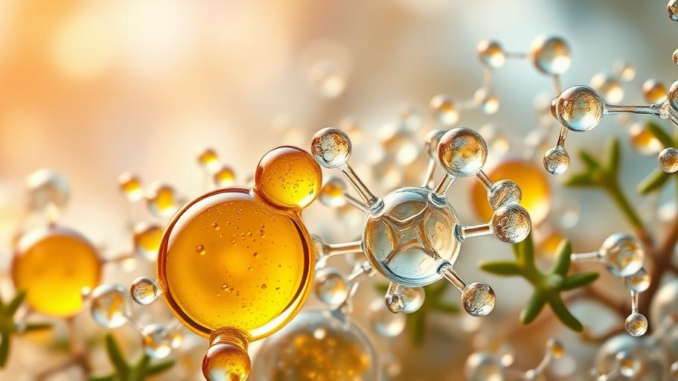
Abstract
Ceramides, a family of sphingolipids, are increasingly recognized as pivotal players in a diverse array of cellular processes, extending far beyond their initial identification as structural components of the stratum corneum. This review provides a comprehensive overview of ceramide biosynthesis, metabolism, and diverse molecular species, highlighting their multifaceted roles in various tissues and their involvement in a spectrum of diseases. We delve into the specific enzymes and regulatory mechanisms governing ceramide metabolism, with a particular focus on Nogo-B and ORMDL proteins, clarifying their intricate interactions and their influence on cellular ceramide homeostasis. Furthermore, we address the evolving perspective on ceramides, reconciling the traditional view of elevated ceramide levels as detrimental with the emerging understanding of their essential functions and the potential implications of ceramide deficiency. Finally, we discuss current methods for measuring ceramide levels and explore potential therapeutic interventions targeting ceramide pathways, aiming to modulate ceramide metabolism for therapeutic benefit in various disease contexts.
Many thanks to our sponsor Esdebe who helped us prepare this research report.
1. Introduction
Ceramides, consisting of a sphingosine base N-acylated with a fatty acid, represent a complex class of lipids with fundamental roles in cellular structure and signaling. Historically viewed primarily as key structural components of the stratum corneum, contributing to the skin’s barrier function, ceramides are now appreciated as bioactive molecules involved in cell growth, differentiation, apoptosis, inflammation, and insulin resistance [1, 2]. This expanded understanding has driven intense research into the synthesis, metabolism, and diverse functions of ceramides across a range of tissues and disease states.
The complexity of ceramide biology arises from the diversity of ceramide species, differing in the length and saturation of both the sphingosine base and the acyl chain. This diversity enables ceramides to exert distinct, and sometimes opposing, effects within cells and tissues. The intricate network of enzymes involved in ceramide synthesis and degradation further contributes to the complexity, allowing for precise regulation of ceramide levels and composition in response to various stimuli.
This review aims to provide a comprehensive and up-to-date overview of ceramide biology, covering the major aspects of ceramide synthesis, metabolism, and function. We will discuss the diverse roles of ceramides in different tissues, their involvement in various diseases, and the potential for therapeutic interventions targeting ceramide pathways. We will pay particular attention to the roles of Nogo-B and ORMDL proteins in ceramide regulation, and address the evolving understanding of the complex and sometimes paradoxical effects of ceramides in different physiological and pathological conditions.
Many thanks to our sponsor Esdebe who helped us prepare this research report.
2. Ceramide Synthesis
The de novo synthesis of ceramides begins with the condensation of palmitoyl-CoA and serine, catalyzed by serine palmitoyltransferase (SPT), to form 3-ketosphinganine [3]. SPT is a rate-limiting enzyme and a key regulator of sphingolipid biosynthesis. The activity of SPT is tightly regulated by several factors, including substrate availability, feedback inhibition by sphingolipids, and transcriptional regulation by various transcription factors.
The 3-ketosphinganine is then reduced to sphinganine by 3-ketosphinganine reductase. Sphinganine is N-acylated by ceramide synthases (CerS) to form dihydroceramides. There are six different CerS isoforms (CerS1-6) in mammals, each exhibiting specificity for acyl-CoA substrates with different chain lengths [4]. This specificity contributes to the generation of diverse ceramide species with distinct biological activities. For example, CerS2 primarily synthesizes ceramides with very long-chain fatty acids (C22-C26), while CerS6 preferentially utilizes C16-CoA.
Dihydroceramides are then desaturated by dihydroceramide desaturase 1 (DES1) to form ceramides. DES1 is a critical enzyme in ceramide biosynthesis, and its activity is essential for maintaining cellular ceramide levels and regulating ceramide-mediated signaling pathways. Besides de novo synthesis, ceramides can also be generated through the sphingomyelinase pathway, which involves the hydrolysis of sphingomyelin by sphingomyelinases, and the salvage pathway, which involves the breakdown of complex sphingolipids and the recycling of sphingosine. This complex interplay between different pathways ensures tight regulation of ceramide homeostasis.
Many thanks to our sponsor Esdebe who helped us prepare this research report.
3. Ceramide Metabolism
Ceramide metabolism is a dynamic process involving multiple enzymes that regulate ceramide levels and interconvert different sphingolipid species. Ceramides can be hydrolyzed by ceramidases to generate sphingosine and fatty acids [5]. There are five known ceramidases in mammals: acid ceramidase (ASAH1), neutral ceramidase (ASAH2), alkaline ceramidases (ACER1, ACER2, and ACER3), each exhibiting distinct substrate specificities, tissue distributions, and pH optima.
Sphingosine can be phosphorylated by sphingosine kinases (SphK1 and SphK2) to generate sphingosine-1-phosphate (S1P), a signaling lipid with diverse biological activities, including cell growth, survival, and inflammation [6]. The balance between ceramide and S1P levels is crucial for determining cell fate, with ceramide generally promoting apoptosis and S1P promoting cell survival. This balance is often referred to as the “sphingolipid rheostat”.
Ceramides can also be converted back to sphingomyelin by sphingomyelin synthases (SMS1 and SMS2) [7]. SMS1 is primarily located in the Golgi apparatus, while SMS2 is located at the plasma membrane. Sphingomyelin is a major structural component of cell membranes and is involved in various cellular processes, including membrane organization and signaling.
The activity of these enzymes is tightly regulated by various factors, including substrate availability, post-translational modifications, and interactions with other proteins. Dysregulation of ceramide metabolism has been implicated in various diseases, including cancer, cardiovascular disease, and neurodegenerative disorders.
Many thanks to our sponsor Esdebe who helped us prepare this research report.
4. Ceramide Species and Their Roles
Ceramides are a diverse family of lipids, differing in the length and saturation of both the sphingosine base and the acyl chain. The most common sphingosine base is sphingosine (d18:1), but other bases, such as dihydrosphingosine (d18:0) and phytosphingosine (t18:0), can also be found. The acyl chain can vary in length from C2 to C26 and can be saturated or unsaturated. The diversity of ceramide species allows for distinct biological activities, with different ceramides exhibiting different effects on cell growth, differentiation, apoptosis, and inflammation.
For example, C16-ceramide has been shown to promote apoptosis in various cell types [8], while C24-ceramide has been shown to protect cells from apoptosis [9]. This difference in activity may be due to the different interactions of these ceramides with other proteins or their different effects on membrane structure. Specific ceramide species are also enriched in particular tissues. For instance, very long-chain ceramides are abundant in the brain, where they play important roles in myelin formation and neuronal function [10]. In contrast, short-chain ceramides are more prevalent in the skin, where they contribute to the barrier function of the stratum corneum [11].
The specific roles of different ceramide species are still being investigated, but it is becoming increasingly clear that the ceramide composition of cells and tissues is a critical determinant of cell fate and tissue function. Furthermore, alterations in ceramide composition have been implicated in the pathogenesis of various diseases.
Many thanks to our sponsor Esdebe who helped us prepare this research report.
5. Ceramides in Different Tissues
Ceramides play diverse roles in various tissues, reflecting the specific functions of each tissue and the local regulation of ceramide metabolism.
5.1. Skin
In the skin, ceramides are essential components of the stratum corneum, forming a lipid barrier that prevents water loss and protects against environmental insults [12]. The major ceramide species in the skin are very long-chain ceramides, particularly C24-ceramide. Deficiency in skin ceramides has been implicated in various skin conditions, including atopic dermatitis and psoriasis.
5.2. Brain
In the brain, ceramides are involved in myelin formation, neuronal function, and neuroinflammation [13]. Very long-chain ceramides are abundant in myelin, where they contribute to the insulation of nerve fibers. Ceramides have also been implicated in neurodegenerative disorders, such as Alzheimer’s disease and Parkinson’s disease. In these diseases, increased ceramide levels have been shown to promote neuronal apoptosis and inflammation.
5.3. Liver
In the liver, ceramides are involved in lipid metabolism, insulin resistance, and non-alcoholic fatty liver disease (NAFLD) [14]. Elevated ceramide levels in the liver have been shown to impair insulin signaling and promote lipid accumulation, leading to NAFLD. Inhibition of ceramide synthesis has been shown to improve insulin sensitivity and reduce liver fat content in animal models of NAFLD.
5.4. Pancreas
In the pancreas, ceramides are involved in insulin secretion and beta-cell function [15]. Increased ceramide levels in pancreatic beta-cells have been shown to impair insulin secretion and promote beta-cell apoptosis, leading to type 2 diabetes. Inhibition of ceramide synthesis has been shown to improve insulin secretion and protect beta-cells from apoptosis in animal models of type 2 diabetes.
5.5. Muscle
In skeletal muscle, ceramides contribute to insulin resistance and impaired glucose uptake [16]. Intramyocellular lipid accumulation, including ceramides, is strongly associated with insulin resistance. Reducing ceramide levels in muscle can improve insulin sensitivity and glucose metabolism.
Many thanks to our sponsor Esdebe who helped us prepare this research report.
6. Ceramides in Disease
Ceramides have been implicated in a wide range of diseases, highlighting their importance in maintaining cellular homeostasis and tissue function.
6.1. Cardiovascular Disease
Increased ceramide levels have been associated with an increased risk of cardiovascular disease [17]. Ceramides promote endothelial dysfunction, inflammation, and atherosclerosis. Ceramide accumulation in the heart can lead to cardiac dysfunction and heart failure. Plasma ceramide levels are emerging as potential biomarkers for cardiovascular risk.
6.2. Diabetes
Ceramides contribute to insulin resistance and pancreatic beta-cell dysfunction, leading to type 2 diabetes [18]. Ceramides impair insulin signaling in muscle, liver, and adipose tissue. Increased ceramide levels in pancreatic beta-cells impair insulin secretion and promote beta-cell apoptosis.
6.3. Cancer
The role of ceramides in cancer is complex and context-dependent [19]. In some cancers, ceramides promote apoptosis and inhibit tumor growth. In other cancers, ceramides promote cell survival and metastasis. The specific role of ceramides in cancer depends on the cell type, the ceramide species, and the stage of tumor development.
6.4. Neurodegenerative Disorders
Increased ceramide levels have been implicated in neurodegenerative disorders, such as Alzheimer’s disease and Parkinson’s disease [20]. Ceramides promote neuronal apoptosis, inflammation, and synaptic dysfunction. Modulation of ceramide metabolism may offer therapeutic potential for these disorders.
6.5. Skin Conditions
Deficiency in skin ceramides has been implicated in various skin conditions, including atopic dermatitis and psoriasis [21]. Topical application of ceramides can improve skin barrier function and reduce inflammation in these conditions.
Many thanks to our sponsor Esdebe who helped us prepare this research report.
7. Nogo-B and ORMDL in Ceramide Regulation
Nogo-B and ORMDL proteins are key regulators of ceramide synthesis and metabolism. Nogo-B, a reticulon protein located in the endoplasmic reticulum (ER), interacts with SPT and regulates its activity [22]. Nogo-B promotes the formation of the SPT complex and increases ceramide synthesis. Conversely, ORMDL proteins, also located in the ER, inhibit SPT activity and reduce ceramide synthesis [23].
The interplay between Nogo-B and ORMDL proteins is crucial for maintaining cellular ceramide homeostasis. ORMDL proteins are regulated by cellular stress and nutrient availability. When cells are under stress, ORMDL proteins are upregulated, leading to inhibition of SPT and reduction of ceramide synthesis. This mechanism protects cells from ceramide-mediated apoptosis.
The exact mechanisms by which Nogo-B and ORMDL proteins regulate SPT activity are still being investigated, but it is clear that these proteins play a critical role in controlling ceramide levels and influencing cell fate. Dysregulation of Nogo-B and ORMDL expression has been implicated in various diseases, including cancer and metabolic disorders.
Many thanks to our sponsor Esdebe who helped us prepare this research report.
8. Conflicting Viewpoints: High vs. Low Ceramide Levels
The traditional view of ceramides has been that elevated ceramide levels are generally detrimental, promoting apoptosis, inflammation, and insulin resistance. This view is supported by numerous studies showing that increased ceramide levels are associated with various diseases, including cardiovascular disease, diabetes, and neurodegenerative disorders [24].
However, an emerging perspective recognizes that ceramides are essential for various cellular functions and that ceramide deficiency can also be detrimental. For example, ceramides are crucial for the formation of the skin barrier and for myelin formation in the brain. Furthermore, some studies have shown that certain ceramide species can protect cells from apoptosis and promote cell survival [25].
The apparent conflict between these viewpoints can be resolved by considering the specific ceramide species, the tissue context, and the overall cellular environment. The effects of ceramides depend on their concentration, their location within the cell, and their interactions with other proteins and lipids. Furthermore, the cellular response to ceramides can be influenced by the presence of other signaling molecules and the activation of other signaling pathways.
Therefore, it is important to consider the complex interplay of factors that determine the effects of ceramides in different physiological and pathological conditions. A more nuanced understanding of ceramide biology is needed to develop effective therapeutic strategies targeting ceramide pathways.
Many thanks to our sponsor Esdebe who helped us prepare this research report.
9. Methods for Measuring Ceramide Levels
Accurate measurement of ceramide levels is essential for understanding their roles in health and disease. Several methods are available for measuring ceramide levels, including:
- Thin-layer chromatography (TLC): TLC is a traditional method for separating and quantifying lipids, including ceramides. It is a relatively simple and inexpensive method, but it is not very sensitive and requires large sample volumes [26].
- High-performance liquid chromatography (HPLC): HPLC is a more sensitive and versatile method for separating and quantifying lipids. It can be coupled to various detectors, such as UV detectors, fluorescence detectors, and mass spectrometers [27].
- Mass spectrometry (MS): MS is the most sensitive and specific method for measuring ceramide levels. It can be used to identify and quantify individual ceramide species with high accuracy. MS-based methods, such as liquid chromatography-mass spectrometry (LC-MS) and gas chromatography-mass spectrometry (GC-MS), are widely used in ceramide research [28].
- Enzyme-linked immunosorbent assay (ELISA): ELISA is a method for measuring ceramide levels using antibodies that specifically bind to ceramides. ELISA is a relatively simple and high-throughput method, but it may not be as sensitive or specific as MS-based methods [29].
The choice of method depends on the specific research question, the sample type, and the available resources. For accurate and comprehensive analysis of ceramide levels, MS-based methods are generally preferred.
Many thanks to our sponsor Esdebe who helped us prepare this research report.
10. Therapeutic Interventions Targeting Ceramide Pathways
The growing understanding of ceramide biology has led to the development of therapeutic interventions targeting ceramide pathways. These interventions aim to modulate ceramide metabolism for therapeutic benefit in various disease contexts. Several strategies are being explored, including:
- Inhibition of ceramide synthesis: Several inhibitors of SPT and CerS have been developed. Myriocin, a potent inhibitor of SPT, has been shown to reduce ceramide levels and improve insulin sensitivity in animal models of diabetes [30]. Eliglustat, an inhibitor of glucosylceramide synthase, is approved for the treatment of Gaucher disease [31].
- Enhancement of ceramide degradation: Strategies to enhance ceramide degradation include increasing the activity of ceramidases. However, the development of specific and effective ceramidase activators remains a challenge.
- Modulation of sphingolipid signaling: Targeting sphingolipid kinases and phosphatases can modulate the balance between ceramide and S1P. Sphingosine kinase inhibitors are being developed as potential anticancer agents [32].
- Topical application of ceramides: Topical application of ceramides can improve skin barrier function and reduce inflammation in skin conditions such as atopic dermatitis and psoriasis [33].
These therapeutic interventions are still in early stages of development, but they hold promise for the treatment of various diseases associated with dysregulation of ceramide metabolism. Future research will focus on developing more specific and effective therapeutic strategies targeting ceramide pathways.
Many thanks to our sponsor Esdebe who helped us prepare this research report.
11. Conclusion
Ceramides are multifaceted lipids with diverse roles in cellular structure, signaling, and tissue function. The complexity of ceramide biology arises from the diversity of ceramide species, the intricate network of enzymes involved in ceramide metabolism, and the context-dependent effects of ceramides in different tissues and disease states. While elevated ceramide levels have traditionally been viewed as detrimental, an emerging perspective recognizes the essential functions of ceramides and the potential implications of ceramide deficiency. Accurate measurement of ceramide levels is essential for understanding their roles in health and disease, and therapeutic interventions targeting ceramide pathways hold promise for the treatment of various diseases. Continued research into the intricate mechanisms governing ceramide synthesis, metabolism, and function will undoubtedly lead to a deeper understanding of their roles in health and disease, and pave the way for the development of novel therapeutic strategies.
Many thanks to our sponsor Esdebe who helped us prepare this research report.
References
[1] Hannun, Y. A., & Obeid, L. M. (2018). Sphingolipids and their metabolism in physiology and disease. Nature Reviews Molecular Cell Biology, 19(3), 175-191.
[2] Merrill Jr, A. H. (2011). Sphingolipids: complex lipids that regulate cell growth, differentiation, and death. The FASEB Journal, 25(8), 2584-2605.
[3] Hanada, K. (2003). Serine palmitoyltransferase, a key enzyme of sphingolipid metabolism. Biochimica et Biophysica Acta (BBA)-Molecular and Cell Biology of Lipids, 1632(1-3), 16-30.
[4] Pewzner-Jung, Y., Ben-Dor, S., Futerman, A. H. (2006). Ceramide synthases: roles in cell physiology and disease. The Journal of Biological Chemistry, 281(34), 25884-25888.
[5] Mao, C., Obeid, L. M. (2008). Ceramidases: regulators of cellular stress. Advances in Experimental Medicine and Biology, 615, 95-105.
[6] Spiegel, S., & Milstien, S. (2011). Sphingosine-1-phosphate: an enigmatic signalling lipid. Nature Reviews Molecular Cell Biology, 12(12), 758-768.
[7] Huitema, K., van den Dikkenberg, J., Bootman, M. D., & de Smedt, H. (2004). The role of ceramide synthases in sphingolipid metabolism and biology. Biochimica et Biophysica Acta (BBA)-Molecular Cell Research, 1636(1), 1-16.
[8] Saddoughi, S. A., Song, P., Ogretmen, B. (2008). Ceramide and ceramide analogs as novel cancer therapeutics. Acta Pharmacologica Sinica, 29(6), 687-700.
[9] Novgorodov, S. A., Gudz, T. I., Pomierny-Chamielec, L., et al. (2011). C24-ceramide induces autophagy in a p53-dependent manner in human colon cancer cells. Journal of Cellular Biochemistry, 112(10), 2764-2776.
[10] Cutler, R. G., Kelly, J., Storie, K., et al. (2004). Involvement of oxidative stress-induced abnormalities in ceramide and cholesterol metabolism in brain aging and Alzheimer’s disease. Proceedings of the National Academy of Sciences, 101(7), 2070-2075.
[11] Imokawa, G., Akasaki, S., Hattori, M., & Yoshizumi, M. (1991). A possible function of stratum corneum lipids in the water-holding properties of the skin. Journal of Investigative Dermatology, 96(6), 845-851.
[12] Madison, K. C. (2003). Barrier function of the skin: “la raison d’être” of the epidermis. Journal of Investigative Dermatology, 121(2), 231-241.
[13] He, X., Huang, Y., Li, B., Gong, C. X., & Schiöth, H. B. (2010). Sphingolipid metabolism in Alzheimer’s disease. Acta Neuropathologica, 120(3), 271-283.
[14] Luukkonen, P. K., Zhou, Y., Sadevirta, S., et al. (2016). Hepatic ceramides dissociate steatosis and insulin resistance in patients with non-alcoholic fatty liver disease. Journal of Hepatology, 64(5), 1167-1175.
[15] Xia, J. Y., Holland, W. L., Kusminski, C. M., et al. (2010). Targeted induction of ceramide degradation in beta-cells protects against glucolipotoxicity. Journal of Biological Chemistry, 285(35), 26493-26503.
[16] Straczkowski, M., Kowalska, I., Baranowski, M., et al. (2007). Intramyocellular lipids and insulin resistance: is it all about the muscle? American Journal of Physiology-Endocrinology and Metabolism, 293(1), E301-E309.
[17] Summers, S. A. (2016). Ceramides in insulin resistance and lipotoxicity. Progress in Lipid Research, 63, 36-49.
[18] Chavez, J. A., & Summers, S. A. (2012). A lipid basis for insulin resistance. Cell Metabolism, 15(5), 585-594.
[19] Ogretmen, B. (2018). Sphingolipid metabolism in cancer signalling and therapy. Nature Reviews Cancer, 18(2), 92-110.
[20] Haughey, N. J., Bandaru, V. V., Bae, M., Mattson, M. P., & Cutler, R. G. (2010). Involvement of altered sphingolipid metabolism in apoptosis and neuritic retraction in Alzheimer’s disease. Journal of Neurochemistry, 114(5), 1207-1217.
[21] Elias, P. M., & Steinhoff, M. (2008). “Outside-in” (environmental) triggers for itch: a common mechanistic pathway for chronic itch. Journal of Investigative Dermatology, 128(9), 2189-2192.
[22] Yang, G., Batchu, S. N., Lee, H. Y., et al. (2012). Nogo-B receptor (NgBR) is a novel regulator of de novo ceramide synthesis. The Journal of Biological Chemistry, 287(38), 32049-32061.
[23] Bajaj, K., Miller, S. A., Quick, C. M., et al. (2005). ORMDL proteins regulate ceramide synthesis by controlling serine palmitoyltransferase activity. Journal of Biological Chemistry, 280(34), 30839-30847.
[24] Holland, W. L., Knotts, T. A., Chavez, J. A., Wang, L. P., Hoehn, K. L., Goodpaster, B. H., & Summers, S. A. (2007). Ceramides mediate insulin resistance. Diabetes, 56(10), 2589-2597.
[25] Ghasemi, R., Teitelbaum, J. E., Brown, K., & Billiar, T. R. (2008). Role of ceramide in hepatocyte apoptosis and liver diseases. World Journal of Gastroenterology: WJG, 14(47), 7163.
[26] Christie, W. W. (2003). Lipid analysis: isolation, separation, identification and structural analysis of lipids. Oily Press.
[27] Byrdwell, W. C. (2001). Dual parallel chromatography with multiple detectors: comprehensive system for lipid analysis. Journal of Chromatography A, 936(1-2), 35-61.
[28] Wenk, M. R. (2005). Lipidomics. Chemistry & Biology, 12(9), 989-998.
[29] Zehethofer, S., Freytag, S., Maurer, D., Poettler, T., Zemann, B., & Koeller, D. M. (2018). High-throughput screening of enzyme-substrate interactions in sphingolipid metabolism. Scientific Reports, 8(1), 1-11.
[30] Yoshimura, S., Banno, Y., Nakashima, S., et al. (2003). Ceramide is involved in apoptosis induced by tumor necrosis factor-α in L929 cells. Journal of Biological Chemistry, 278(13), 11521-11529.
[31] McGovern, M. M., Lippa, A. M., Pastores, G. M., et al. (2017). Long-term efficacy and safety of eliglustat in patients with Gaucher disease type 1: Final results of a phase 2 clinical trial. Blood cells, molecules & diseases, 67, 78-85.
[32] French, K. J., Upson, S. E., Keller, S. N., et al. (2003). Antitumor activity of sphingosine kinase inhibitors. Journal of Pharmacology and Experimental Therapeutics, 306(3), 1185-1192.
[33] Bieber, T. (2008). Atopic dermatitis. New England Journal of Medicine, 358*(14), 1483-1494.
Given the conflicting views on ceramide levels, how might tissue-specific ceramide profiles be leveraged for targeted therapeutic interventions, rather than broad systemic modulation?
That’s a great question! Tissue-specific ceramide profiles offer a fantastic opportunity to develop therapies that are more precise. By understanding the unique ceramide landscape in different tissues, we can design interventions that address specific imbalances without causing widespread effects. This targeted approach holds immense potential for personalized medicine.
Editor: MedTechNews.Uk
Thank you to our Sponsor Esdebe
Given the often paradoxical roles of ceramides, what are the critical determinants that dictate whether ceramide modulation will result in a beneficial versus a detrimental outcome in specific disease contexts?
That’s a really insightful question! Thinking about the context-dependent effects of ceramides, it highlights the need for precise diagnostic tools. Perhaps advancements in lipidomics, allowing for detailed profiling of ceramide species in specific tissues, will be key to predicting therapeutic outcomes and avoiding unintended consequences.
Editor: MedTechNews.Uk
Thank you to our Sponsor Esdebe
The discussion of Nogo-B and ORMDL’s role in ceramide regulation is compelling. How does their interplay respond to varied cellular stressors, and could this be pharmacologically modulated to promote resilience in specific disease contexts?