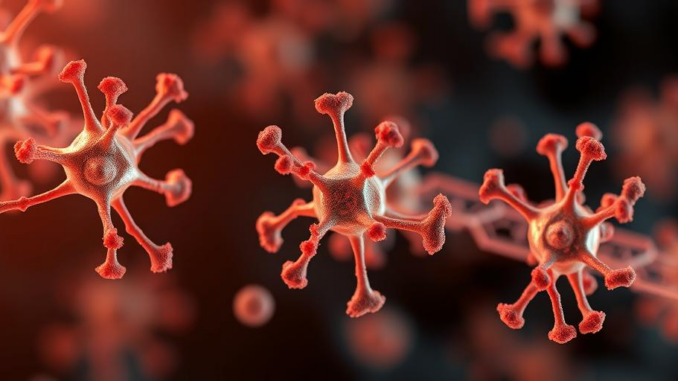
Abstract
Receptors, the gatekeepers of cellular communication, orchestrate a vast array of physiological processes by binding to diverse ligands. This report provides a comprehensive overview of the receptor landscape, encompassing major receptor families, their structural characteristics, signaling mechanisms, and roles in various physiological systems, including but not limited to pain perception. We delve into the intricacies of drug-receptor interactions, highlighting the principles of receptor-based drug discovery and the challenges associated with achieving selectivity and efficacy. Furthermore, we explore recent advancements in structural biology, computational modeling, and targeted drug delivery that are revolutionizing receptor-focused therapeutics. This review aims to provide a resource for researchers and clinicians seeking a deeper understanding of receptors as crucial targets for therapeutic intervention.
Many thanks to our sponsor Esdebe who helped us prepare this research report.
1. Introduction
Cellular communication is fundamental to life, enabling organisms to respond to their environment and maintain homeostasis. At the heart of this communication lie receptors, specialized proteins embedded in cell membranes or located intracellularly that bind to specific signaling molecules, known as ligands. This binding initiates a cascade of intracellular events, ultimately leading to a cellular response. The diversity of receptors and their signaling pathways allows for exquisite control over a wide range of physiological processes, including neurotransmission, hormone signaling, immune responses, and cell growth. Consequently, receptors represent prime targets for therapeutic intervention, and a significant proportion of currently marketed drugs exert their effects by modulating receptor activity.
This review aims to provide a comprehensive overview of the receptor landscape, covering the major receptor families, their structural features, signaling mechanisms, and roles in health and disease. We will explore the principles of drug-receptor interactions, focusing on the factors that govern ligand binding, receptor activation, and downstream signaling. Furthermore, we will examine the challenges and advancements in receptor-based drug discovery, highlighting the strategies used to identify and develop selective receptor modulators. Finally, we will discuss the emerging therapeutic potential of targeting specific receptor subtypes for the treatment of various diseases.
Many thanks to our sponsor Esdebe who helped us prepare this research report.
2. Major Receptor Families
The receptor universe is vast and diverse, encompassing a wide range of protein structures and signaling mechanisms. Receptors can be broadly classified into several major families based on their structural characteristics and signaling pathways:
2.1. G Protein-Coupled Receptors (GPCRs)
GPCRs constitute the largest and most diverse family of cell surface receptors in the human genome, comprising approximately 800 members [1]. These receptors are characterized by their seven transmembrane α-helices, connected by intracellular and extracellular loops. Upon ligand binding, GPCRs undergo a conformational change that activates intracellular heterotrimeric G proteins. G proteins, composed of α, β, and γ subunits, dissociate and modulate the activity of downstream effector proteins, such as adenylyl cyclase, phospholipase C, and ion channels. These effector proteins, in turn, generate second messengers like cyclic AMP (cAMP), inositol trisphosphate (IP3), and diacylglycerol (DAG), which amplify the signal and trigger various cellular responses.
The diversity of GPCRs and G proteins allows for a wide range of signaling pathways. GPCRs are involved in numerous physiological processes, including neurotransmission, hormone signaling, olfaction, vision, and immune responses. They are also major targets for drug development, with approximately 34% of all FDA-approved drugs acting on GPCRs [2].
Example: The β-adrenergic receptors, a subtype of GPCRs, bind to adrenaline and noradrenaline, triggering the activation of adenylyl cyclase and increasing intracellular cAMP levels. This pathway mediates the “fight-or-flight” response, leading to increased heart rate, bronchodilation, and glycogen breakdown.
2.2. Receptor Tyrosine Kinases (RTKs)
RTKs are transmembrane receptors that possess intrinsic tyrosine kinase activity. They play a crucial role in regulating cell growth, differentiation, and survival. RTKs typically consist of an extracellular ligand-binding domain, a single transmembrane helix, and an intracellular tyrosine kinase domain. Upon ligand binding, RTKs dimerize or oligomerize, leading to autophosphorylation of tyrosine residues within the intracellular domain. These phosphorylated tyrosine residues serve as docking sites for intracellular signaling proteins, such as Src homology 2 (SH2) domain-containing proteins, which activate downstream signaling pathways, including the Ras/MAPK pathway, the PI3K/Akt pathway, and the JAK/STAT pathway.
Dysregulation of RTK signaling is implicated in various cancers. Activating mutations, gene amplifications, and overexpression of RTKs can lead to uncontrolled cell growth and proliferation. Therefore, RTKs are important targets for cancer therapy, and several RTK inhibitors have been developed and approved for clinical use.
Example: The epidermal growth factor receptor (EGFR) is an RTK that binds to epidermal growth factor (EGF). Activation of EGFR leads to the activation of the Ras/MAPK pathway, promoting cell proliferation and survival. EGFR is frequently overexpressed or mutated in various cancers, making it a prime target for cancer therapy.
2.3. Ligand-Gated Ion Channels (LGICs)
LGICs are transmembrane proteins that form ion-conducting pores. These channels open or close in response to the binding of specific ligands, allowing ions to flow across the cell membrane. LGICs mediate rapid synaptic transmission in the nervous system and are involved in other physiological processes, such as muscle contraction and sensory transduction.
LGICs are typically composed of multiple subunits that assemble to form a functional channel. The ligand-binding site is located on one or more of the subunits. Upon ligand binding, the channel undergoes a conformational change that opens the pore, allowing ions to flow down their electrochemical gradient. The type of ions that flow through the channel determines the effect on the cell membrane potential. For example, the influx of positive ions, such as Na+ or Ca2+, leads to depolarization, while the influx of negative ions, such as Cl-, leads to hyperpolarization.
Example: The nicotinic acetylcholine receptor (nAChR) is an LGIC that binds to acetylcholine. Activation of the nAChR allows Na+ ions to flow into the cell, leading to depolarization and muscle contraction.
2.4. Nuclear Receptors
Nuclear receptors are intracellular receptors that regulate gene transcription. Unlike other receptor families, nuclear receptors are located in the cytoplasm or nucleus. They bind to lipophilic ligands, such as steroid hormones, thyroid hormones, and retinoids, which diffuse across the cell membrane and enter the cell. Upon ligand binding, nuclear receptors undergo a conformational change that allows them to bind to specific DNA sequences, called hormone response elements (HREs), located in the promoter regions of target genes. This binding can either activate or repress gene transcription, depending on the receptor and the specific HRE.
Nuclear receptors play a crucial role in regulating a wide range of physiological processes, including development, metabolism, reproduction, and immune responses. They are also important targets for drug development, with several nuclear receptor agonists and antagonists used to treat various diseases.
Example: The estrogen receptor (ER) is a nuclear receptor that binds to estrogen. Activation of the ER leads to the transcription of genes involved in female reproductive development and function.
2.5. Enzyme-Linked Receptors
This category includes receptors that are directly linked to enzymes or possess enzymatic activity themselves, beyond the RTKs already discussed. A prominent example is receptor guanylyl cyclases. Upon ligand binding, these receptors catalyze the conversion of GTP to cGMP, a second messenger that activates protein kinases and other downstream targets. These receptors are involved in regulating blood pressure, fluid balance, and intestinal motility. Natriuretic peptides (ANP, BNP, CNP) are examples of ligands for these receptors. Deficiencies in this pathway can lead to hypertension and heart failure.
Many thanks to our sponsor Esdebe who helped us prepare this research report.
3. Receptor Function in Physiological Processes
Receptors play a critical role in mediating a vast array of physiological processes, from basic cellular functions to complex organ system interactions. Their involvement spans nearly every aspect of human biology.
3.1. Neurotransmission
Receptors are essential for neurotransmission, the process by which neurons communicate with each other. Neurotransmitters, such as acetylcholine, dopamine, serotonin, and glutamate, bind to specific receptors on the postsynaptic neuron, triggering a change in membrane potential and initiating a new action potential. The type of receptor activated and the resulting change in membrane potential determine whether the signal is excitatory or inhibitory. This is how information is rapidly transmitted throughout the brain and body. The opioid receptors (mu, delta, kappa) mentioned in the introduction are crucial in modulating pain perception in the central nervous system. Endogenous opioid peptides, such as endorphins and enkephalins, bind to these receptors, reducing pain signals. Targeting these receptors with opioid analgesics has been a mainstay of pain management, but this approach is also associated with significant side effects, including addiction and respiratory depression. This motivates research into more selective opioid receptor modulators and alternative pain management strategies.
3.2. Hormone Signaling
Hormones, chemical messengers produced by endocrine glands, travel through the bloodstream to target cells, where they bind to specific receptors. The type of receptor activated and the resulting intracellular signaling pathway determine the cellular response. For example, insulin binds to the insulin receptor on target cells, stimulating glucose uptake and metabolism. Hormones regulate a wide range of physiological processes, including growth, development, metabolism, reproduction, and mood. Dysregulation of hormone signaling can lead to various endocrine disorders, such as diabetes, hypothyroidism, and hyperthyroidism.
3.3. Immune Responses
Receptors are crucial for the immune system to recognize and respond to pathogens. Immune cells, such as T cells, B cells, and macrophages, express a variety of receptors that recognize specific antigens, molecules derived from pathogens. Upon antigen binding, these receptors activate intracellular signaling pathways that trigger immune responses, such as antibody production, T cell activation, and inflammation. Cytokine receptors are another class of immune receptors. These bind to cytokines, which are signaling molecules that regulate immune cell function. Dysregulation of immune receptor signaling can lead to autoimmune diseases, such as rheumatoid arthritis and lupus, and immunodeficiency disorders, such as HIV/AIDS.
3.4. Sensory Perception
Receptors are essential for sensory perception, allowing us to detect and respond to stimuli from the environment. Sensory receptors, located in specialized sensory organs, transduce physical or chemical stimuli into electrical signals that are transmitted to the brain. For example, photoreceptors in the eye detect light, mechanoreceptors in the skin detect touch, and chemoreceptors in the nose and tongue detect smell and taste. The type of receptor activated and the resulting signal transduction pathway determine the type of sensation perceived.
3.5. Pain Perception
Pain perception involves a complex interplay of receptors and signaling pathways. Nociceptors, specialized sensory neurons that detect noxious stimuli, express a variety of receptors that respond to different pain-inducing agents. These receptors include transient receptor potential (TRP) channels, which are activated by heat, cold, and inflammatory mediators; purinergic receptors, which are activated by ATP released from damaged cells; and bradykinin receptors, which are activated by bradykinin, an inflammatory peptide. Activation of these receptors leads to the transmission of pain signals to the brain, where they are processed and interpreted.
As noted previously, opioid receptors play a key modulatory role in pain. While crucial, reliance on opioid-based analgesics has led to a significant public health crisis. Thus, research is now actively focused on identifying new targets within the pain pathway. For example, targeting specific TRP channels offers a potential alternative approach to pain management. Blockers of TRPV1 (capsaicin receptor) are being investigated for treatment of neuropathic pain and inflammatory pain. Another active area of investigation involves the development of antagonists for CGRP (calcitonin gene-related peptide) receptors, which are implicated in migraine pathogenesis.
Many thanks to our sponsor Esdebe who helped us prepare this research report.
4. Mechanisms of Drug-Receptor Interactions
The interaction between a drug and its target receptor is governed by a variety of factors, including the chemical structure of the drug, the shape and charge distribution of the receptor binding site, and the surrounding environment. Understanding these factors is crucial for designing drugs that bind to their target receptors with high affinity and selectivity.
4.1. Binding Affinity and Selectivity
Binding affinity refers to the strength of the interaction between a drug and its receptor. It is typically measured by the dissociation constant (Kd), which represents the concentration of drug required to occupy 50% of the receptors at equilibrium. A lower Kd value indicates a higher binding affinity.
Selectivity refers to the ability of a drug to bind to its target receptor with a higher affinity than to other receptors. Selectivity is crucial for minimizing off-target effects and improving the therapeutic index of a drug. Achieving high selectivity is a major challenge in drug discovery, as many receptors share structural similarities and bind to similar ligands. Rational drug design, based on detailed structural information about the target receptor, is increasingly used to improve selectivity. Furthermore, kinetic selectivity, where a drug binds with similar affinity to multiple targets but has significantly different residence times on each, is emerging as an important design principle.
4.2. Receptor Activation and Signaling
Upon binding to its receptor, a drug can either activate or inhibit the receptor. Agonists are drugs that activate receptors, mimicking the effect of the endogenous ligand. Antagonists are drugs that bind to receptors but do not activate them, blocking the binding of the endogenous ligand and preventing receptor activation. Partial agonists are drugs that bind to receptors and activate them, but with a lower efficacy than the endogenous ligand.
The mechanism of receptor activation varies depending on the type of receptor. For example, GPCR activation involves a conformational change that allows the receptor to interact with G proteins, while RTK activation involves receptor dimerization and autophosphorylation. The downstream signaling pathways activated by a drug determine the cellular response. Biased agonism, where a drug selectively activates only a subset of signaling pathways downstream of a receptor, is a particularly active area of research. This allows for the development of drugs that can achieve desired therapeutic effects while minimizing unwanted side effects.
4.3. Allosteric Modulation
Allosteric modulators are drugs that bind to a receptor at a site distinct from the orthosteric (ligand-binding) site. Allosteric modulators can either enhance (positive allosteric modulators, or PAMs) or inhibit (negative allosteric modulators, or NAMs) the activity of the receptor. Allosteric modulation offers several advantages over orthosteric modulation, including the potential for greater selectivity and reduced risk of desensitization. Because allosteric sites are generally less conserved than orthosteric sites, PAMs and NAMs can exhibit greater selectivity for receptor subtypes. Additionally, because allosteric modulators typically require the presence of the endogenous ligand to exert their effects, they are less likely to cause receptor desensitization.
4.4. Receptor Desensitization and Downregulation
Prolonged exposure to an agonist can lead to receptor desensitization, a decrease in receptor responsiveness. Desensitization can occur through various mechanisms, including receptor phosphorylation, internalization, and downregulation. Receptor phosphorylation can reduce the ability of the receptor to interact with downstream signaling proteins. Receptor internalization involves the removal of receptors from the cell surface via endocytosis. Receptor downregulation involves a decrease in the total number of receptors expressed by the cell. Understanding the mechanisms of receptor desensitization is crucial for developing drugs that maintain their efficacy over time.
Many thanks to our sponsor Esdebe who helped us prepare this research report.
5. Therapeutic Potential of Targeting Specific Receptors
Targeting specific receptors for therapeutic purposes has revolutionized the treatment of many diseases. By selectively modulating the activity of specific receptors, drugs can achieve desired therapeutic effects with minimal off-target effects.
5.1. GPCRs as Drug Targets
GPCRs are the most successful class of drug targets, with approximately 34% of all FDA-approved drugs acting on GPCRs. GPCR-targeting drugs are used to treat a wide range of diseases, including hypertension, asthma, depression, pain, and Parkinson’s disease. The success of GPCRs as drug targets is due to their large number, their involvement in a wide range of physiological processes, and their amenability to drug modulation.
5.2. RTKs as Drug Targets
RTKs are important targets for cancer therapy, and several RTK inhibitors have been developed and approved for clinical use. These inhibitors work by blocking the tyrosine kinase activity of RTKs, preventing the activation of downstream signaling pathways that promote cell growth and proliferation. RTK inhibitors have shown significant clinical benefit in the treatment of various cancers, including lung cancer, breast cancer, and leukemia.
5.3. LGICs as Drug Targets
LGICs are important targets for drugs that treat neurological and psychiatric disorders. For example, benzodiazepines, which are used to treat anxiety and insomnia, bind to the GABA(A) receptor, an LGIC that mediates inhibitory neurotransmission. Anticonvulsants, which are used to treat epilepsy, target various LGICs, including the GABA(A) receptor and the glutamate receptor.
5.4. Nuclear Receptors as Drug Targets
Nuclear receptors are important targets for drugs that treat endocrine disorders, metabolic disorders, and cancer. For example, selective estrogen receptor modulators (SERMs), such as tamoxifen, are used to treat breast cancer by blocking the effects of estrogen on breast cancer cells. PPAR agonists, such as thiazolidinediones, are used to treat type 2 diabetes by improving insulin sensitivity.
5.5. Targeted Therapies and Personalized Medicine
The advancement of genomic technologies has enabled a more personalized approach to receptor-based drug therapy. Identifying genetic variations in receptors that affect drug response can help predict which patients are most likely to benefit from a particular drug. For example, genetic variations in the CYP2C19 gene, which encodes an enzyme that metabolizes clopidogrel (an antiplatelet drug that acts on the P2Y12 receptor), can affect the effectiveness of clopidogrel. By genotyping patients for CYP2C19 variants, clinicians can identify patients who are at risk of clopidogrel resistance and prescribe alternative antiplatelet drugs.
Many thanks to our sponsor Esdebe who helped us prepare this research report.
6. Challenges and Advancements in Receptor-Based Drug Discovery
Receptor-based drug discovery is a complex and challenging process. Several factors can hinder the development of successful receptor-targeting drugs, including the difficulty of achieving high selectivity, the potential for receptor desensitization, and the challenges of developing drugs that can cross the blood-brain barrier. However, recent advancements in structural biology, computational modeling, and targeted drug delivery are revolutionizing receptor-focused therapeutics.
6.1. Structural Biology and Cryo-EM
Determining the three-dimensional structure of receptors is crucial for understanding their function and for designing drugs that bind to them with high affinity and selectivity. X-ray crystallography has been the traditional method for determining protein structures. Cryo-electron microscopy (cryo-EM) is rapidly emerging as a powerful tool for determining the structures of receptors, particularly membrane proteins, which are often difficult to crystallize. Cryo-EM allows researchers to determine the structures of receptors in their native state, providing valuable insights into their function and drug-binding properties.
6.2. Computational Modeling and Virtual Screening
Computational modeling and virtual screening are increasingly used to accelerate the drug discovery process. Computational modeling can be used to predict the binding affinity and selectivity of drugs to receptors, as well as to identify potential drug candidates. Virtual screening involves the use of computer algorithms to screen large libraries of chemical compounds for their ability to bind to a target receptor. These techniques reduce the time and cost associated with traditional drug discovery methods.
6.3. Targeted Drug Delivery
Targeted drug delivery systems can improve the efficacy and reduce the toxicity of receptor-targeting drugs. Targeted drug delivery systems involve the use of nanoparticles or other carriers to deliver drugs specifically to target cells or tissues. This approach can increase the concentration of drug at the target site, while minimizing exposure to other tissues. Examples of targeted drug delivery systems include liposomes, nanoparticles, and antibody-drug conjugates.
6.4. Overcoming the Blood-Brain Barrier (BBB)
Developing drugs that can cross the BBB is a major challenge for the treatment of neurological and psychiatric disorders. The BBB is a highly selective barrier that protects the brain from harmful substances in the blood. Several strategies are being developed to overcome the BBB, including the use of nanoparticles that can be actively transported across the BBB and the development of prodrugs that are converted to active drugs within the brain.
Many thanks to our sponsor Esdebe who helped us prepare this research report.
7. Conclusion
Receptors are essential for cellular communication and play a critical role in a wide range of physiological processes. Understanding the structure, function, and regulation of receptors is crucial for developing effective therapies for various diseases. Recent advancements in structural biology, computational modeling, and targeted drug delivery are revolutionizing receptor-based drug discovery, paving the way for the development of more selective and effective receptor-targeting drugs. The future of receptor-based therapeutics lies in personalized medicine, where genetic and other biomarkers are used to tailor drug therapy to individual patients. Understanding the full receptor landscape provides the foundation for designing novel therapeutics and improving the health and well-being of individuals worldwide.
Many thanks to our sponsor Esdebe who helped us prepare this research report.
References
[1] Fredriksson R, Lagerström MC, Lundin D, Schiöth HB. The G-protein-coupled receptors in the human genome are non-uniformly distributed. Trends Pharmacol Sci. 2003;24(8):408-12.
[2] Hauser AS, Attwood MM, Rask-Andersen M, Schiöth HB, Gloriam DE. Trends in GPCR drug discovery: new agents, targets and indications. Nat Rev Drug Discov. 2017;16(12):829-842.
[3] Kobilka BK. Structural biology of G protein-coupled receptors. Annu Rev Pharmacol Toxicol. 2013;53:1-16.
[4] Lemmon MA, Schlessinger J. Cell signaling by receptor tyrosine kinases. Cell. 2010;141(7):1117-34.
[5] Albuquerque EX, Pereira EF, Alkondon M, Rogers SW. Mammalian nicotinic acetylcholine receptors: from structure to function. Physiol Rev. 2009;89(1):73-120.
[6] Mangelsdorf DJ, Evans RM. The pharmaceutical potential of nuclear receptors. Science. 1995;268(5215):1194-9.
[7] Julius D. TRP channels and pain. Annu Rev Cell Dev Biol. 2013;29:355-84.
[8] G protein-Coupled Receptors as Drug Targets. ScienceSignaling, https://www.sciencesignaling.org/content/sigtrans/10/498/eaah6343.full
[9] Roth, B.L., Kroeze, W.K., Patel, S. et al. The promises and perils of molecularly targeted therapeutics. Nat Rev Drug Discov 3, 353–366 (2004). https://doi.org/10.1038/nrd1367
[10] Lounnas V, Rognan D. Rational design of ligands targeting transmembrane receptors. Curr Top Med Chem. 2002;2(12):1269-82.
[11] Kenakin T. Functional Selectivity and Biased Receptor Signaling in Drug Discovery. Expert Opin Drug Discov. 2010;5(7):625-641.
[12] Christopoulos A. Allosteric drug targets: current status and future directions. Nat Rev Drug Discov. 2002;1(3):198-210.
[13] Beaulieu JM, Gainetdinov RR. The physiology, signaling, and pharmacology of dopamine receptors. Pharmacol Rev. 2011;63(1):182-217.
[14] Dancey J. Targeting receptor tyrosine kinases: the example of EGFR. Curr Opin Pharmacol. 2002;2(4):391-7.
[15] Nestler EJ. Molecular mechanisms of drug addiction. Neuropharmacology. 2005;47(3):24-37.
[16] Pardridge WM. Blood-brain barrier drug targeting: the future of brain drug development. Mol Interv. 2003;3(2):90-105.
This review effectively highlights the significance of receptor-based drug discovery. Given the challenges of crossing the blood-brain barrier, could future research explore novel strategies like focused ultrasound to enhance drug delivery specifically to targeted brain regions?
That’s a great point! Focused ultrasound is indeed a promising avenue for enhancing drug delivery across the blood-brain barrier. It allows for targeted and localized delivery, potentially improving treatment efficacy for neurological disorders. Future studies combining receptor-targeted drugs with focused ultrasound could be very impactful. Thanks for raising this important area!
Editor: MedTechNews.Uk
Thank you to our Sponsor Esdebe
This is an excellent review of receptor-based drug discovery! Given the challenges of achieving drug selectivity, could you elaborate on the potential of PROTACs (proteolysis-targeting chimeras) to selectively degrade target receptors, offering a novel therapeutic avenue?
Thanks for the insightful comment! PROTACs are definitely an exciting area. Their potential to selectively degrade target receptors, rather than just inhibiting them, offers a unique approach to overcoming some selectivity challenges. This could lead to more targeted and effective therapies with fewer off-target effects. It’s a complex field, but the potential is huge!
Editor: MedTechNews.Uk
Thank you to our Sponsor Esdebe
The discussion of allosteric modulation is particularly interesting. The potential for increased receptor subtype selectivity and reduced desensitization compared to orthosteric modulation could lead to novel therapeutic strategies.
Thanks! Allosteric modulation does indeed open exciting doors. The enhanced selectivity is a real game-changer, and the reduced desensitization could lead to more durable therapeutic effects. Exploring allosteric modulators of different receptor families seems very promising!
Editor: MedTechNews.Uk
Thank you to our Sponsor Esdebe
The review’s discussion of personalized medicine and genetic variations affecting drug response is crucial. Exploring the ethical considerations and potential disparities in access to such personalized therapies would be valuable for a comprehensive understanding.