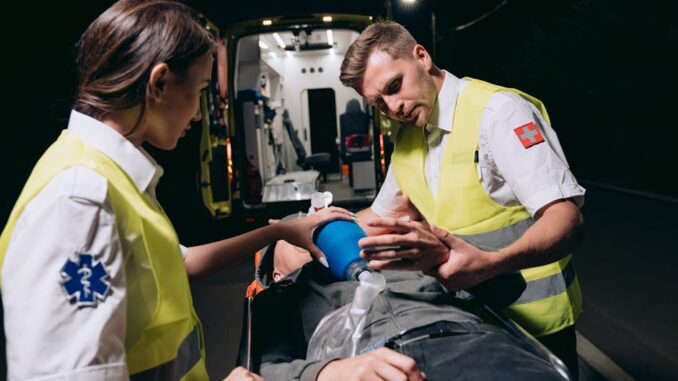
Contemporary Challenges and Future Directions in Cardiopulmonary Resuscitation: A Critical Appraisal
Abstract
Cardiopulmonary resuscitation (CPR) remains a cornerstone of emergency medical care, vital for improving survival rates following cardiac arrest. Despite decades of refinement in resuscitation guidelines, significant variability in outcomes persists. This report provides a comprehensive review of contemporary CPR practices, critically evaluating the evidence base for key interventions, exploring emerging technologies, and identifying areas for future research. We delve into the nuances of compression-ventilation ratios, the role of advanced airway management, the impact of individual patient factors on resuscitation success, and the potential of novel pharmacological and mechanical adjuncts. Furthermore, we address the importance of post-resuscitation care and strategies to mitigate long-term neurological sequelae. By highlighting current limitations and opportunities, this report aims to stimulate further investigation and innovation in the field of resuscitation science, ultimately contributing to improved patient outcomes.
1. Introduction
Cardiac arrest represents a critical medical emergency with substantial morbidity and mortality. Cardiopulmonary resuscitation (CPR) is the primary intervention aimed at restoring spontaneous circulation and mitigating irreversible organ damage. Since its inception, CPR techniques and guidelines have undergone continuous evolution, informed by ongoing research and clinical experience. While these advancements have demonstrably improved survival rates, significant challenges remain in optimizing CPR delivery and enhancing long-term patient outcomes. The inherent complexity of CPR necessitates a multifaceted approach, considering factors ranging from early recognition and bystander intervention to advanced life support and post-resuscitation care. Furthermore, the heterogeneous nature of cardiac arrest etiology and patient characteristics introduces significant variability in treatment response and prognosis. This report aims to provide a critical appraisal of current CPR practices, highlighting areas of controversy, exploring emerging technologies, and identifying key research priorities to further advance the field.
2. Evolution of CPR Guidelines and Current Recommendations
The history of CPR is marked by a progression from empirical observations to evidence-based recommendations. Early CPR techniques focused primarily on artificial respiration, with chest compressions gaining prominence in the latter half of the 20th century. The American Heart Association (AHA) and the European Resuscitation Council (ERC) have played pivotal roles in developing and disseminating CPR guidelines, which are regularly updated to reflect the latest scientific evidence [1, 2].
Current guidelines emphasize the importance of early recognition of cardiac arrest, prompt activation of emergency medical services, and immediate initiation of chest compressions. The recommended compression rate is 100-120 compressions per minute, with a compression depth of at least 5 cm (2 inches) in adults [1]. Ventilation is typically delivered via bag-valve-mask or advanced airway, with a compression-ventilation ratio of 30:2 in adults. Recent guidelines have also focused on minimizing interruptions in chest compressions, recognizing that prolonged pauses can significantly reduce coronary perfusion pressure and decrease the likelihood of successful resuscitation [3].
While these guidelines provide a standardized framework for CPR, several areas remain subject to debate. For example, the optimal compression depth and rate may vary depending on patient size and underlying comorbidities. Furthermore, the role of rescue breaths in certain scenarios, such as bystander CPR for children, is still under investigation. Additionally, translating guideline recommendations into consistent clinical practice remains a challenge, highlighting the need for improved education and training programs.
3. Compression-Ventilation Ratios: Finding the Optimal Balance
The optimal compression-ventilation ratio during CPR is a subject of ongoing investigation. While the current recommendation for a 30:2 ratio in adults is based on a balance between providing adequate ventilation and minimizing interruptions in chest compressions, alternative strategies have been proposed. Continuous chest compressions with asynchronous ventilation, also known as compression-only CPR, has emerged as a simplified approach, particularly for bystander CPR [4]. Studies have shown that compression-only CPR can be as effective as standard CPR in adults with out-of-hospital cardiac arrest, particularly when the arrest is witnessed [5]. The rationale behind this approach is that continuous chest compressions maintain coronary perfusion pressure, while passive ventilation can provide adequate oxygenation during the initial phase of resuscitation.
However, compression-only CPR may not be appropriate for all patients. Individuals with respiratory arrest or prolonged down time may benefit from rescue breaths to address underlying hypoxemia. Furthermore, the optimal compression-ventilation ratio may differ in children and infants, where respiratory causes of cardiac arrest are more common [6]. Further research is needed to determine the optimal compression-ventilation strategy for different patient populations and clinical scenarios.
4. Advanced Airway Management: Techniques and Outcomes
Securing a definitive airway is a critical step in advanced life support, facilitating oxygenation, ventilation, and protection against aspiration. Several airway management techniques are available, including bag-valve-mask ventilation, endotracheal intubation, and supraglottic airway devices. The choice of technique depends on factors such as operator skill, patient anatomy, and the presence of underlying medical conditions.
Endotracheal intubation (ETI) is considered the gold standard for airway management, providing a secure and reliable airway. However, ETI requires specialized training and can be associated with complications such as esophageal intubation, aspiration, and hemodynamic instability [7]. Supraglottic airway devices (SGAs), such as laryngeal mask airways and esophageal-tracheal combitubes, offer an alternative to ETI, particularly in situations where intubation is difficult or delayed. SGAs are relatively easy to insert and can provide adequate ventilation in most patients [8].
Recent studies have compared the effectiveness of ETI and SGAs during out-of-hospital cardiac arrest. Some trials have shown no significant difference in survival rates between the two techniques [9], while others have suggested that SGAs may be associated with improved outcomes, particularly when performed by paramedics with limited intubation experience [10]. The optimal airway management strategy remains a subject of ongoing debate, with the choice of technique likely depending on the specific clinical context and the skills of the healthcare provider.
5. Pharmacological Interventions: Current Evidence and Future Directions
Pharmacological interventions play a crucial role in advanced cardiac life support, aiming to restore spontaneous circulation and improve myocardial function. Epinephrine is the most commonly used medication during cardiac arrest, acting as an alpha-adrenergic agonist to increase peripheral vascular resistance and improve coronary perfusion pressure. While epinephrine has been shown to increase the likelihood of achieving return of spontaneous circulation (ROSC), its impact on long-term survival and neurological outcomes remains controversial [11]. Some studies have suggested that epinephrine may be associated with increased mortality and adverse neurological outcomes, particularly in patients with non-shockable rhythms [12].
Other pharmacological agents, such as amiodarone and lidocaine, are used to treat refractory ventricular fibrillation and ventricular tachycardia. These antiarrhythmic drugs can help to terminate arrhythmias and improve the likelihood of defibrillation success [13]. However, the optimal timing and dosage of these medications are still under investigation. Vasopressin, a non-adrenergic vasopressor, has also been used as an alternative to epinephrine in some cardiac arrest protocols. However, studies have shown no significant difference in outcomes between vasopressin and epinephrine [14].
Emerging research is exploring the potential of novel pharmacological agents to improve resuscitation outcomes. For example, adenosine has shown promise in treating certain types of supraventricular tachycardia that can lead to cardiac arrest. Calcium channel blockers are sometimes useful in situations such as Calcium Channel Blocker overdose. Further research is needed to evaluate the efficacy and safety of these and other emerging pharmacological interventions.
6. Mechanical Adjuncts to CPR: Enhancing Circulation and Ventilation
Mechanical adjuncts to CPR have been developed to enhance circulation and ventilation, potentially improving resuscitation outcomes. These devices include mechanical chest compression devices, impedance threshold devices, and extracorporeal membrane oxygenation (ECMO).
Mechanical chest compression devices, such as the LUCAS device and the AutoPulse, provide consistent and uninterrupted chest compressions, potentially improving coronary perfusion pressure and reducing rescuer fatigue [15]. Studies have shown that mechanical chest compression devices can be as effective as manual compressions, particularly in situations where manual compressions are difficult or impractical, such as during transport or in the angiography suite [16].
Impedance threshold devices (ITDs) are designed to increase negative intrathoracic pressure during the recoil phase of chest compressions, potentially improving venous return and cardiac output [17]. However, the evidence supporting the use of ITDs is mixed, with some studies showing improved survival rates while others have found no significant benefit [18].
Extracorporeal membrane oxygenation (ECMO) is a form of mechanical circulatory support that provides oxygenation and carbon dioxide removal, bypassing the heart and lungs. ECMO has been used in refractory cardiac arrest patients who have failed to respond to conventional CPR [19]. Studies have shown that ECMO can improve survival rates in selected patients, particularly those with reversible causes of cardiac arrest [20]. However, ECMO is an invasive procedure with significant risks, and its use is limited to specialized centers with experienced personnel.
7. Factors Affecting Resuscitation Success: Patient Characteristics and Environmental Conditions
Several factors can influence resuscitation success, including patient characteristics, environmental conditions, and the timing and quality of CPR. Patient factors such as age, underlying medical conditions, and initial cardiac rhythm can significantly impact the likelihood of achieving ROSC and long-term survival [21]. Older patients and those with pre-existing heart disease are generally less likely to survive cardiac arrest. Patients with shockable rhythms, such as ventricular fibrillation and ventricular tachycardia, have a higher chance of successful defibrillation and ROSC compared to those with non-shockable rhythms, such as asystole and pulseless electrical activity [22].
Environmental conditions, such as water temperature and submersion time in drowning victims, can also affect resuscitation outcomes. Hypothermia can protect the brain from ischemic damage, potentially improving survival rates in drowning victims with prolonged submersion [23]. However, prolonged submersion can also lead to severe hypoxemia and metabolic acidosis, which can decrease the likelihood of successful resuscitation.
The timing and quality of CPR are critical determinants of resuscitation success. Early initiation of CPR, particularly by bystanders, can significantly improve survival rates [24]. The quality of chest compressions, including the rate, depth, and minimizing interruptions, is also crucial for maintaining coronary perfusion pressure and improving the likelihood of ROSC. Furthermore, the effectiveness of advanced life support interventions, such as defibrillation and medication administration, depends on the timing and accuracy of their delivery.
8. Post-Resuscitation Care: Optimizing Neurological Outcomes
Post-resuscitation care is a critical component of the resuscitation process, aimed at preventing secondary brain injury and optimizing neurological outcomes. Following ROSC, patients are at risk for a variety of complications, including hypotension, hypoxemia, seizures, and cerebral edema. Targeted temperature management (TTM), also known as therapeutic hypothermia, is a cornerstone of post-resuscitation care, involving the induction and maintenance of mild hypothermia (32-36°C) for 24 hours [25]. TTM has been shown to improve neurological outcomes in patients who remain comatose after cardiac arrest [26].
Other important aspects of post-resuscitation care include optimizing hemodynamic stability, preventing seizures, and managing cerebral edema. Patients should be closely monitored for signs of hypotension and treated with intravenous fluids and vasopressors as needed. Anticonvulsant medications should be administered to prevent or treat seizures. Hypertonic saline or mannitol may be used to reduce cerebral edema [27].
Emerging research is exploring the potential of other neuroprotective strategies to improve neurological outcomes after cardiac arrest. These strategies include hyperbaric oxygen therapy, xenon gas inhalation, and stem cell therapy. Further research is needed to evaluate the efficacy and safety of these and other emerging neuroprotective interventions.
9. The Role of Technology in Improving Resuscitation Outcomes
Technological advancements are continuously reshaping the landscape of resuscitation, offering new tools and strategies to improve patient outcomes. Telemedicine and mobile health technologies are being used to improve early recognition of cardiac arrest and facilitate bystander CPR [28]. Wearable sensors and smartphone apps can provide real-time feedback on chest compression quality, helping bystanders to perform CPR more effectively [29].
Artificial intelligence (AI) and machine learning algorithms are being developed to analyze large datasets of cardiac arrest data, identifying patterns and predictors of resuscitation success [30]. These algorithms can be used to personalize resuscitation protocols and optimize treatment strategies for individual patients. Virtual reality (VR) and augmented reality (AR) technologies are being used to train healthcare providers in CPR and advanced life support techniques, providing a realistic and immersive learning experience [31].
Nanotechnology is also being explored for its potential applications in resuscitation. Nanoparticles can be used to deliver targeted therapies to the brain, reducing ischemic damage and improving neurological outcomes. Furthermore, nanobiosensors can be used to monitor physiological parameters in real-time, providing clinicians with valuable information to guide treatment decisions [32].
10. Conclusion and Future Directions
Cardiopulmonary resuscitation is a dynamic and evolving field, driven by ongoing research and technological innovation. While significant progress has been made in improving survival rates following cardiac arrest, substantial challenges remain in optimizing CPR delivery and enhancing long-term patient outcomes. Future research should focus on addressing the following key areas:
- Personalized CPR: Developing individualized resuscitation protocols based on patient characteristics, etiology of cardiac arrest, and real-time physiological monitoring.
- Optimizing Compression-Ventilation Ratios: Determining the optimal compression-ventilation strategy for different patient populations and clinical scenarios.
- Novel Pharmacological Interventions: Evaluating the efficacy and safety of emerging pharmacological agents to improve myocardial function and reduce ischemic damage.
- Advanced Airway Management: Comparing the effectiveness of different airway management techniques in various clinical settings.
- Mechanical Adjuncts to CPR: Investigating the role of mechanical chest compression devices, ITDs, and ECMO in improving resuscitation outcomes.
- Post-Resuscitation Care: Developing and implementing evidence-based post-resuscitation care protocols to prevent secondary brain injury and optimize neurological outcomes.
- Technology Integration: Exploring the potential of telemedicine, AI, VR, and nanotechnology to improve CPR training, delivery, and monitoring.
By addressing these challenges and embracing innovation, the field of resuscitation science can continue to advance, ultimately leading to improved survival rates and quality of life for patients who experience cardiac arrest.
References
[1] Panchal AR, Bartos JA, Cabañas JG, et al. 2020 American Heart Association Guidelines for Cardiopulmonary Resuscitation and Emergency Cardiovascular Care. Part 3: Adult Basic and Advanced Life Support. Circulation. 2020;142(16_suppl_2):S366-S468.
[2] Nolan JP, Soar J, Cariou A, et al. European Resuscitation Council Guidelines 2015: Section 3. Adult advanced life support. Resuscitation. 2015;95:100-147.
[3] Edelson DP, Abella BS, Kramer-Johansen J, et al. Effects of compression depth and pre-shock pause duration on survival after in-hospital cardiac arrest. Crit Care Med. 2006;34(4):1080-1087.
[4] Sayre MR, Cantrell J, Wilcox WC, et al. Compression-only CPR is effective in out-of-hospital cardiac arrest. Prehosp Emerg Care. 2008;12(3):279-282.
[5] Bobrow BJ, Clark LL, Ewy GA, et al. Minimally interrupted cardiac resuscitation by emergency medical services for out-of-hospital cardiac arrest. JAMA. 2008;299(10):1158-1165.
[6] Topjian AA, Raymond TT, Atkins DL, et al. Part 4: Pediatric Basic and Advanced Life Support: 2020 American Heart Association Guidelines for Cardiopulmonary Resuscitation and Emergency Cardiovascular Care. Circulation. 2020;142(16_suppl_2):S469-S523.
[7] Hasegawa K, Hiraide A, Chang Y, Brown DF. Association of prehospital advanced airway management with neurologic outcome and survival in patients with out-of-hospital cardiac arrest. JAMA. 2013;309(3):257-266.
[8] Jabre P, Combes X, Dhaouadi M, et al. Endotracheal intubation versus supraglottic airway device for out-of-hospital cardiac arrest: a randomized clinical trial. JAMA. 2018;319(22):2338-2348.
[9] Wang HE, Szydlowski V, Smith RA, et al. Association of early advanced airway management with out-of-hospital cardiac arrest survival. Emerg Med J. 2016;33(10):697-703.
[10] Gatien M, Jabre P, Monteil J, et al. Effect of multiple attempts on supraglottic airway device insertion success during out-of-hospital cardiac arrest. Resuscitation. 2019;142:51-57.
[11] Callaway CW, Gent LM, Kramer AH, et al. Epinephrine for Out-of-Hospital Cardiac Arrest: A Systematic Review and Meta-Analysis. Resuscitation. 2023;183:109674.
[12] Hagihara A, Hasegawa M, Abe T, Nagata T, Wakata Y, Miyazaki S. Prehospital epinephrine use and survival among patients with out-of-hospital cardiac arrest. JAMA. 2012;307(11):1161-1168.
[13] Kudenchuk PJ, Brown SP, Daya M, et al. Amiodarone, lidocaine, or placebo in out-of-hospital cardiac arrest after defibrillation. N Engl J Med. 2016;374(18):1715-1725.
[14] Wenzel V, Krismer AC, Arntz HR, et al. A comparison of vasopressin and epinephrine for out-of-hospital cardiopulmonary resuscitation. N Engl J Med. 2004;350(2):105-113.
[15] Rubertsson S, Lindgren E, Smekal D, et al. Mechanical chest compressions and simultaneous defibrillation vs conventional cardiopulmonary resuscitation in out-of-hospital cardiac arrest: the LUCAS study. JAMA. 2011;305(21):2167-2174.
[16] Wik L, Olsen JE, Persse D, et al. Manual vs. integrated automatic load-distributing band CPR with equal survival after out-of-hospital cardiac arrest. The randomized CIRC trial. Resuscitation. 2014;85(6):741-748.
[17] Lurie KG, Voelckel WG, Mulligan C, et al. Improving the effectiveness of open-chest CPR with an inspiratory impedance threshold valve. Circulation. 2001;104(25):2991-2995.
[18] Aufderheide TP, Frascone RJ, Wayne MA, et al. Standard cardiopulmonary resuscitation with or without an impedance threshold device. JAMA. 2011;306(20):2251-2259.
[19] Chen YS, Yu HY, Huang SC, et al. Extracorporeal membrane oxygenation support for adults with refractory cardiac arrest. J Am Coll Cardiol. 2008;51(3):317-323.
[20] Sakamoto T, Morimura N, Nagao K, et al. Extracorporeal cardiopulmonary resuscitation versus conventional cardiopulmonary resuscitation in adults with out-of-hospital cardiac arrest: a prospective observational study. Resuscitation. 2014;85(6):762-768.
[21] Daya MR, Schmicker RH, May S, Morrison LJ. Demographics and outcomes in adult out-of-hospital cardiac arrest: Implications for improving resuscitation performance. Resuscitation. 2010;81(10):1262-1268.
[22] Atwood C, Eisenberg MS, Herlitz J, Rea TD. Incidence and outcomes from out-of-hospital cardiac arrest in Europe. Resuscitation. 2005;67(1):75-80.
[23] Bierens JJLM, Knape JTA, Gelder WMDJ. Drowning. N Engl J Med. 2005;352(6):605-616.
[24] Sasson C, Rogers MAM, Dahl J, Kellermann AL. Predictors of survival from out-of-hospital cardiac arrest: a systematic review and meta-analysis. Circ Cardiovasc Qual Outcomes. 2010;3(1):63-81.
[25] Bernard SA, Gray TW, Buist MD, et al. Treatment of comatose survivors of out-of-hospital cardiac arrest with induced hypothermia. N Engl J Med. 2002;346(8):557-563.
[26] Hypothermia after Cardiac Arrest Study Group. Mild therapeutic hypothermia to improve the neurologic outcome after cardiac arrest. N Engl J Med. 2002;346(8):549-556.
[27] Oddo M, Taccone FS, Eisai H, et al. Extracranial complications of therapeutic hypothermia after cardiac arrest: a systematic review of randomized controlled trials. Crit Care Med. 2011;39(2):184-192.
[28] Ringh M, Rosenqvist M, Hollenberg J, et al. Mobile phone dispatch of laypersons for CPR in out-of-hospital cardiac arrest. N Engl J Med. 2015;373(24):2307-2315.
[29] Baldi E, Bendetto G, Brambillasca P, et al. Evaluation of a wearable system with real-time feedback to improve chest compression performance during cardiopulmonary resuscitation. Resuscitation. 2015;91:50-56.
[30] Kramer DB, Wesson PD, Leissner KB, et al. Machine learning for the prediction of survival after cardiac arrest. J Am Heart Assoc. 2019;8(6):e011068.
[31] Lioce L, Lopreiato JO, Jeong K, et al. Healthcare simulation dictionary. Agency for Healthcare Research and Quality (US); 2020 Feb.
[32] Ferrari M. Nanovectors for gene therapy. Nat Rev Genet. 2005;6(11):807-815.
This review highlights the importance of continuous advancements in CPR techniques. The mention of personalized CPR protocols, factoring in individual patient needs and real-time data, is particularly compelling. Tailoring resuscitation efforts could significantly improve outcomes.
Thanks for your comment! I agree that personalized CPR protocols hold significant promise. Imagine a future where wearable sensors automatically adjust compression depth and rate based on real-time feedback and patient-specific data. This could truly revolutionize resuscitation outcomes!
Editor: MedTechNews.Uk
Thank you to our Sponsor Esdebe
The abstract mentions variability in outcomes despite guideline refinement. How effective are current training programs in addressing the nuances of CPR delivery across diverse patient populations and varying levels of rescuer experience?
That’s a great point! The effectiveness of current CPR training in diverse populations is definitely an area needing more focus. How can we better tailor training programs to account for different demographics and rescuer skill levels, maybe through scenario-based learning addressing specific challenges?
Editor: MedTechNews.Uk
Thank you to our Sponsor Esdebe
The mention of AI and machine learning offers exciting possibilities. How might predictive algorithms, analyzing real-time data during resuscitation, dynamically adjust treatment protocols to optimize outcomes in those critical moments?