[24] Teerlink JR, Diaz R, Felker GM, et al. Omecamtiv Mecarbil in Chronic Heart Failure with Reduced Ejection Fraction. N Engl J Med. 2020;382(26):2590-9.
[25] Moss AJ, Hall WJ, Cannom DS, et al. Improved survival with an implanted defibrillator in patients with coronary disease at high risk for ventricular arrhythmia. N Engl J Med. 1996;335(26):1933-40.
[26] Cleland JG, Daubert JC, Erdmann E, et al. Cardiac Resynchronization Therapy in Heart Failure (CARE-HF) study investigators. The effect of cardiac resynchronization on morbidity and mortality in heart failure. N Engl J Med. 2005;352(15):1539-49.
[27] Slaughter MS, Rogers JG, Milano CA, et al. Advanced heart failure treated with continuous-flow left ventricular assist device. N Engl J Med. 2009;361(23):2241-51.
[28] Hajjar RJ, Schmidt-Lucke JA, Sawa Y, Dec GW. Gene therapy for heart failure. Nature Reviews Cardiology. 2013;10(10):608-18.
[29] Shah SJ, Desai AS, Anker SD, et al. Phenotype-specific treatment of heart failure with preserved ejection fraction: a PARAGON-HF exploratory analysis. Lancet. 2021;398(10304):963-72.
The discussion of non-coding RNAs in heart failure pathophysiology is particularly compelling. Could exploring the potential of engineered exosomes to deliver targeted miRNA therapies offer a novel approach to modulate cardiac remodeling and improve outcomes?
That’s a great point! The potential of engineered exosomes for targeted miRNA delivery is indeed a promising avenue. The precision and specificity they could offer in modulating cardiac remodeling processes could be transformative. It’s exciting to consider the possibilities for improving patient outcomes through this approach. Thanks for sharing your insight!
Editor: MedTechNews.Uk
Thank you to our Sponsor Esdebe
Given the complexity of HF pathophysiology involving genetic predispositions, are there specific genetic markers that consistently predict the severity or progression of heart failure across diverse populations and etiologies?
That’s a fantastic question! Identifying consistent genetic markers across diverse populations is indeed a major challenge. While specific mutations are linked to cardiomyopathies, predicting HF severity across different etiologies is complex. Ongoing research, including genome-wide association studies (GWAS), aims to identify more universal predictive markers. Precision medicine approaches might offer solutions for individual risk stratification.
Editor: MedTechNews.Uk
Thank you to our Sponsor Esdebe
Fantastic overview! With all those genes and cellular mechanisms, I’m starting to think untangling heart failure is more complex than assembling IKEA furniture without the instructions. Maybe we need a “Heart Failure for Dummies” guide…sponsored by Esdebe, of course.
Thanks for the kind words! Your IKEA analogy is spot on! Breaking down the complexity is key. Perhaps a series of easily digestible infographics focusing on specific mechanisms could be a good starting point before a complete guide. Always grateful for Esdebe’s support!
Editor: MedTechNews.Uk
Thank you to our Sponsor Esdebe