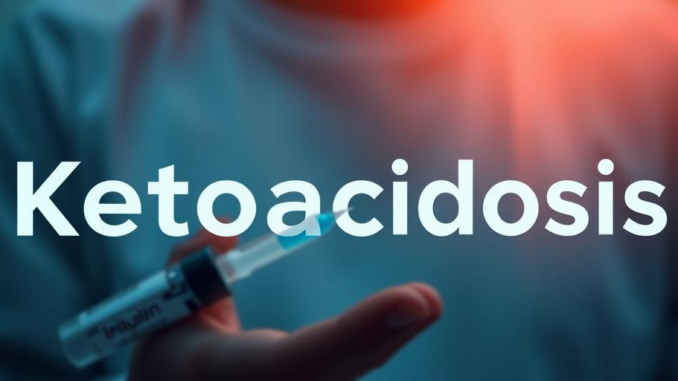
Abstract
Diabetic ketoacidosis (DKA) remains a significant acute complication of diabetes mellitus, particularly in individuals with type 1 diabetes (T1D). While advancements in diabetes management, such as continuous glucose monitoring (CGM) and insulin pump therapy, have improved glycemic control, they have also introduced new challenges, including an increased risk of DKA under certain circumstances. This review provides a comprehensive overview of DKA, encompassing its underlying pathophysiology, precipitating factors, clinical presentation, diagnostic criteria, treatment protocols, and preventive strategies. Furthermore, it delves into the long-term health consequences of recurrent DKA episodes, including potential neurological sequelae and impacts on renal function. Special attention is given to the role of hybrid closed-loop (HCL) systems in DKA development and strategies for optimizing their use to minimize risk. This review also explores novel therapeutic targets and emerging technologies aimed at improving DKA management and preventing its recurrence.
Many thanks to our sponsor Esdebe who helped us prepare this research report.
1. Introduction
Diabetic ketoacidosis (DKA) is a severe metabolic derangement characterized by hyperglycemia, ketonemia, and metabolic acidosis. This life-threatening condition arises from an absolute or relative insulin deficiency coupled with an excess of counter-regulatory hormones, such as glucagon, catecholamines, cortisol, and growth hormone. The resulting metabolic state leads to unrestrained lipolysis, ketogenesis, and gluconeogenesis, overwhelming the body’s buffering capacity and resulting in a cascade of physiological abnormalities. DKA predominantly affects individuals with type 1 diabetes (T1D), where the autoimmune destruction of pancreatic beta cells leads to absolute insulin deficiency. However, it can also occur in individuals with type 2 diabetes (T2D), particularly under conditions of severe physiological stress, such as infection or surgery, or in those with latent autoimmune diabetes in adults (LADA). Recent advancements in diabetes technology, including continuous subcutaneous insulin infusion (CSII) and continuous glucose monitoring (CGM), have revolutionized diabetes management, providing improved glycemic control and enhanced quality of life. However, these technologies are not without their challenges. Notably, reports have emerged suggesting that hybrid closed-loop (HCL) systems, while generally effective, can paradoxically increase the risk of DKA under specific circumstances, such as pump malfunction or infusion site failure [1]. This review aims to provide a comprehensive overview of DKA, examining its underlying pathophysiology, risk factors, clinical presentation, diagnostic criteria, treatment protocols, and preventive strategies, with a particular focus on the complexities introduced by modern diabetes technologies and the potential for long-term complications. Furthermore, the report explores potential future treatment options.
Many thanks to our sponsor Esdebe who helped us prepare this research report.
2. Pathophysiology of DKA
The pathophysiology of DKA is complex and involves a series of interconnected metabolic disturbances initiated by insulin deficiency and counter-regulatory hormone excess. The central event is the inability of glucose to enter cells for energy production, leading to cellular starvation despite hyperglycemia. This triggers a cascade of metabolic adaptations aimed at providing alternative fuel sources, but these adaptations ultimately exacerbate the metabolic imbalance.
2.1 Insulin Deficiency and Counter-Regulatory Hormone Excess
Insulin plays a critical role in regulating glucose metabolism, inhibiting lipolysis, and suppressing ketogenesis. In the absence of sufficient insulin, glucose cannot be effectively transported into cells, leading to hyperglycemia. Simultaneously, the lack of insulin’s inhibitory effect on lipolysis results in the breakdown of triglycerides into free fatty acids (FFAs) and glycerol. The elevated FFAs are then transported to the liver, where they undergo beta-oxidation. Counter-regulatory hormones, such as glucagon, epinephrine, cortisol, and growth hormone, further amplify these metabolic processes. Glucagon stimulates glycogenolysis (breakdown of glycogen) and gluconeogenesis (synthesis of glucose from non-carbohydrate sources) in the liver, contributing to hyperglycemia. Epinephrine and cortisol enhance lipolysis, providing more FFAs for ketone body production. Together, these hormonal imbalances create a state of profound metabolic dysregulation [2].
2.2 Ketogenesis and Metabolic Acidosis
In the liver, FFAs are metabolized to acetyl-CoA, which can enter the Krebs cycle for energy production. However, when the Krebs cycle is overwhelmed by the excessive amount of acetyl-CoA, it is shunted towards ketogenesis. Ketogenesis is the process of producing ketone bodies, including acetoacetate, beta-hydroxybutyrate, and acetone. Acetoacetate and beta-hydroxybutyrate are organic acids that dissociate in solution, releasing hydrogen ions (H+). The accumulation of these hydrogen ions leads to a decrease in blood pH, resulting in metabolic acidosis. Acetone, a volatile ketone body, is exhaled through the lungs, contributing to the characteristic fruity odor on the breath of individuals in DKA. The body attempts to buffer the excess hydrogen ions using bicarbonate, but as bicarbonate stores are depleted, the acidosis worsens [3].
2.3 Hyperglycemia and Osmotic Diuresis
The persistent hyperglycemia in DKA exceeds the renal threshold for glucose reabsorption, resulting in glucosuria (glucose in the urine). Glucose in the urine acts as an osmotic diuretic, drawing water and electrolytes into the urine. This osmotic diuresis leads to dehydration, electrolyte imbalances (including sodium, potassium, chloride, and phosphate), and a reduction in blood volume. The dehydration further exacerbates hyperglycemia by concentrating the glucose in the remaining blood volume. Potassium loss, though not always reflected in serum potassium levels due to intracellular shifts, is a significant concern, as rapid correction of acidosis can lead to severe hypokalemia [4].
2.4 Electrolyte Imbalances
As outlined, multiple electrolyte imbalances occur in DKA. Sodium is lost through osmotic diuresis. While serum potassium may initially be normal or even elevated due to intracellular shifting caused by acidosis, the total body potassium is significantly depleted. As acidosis is corrected with insulin therapy, potassium shifts back into cells, potentially leading to life-threatening hypokalemia if not carefully monitored and supplemented. Phosphate is also lost through osmotic diuresis and can lead to hypophosphatemia during treatment, which can impair cellular function and oxygen delivery. Chloride imbalances can also occur due to vomiting and dehydration [5].
Many thanks to our sponsor Esdebe who helped us prepare this research report.
3. Risk Factors for DKA
Several factors can precipitate DKA, particularly in individuals with T1D. These risk factors can be broadly categorized as follows:
3.1 Missed or Inadequate Insulin Doses
This is the most common precipitating factor for DKA. Omitting or reducing insulin doses, intentionally or unintentionally, can lead to a rapid rise in blood glucose levels and a subsequent cascade of metabolic events leading to DKA. This can occur due to factors such as non-adherence to insulin regimens, fear of hypoglycemia, or difficulty managing insulin doses during periods of illness or stress [6].
3.2 Infections
Infections trigger the release of counter-regulatory hormones, such as cortisol and epinephrine, which exacerbate insulin resistance and increase glucose production. Infections also increase metabolic demands, further stressing the body’s ability to maintain glucose homeostasis. Common infections that can precipitate DKA include pneumonia, urinary tract infections, and gastroenteritis [7].
3.3 Insulin Pump Malfunctions
Insulin pumps deliver a continuous basal rate of insulin and bolus doses for meals. Malfunctions, such as pump occlusion, tubing kinks, or battery failure, can interrupt insulin delivery, leading to a rapid onset of DKA, particularly if not detected promptly. Users of HCL systems might experience DKA because of malfunction, as they can lead to a false sense of security, as the pump is supposed to manage the insulin levels [8].
3.4 Other Medical Conditions and Stress
Conditions such as myocardial infarction, stroke, pancreatitis, and trauma can trigger DKA. These conditions are associated with increased levels of stress hormones, which promote insulin resistance and glucose production. Psychological stress can also contribute to DKA by affecting insulin adherence and increasing counter-regulatory hormone release [9].
3.5 Eating Disorders
Eating disorders, such as anorexia nervosa and bulimia nervosa, can disrupt insulin management and lead to DKA. Restricting food intake or intentionally omitting insulin doses to induce weight loss can trigger severe metabolic imbalances [10].
3.6 Substance Abuse
Substance abuse, particularly alcohol and illicit drugs, can impair insulin sensitivity and increase the risk of DKA. Alcohol can interfere with glucose metabolism and promote ketogenesis. Certain drugs, such as cocaine, can stimulate the release of counter-regulatory hormones [11].
3.7 HCL Systems and Associated Risks
While HCL systems represent a significant advance in diabetes management, they are not without their limitations and associated risks. Infusion site failure, due to lipohypertrophy or infection, can disrupt insulin delivery, leading to DKA. Algorithm errors or sensor inaccuracies can also contribute to suboptimal insulin delivery and increased DKA risk. Furthermore, user error, such as incorrect carbohydrate counting or failure to respond to system alerts, can compromise the effectiveness of HCL systems and increase DKA risk. Newer systems with enhanced safety features, such as automated occlusion detection and predictive algorithms, are being developed to mitigate these risks [12].
Many thanks to our sponsor Esdebe who helped us prepare this research report.
4. Clinical Presentation and Diagnosis
The clinical presentation of DKA can vary depending on the severity of the metabolic derangement and the individual’s underlying medical condition. Common signs and symptoms include:
4.1 Classic Symptoms
- Polyuria: Increased urination due to osmotic diuresis.
- Polydipsia: Increased thirst due to dehydration.
- Polyphagia: Increased hunger due to cellular starvation.
- Nausea and Vomiting: Caused by ketonemia and acidosis.
- Abdominal Pain: Can be severe and mimic acute abdominal conditions.
- Weakness and Fatigue: Resulting from dehydration and electrolyte imbalances.
- Fruity Odor on Breath: Due to acetone exhalation.
- Kussmaul Respirations: Deep, rapid breathing to compensate for metabolic acidosis.
- Altered Mental Status: Ranging from confusion to coma [13].
4.2 Diagnostic Criteria
The diagnosis of DKA is based on a combination of clinical and laboratory findings. The American Diabetes Association (ADA) diagnostic criteria for DKA include:
- Hyperglycemia: Blood glucose > 250 mg/dL (13.9 mmol/L).
- Ketonemia: Serum ketones present or urine ketones moderate to large.
- Metabolic Acidosis: Arterial pH < 7.3 or bicarbonate < 18 mEq/L.
- Anion Gap: > 10 mEq/L (calculated as [Na+] – [Cl-] – [HCO3-]) [14].
4.3 Differential Diagnosis
It is important to differentiate DKA from other conditions that can present with similar symptoms, such as:
- Hyperosmolar Hyperglycemic State (HHS): HHS is characterized by severe hyperglycemia (> 600 mg/dL) and hyperosmolality (> 320 mOsm/kg) without significant ketosis or acidosis. It is more common in individuals with T2D [15].
- Alcoholic Ketoacidosis (AKA): AKA occurs in individuals with chronic alcohol abuse and is characterized by ketosis and acidosis but typically without significant hyperglycemia. It is often precipitated by alcohol withdrawal and starvation [16].
- Starvation Ketoacidosis: Similar to AKA, starvation ketoacidosis occurs in individuals with prolonged starvation and is characterized by ketosis and acidosis without significant hyperglycemia [17].
- Lactic Acidosis: Lactic acidosis can be caused by various conditions, including sepsis, shock, and certain medications. It is characterized by elevated lactate levels and metabolic acidosis [18].
Many thanks to our sponsor Esdebe who helped us prepare this research report.
5. Treatment of DKA
The treatment of DKA involves a multifaceted approach aimed at correcting hyperglycemia, reversing ketosis and acidosis, restoring fluid and electrolyte balance, and identifying and treating any underlying precipitating factors. The key components of DKA treatment include:
5.1 Fluid Resuscitation
Fluid resuscitation is the initial step in DKA treatment to correct dehydration and restore blood volume. Isotonic saline (0.9% NaCl) is the preferred initial fluid. The rate of fluid administration depends on the severity of dehydration and the individual’s cardiovascular status. Typically, 1-2 liters of isotonic saline are administered in the first hour, followed by a maintenance infusion of 250-500 mL/hour [19].
5.2 Insulin Therapy
Insulin therapy is essential to suppress ketogenesis and promote glucose utilization. A continuous intravenous infusion of regular insulin is typically used. An initial bolus of 0.1 units/kg is often administered, followed by a continuous infusion of 0.1 units/kg/hour. Blood glucose levels should be monitored hourly, and the insulin infusion rate adjusted to gradually reduce blood glucose to a target range of 150-200 mg/dL. Once blood glucose reaches this range, the insulin infusion rate can be reduced, and dextrose-containing fluids (D5W or D10W) can be added to prevent hypoglycemia [20].
5.3 Electrolyte Replacement
Electrolyte replacement is crucial to correct electrolyte imbalances and prevent complications. Potassium replacement is particularly important, as rapid correction of acidosis can lead to hypokalemia. Potassium chloride (KCl) is typically administered intravenously, guided by frequent monitoring of serum potassium levels. Phosphate replacement may also be necessary, particularly in individuals with severe hypophosphatemia, to prevent complications such as respiratory muscle weakness and cardiac arrhythmias. Sodium and chloride imbalances are usually corrected with fluid resuscitation [21].
5.4 Bicarbonate Therapy
Bicarbonate therapy is generally not recommended for most cases of DKA, as it has not been shown to improve outcomes and may increase the risk of complications, such as cerebral edema and hypokalemia. However, bicarbonate therapy may be considered in individuals with severe acidosis (pH < 6.9) to improve cardiac function and prevent respiratory failure [22].
5.5 Monitoring and Supportive Care
Close monitoring of vital signs, blood glucose levels, electrolytes, arterial blood gases, and urine output is essential throughout DKA treatment. Supportive care, such as antiemetics for nausea and vomiting, and pain management for abdominal pain, should also be provided. Identifying and treating any underlying precipitating factors, such as infections, is crucial to prevent DKA recurrence [23].
5.6 Transition to Subcutaneous Insulin
Once the patient is able to tolerate oral intake, the acidosis has resolved (pH > 7.3 and bicarbonate > 18 mEq/L), and the anion gap has closed, the transition to subcutaneous insulin can begin. A long-acting basal insulin should be administered, and bolus insulin doses should be given before meals. The intravenous insulin infusion should be continued for 1-2 hours after the first subcutaneous insulin injection to ensure adequate insulin coverage [24].
Many thanks to our sponsor Esdebe who helped us prepare this research report.
6. Prevention of DKA
The prevention of DKA requires a proactive and multifaceted approach, focusing on patient education, adherence to insulin regimens, prompt recognition of early warning signs, and effective management of risk factors. Key preventive strategies include:
6.1 Patient Education
Comprehensive patient education is essential to empower individuals with diabetes to effectively manage their condition and prevent DKA. Education should cover the following topics:
- Insulin Administration Techniques: Proper insulin injection or pump site management techniques to ensure optimal insulin absorption and delivery.
- Blood Glucose Monitoring: Regular blood glucose monitoring and interpretation of results to detect early signs of hyperglycemia.
- Ketone Testing: When and how to test for ketones, particularly during periods of illness or stress.
- Sick Day Management: Strategies for managing diabetes during illness, including adjusting insulin doses, increasing fluid intake, and monitoring blood glucose and ketones more frequently.
- Insulin Pump Troubleshooting: Basic troubleshooting techniques for insulin pump malfunctions, such as occlusion or battery failure.
- Recognition of DKA Symptoms: Early recognition of DKA symptoms, such as polyuria, polydipsia, nausea, vomiting, and abdominal pain.
- Emergency Contact Information: Having readily available contact information for healthcare providers and emergency services [25].
6.2 Adherence to Insulin Regimens
Adherence to prescribed insulin regimens is critical for preventing DKA. Strategies to improve adherence include:
- Simplifying Insulin Regimens: Working with healthcare providers to develop insulin regimens that are easy to follow and fit the individual’s lifestyle.
- Using Reminders: Utilizing reminders, such as alarms or mobile apps, to prompt insulin injections or pump site changes.
- Addressing Barriers to Adherence: Identifying and addressing barriers to adherence, such as fear of hypoglycemia, financial constraints, or lack of social support [26].
6.3 Prompt Recognition and Management of Risk Factors
Prompt recognition and management of risk factors, such as infections, insulin pump malfunctions, and stress, can help prevent DKA. Strategies include:
- Early Treatment of Infections: Seeking prompt medical attention for infections and adhering to prescribed antibiotic regimens.
- Regular Insulin Pump Checks: Regularly checking insulin pumps for proper function and replacing infusion sets as recommended.
- Stress Management Techniques: Practicing stress management techniques, such as exercise, meditation, or counseling, to reduce counter-regulatory hormone release [27].
6.4 Optimizing HCL System Use
For individuals using HCL systems, optimizing system use is crucial to minimize DKA risk. Strategies include:
- Proper Infusion Site Management: Rotating infusion sites regularly to prevent lipohypertrophy and infection.
- Accurate Carbohydrate Counting: Accurately estimating carbohydrate intake to ensure appropriate insulin bolus doses.
- Responding to System Alerts: Promptly responding to system alerts, such as sensor errors or occlusion warnings.
- Regular System Audits: Periodically reviewing HCL system data with healthcare providers to identify potential issues and optimize settings.
Newer HCL systems with improved algorithms and safety features are continuously being developed to further reduce DKA risk [28].
Many thanks to our sponsor Esdebe who helped us prepare this research report.
7. Long-Term Health Implications of Recurrent DKA
Recurrent DKA episodes can have significant long-term health consequences, affecting various organ systems and potentially impacting quality of life. While acute DKA episodes are well-managed with current protocols, the long-term consequences of frequent DKA are less studied. The primary areas of concern are:
7.1 Neurological Sequelae
Recurrent DKA episodes can lead to subtle but significant neurological deficits. Cerebral edema, although rare, is a life-threatening complication of DKA, particularly in children. Even without overt cerebral edema, repeated episodes of severe hyperglycemia and acidosis can cause neuronal damage and cognitive impairment. Studies have shown that individuals with a history of recurrent DKA may experience difficulties with attention, memory, and executive function [29]. The exact mechanisms underlying these neurological sequelae are not fully understood but may involve oxidative stress, inflammation, and disruption of neuronal metabolism.
7.2 Renal Dysfunction
DKA can place significant stress on the kidneys, potentially leading to long-term renal dysfunction. Osmotic diuresis and dehydration can cause acute kidney injury (AKI), which, if repeated, can lead to chronic kidney disease (CKD). Furthermore, the metabolic acidosis associated with DKA can exacerbate existing renal damage. Studies have shown that individuals with a history of recurrent DKA have a higher risk of developing albuminuria and reduced glomerular filtration rate, both markers of CKD [30].
7.3 Cardiovascular Complications
DKA is associated with increased cardiovascular risk. The metabolic stress and electrolyte imbalances associated with DKA can trigger arrhythmias and exacerbate underlying cardiac conditions. Furthermore, the inflammation and oxidative stress associated with DKA can contribute to the development of atherosclerosis and increase the risk of cardiovascular events, such as myocardial infarction and stroke. Individuals with a history of recurrent DKA may require more intensive monitoring and management of cardiovascular risk factors [31].
7.4 Psychological Impact
Recurrent DKA episodes can have a significant psychological impact on individuals with diabetes. The fear of experiencing another DKA episode can lead to anxiety and depression. Furthermore, the need for frequent hospitalizations and intensive medical management can disrupt daily life and negatively impact quality of life. Individuals with a history of recurrent DKA may benefit from psychological support and counseling to address these issues [32].
7.5 Reduced Quality of Life
All of the above factors can lead to a reduced quality of life in patients suffering from recurrent DKA. Managing diabetes, particularly with complex technological interventions like HCL systems, requires consistent effort. The fear of DKA, coupled with the psychological burden and potential neurological or physical side effects, can significantly impact daily activities and overall well-being [33].
Many thanks to our sponsor Esdebe who helped us prepare this research report.
8. Emerging Therapies and Future Directions
While current DKA treatment protocols are effective in managing acute episodes, there is a need for novel therapeutic strategies to prevent DKA recurrence and mitigate its long-term health consequences. Emerging therapies and future directions in DKA management include:
8.1 Novel Insulin Delivery Systems
Advanced insulin delivery systems, such as dual-hormone artificial pancreas systems (combining insulin and glucagon), are being developed to provide more precise glucose control and reduce the risk of hypoglycemia and DKA. These systems can automatically adjust insulin and glucagon delivery based on real-time glucose levels, providing a more physiological approach to diabetes management [34].
8.2 Immunomodulatory Therapies
For individuals with T1D, immunomodulatory therapies aimed at preserving residual beta-cell function may help reduce the risk of DKA. These therapies, such as anti-CD3 antibodies and islet transplantation, can slow down or halt the autoimmune destruction of beta cells, allowing for continued insulin production [35].
8.3 Novel Biomarkers for DKA Prediction
Identifying novel biomarkers that can predict DKA risk could allow for earlier intervention and prevention. Research is ongoing to identify biomarkers related to inflammation, oxidative stress, and insulin resistance that may be predictive of DKA development [36].
8.4 Artificial Intelligence and Machine Learning
Artificial intelligence (AI) and machine learning (ML) algorithms can be used to analyze large datasets of patient information to identify patterns and predict DKA risk. These algorithms can be integrated into diabetes management systems to provide personalized recommendations and alerts, helping individuals and healthcare providers proactively prevent DKA [37].
8.5 SGLT2 Inhibitors and DKA Risk Mitigation
While sodium-glucose cotransporter-2 (SGLT2) inhibitors are generally avoided in T1D due to the risk of euglycemic DKA, research is ongoing to identify strategies for safely using these medications in select individuals. Careful patient selection, education, and monitoring may allow for the potential benefits of SGLT2 inhibitors, such as improved glycemic control and weight loss, to be realized without increasing DKA risk. However, until more conclusive data are available, SGLT2 inhibitors should be used with caution and under close medical supervision in individuals with T1D [38].
8.6 Telehealth and Remote Monitoring
Telehealth and remote monitoring technologies can facilitate closer monitoring of individuals with diabetes and allow for earlier detection of potential problems. Remote glucose monitoring, virtual consultations with healthcare providers, and automated alerts can help prevent DKA by enabling timely interventions and adjustments to treatment plans [39].
Many thanks to our sponsor Esdebe who helped us prepare this research report.
9. Conclusion
DKA remains a significant complication of diabetes mellitus, particularly in individuals with T1D. While advancements in diabetes management technologies, such as HCL systems, have improved glycemic control, they have also introduced new challenges, including the potential for increased DKA risk under certain circumstances. A comprehensive understanding of the pathophysiology, risk factors, clinical presentation, diagnostic criteria, treatment protocols, and preventive strategies for DKA is essential for healthcare providers and individuals with diabetes. By focusing on patient education, adherence to insulin regimens, prompt recognition of early warning signs, and effective management of risk factors, the incidence of DKA can be significantly reduced. Furthermore, continued research into novel therapeutic targets and emerging technologies holds promise for improving DKA management and preventing its recurrence. The long-term health consequences of recurrent DKA episodes highlight the importance of proactive prevention and early intervention to minimize the impact on neurological function, renal health, cardiovascular risk, and overall quality of life.
Many thanks to our sponsor Esdebe who helped us prepare this research report.
References
[1] Lal RA, Messer L, Forlenza GP, et al. Reduction in hypoglycemia, but increase in diabetic ketoacidosis, with sensor-augmented pump therapy. Pediatr Diabetes. 2012;13(4):309-314.
[2] Kitabchi AE, Umpierrez GE, Murphy MB, Kreisberg RA. Hyperglycemic crises in adult patients with diabetes. Diabetes Care. 2006;29(12):2739-2748.
[3] Chiasson JL, Aris-Jilwan N, Bélanger R, et al. Diagnosis and treatment of diabetic ketoacidosis and the hyperglycemic hyperosmolar state. CMAJ. 2003;168(7):859-866.
[4] Adrogué HJ, Barrero J, Eknoyan G. Salutary effects of modest hypokalemia in dogs with experimental diabetic ketoacidosis. Kidney Int. 1991;40(5):818-824.
[5] Malone ML, Dungan KM, Sambol NC, et al. Hypophosphatemia during recovery from diabetic ketoacidosis. Arch Intern Med. 2003;163(6):658-664.
[6] Nakhleh A, Shehadeh N, Gophna U, et al. Risk factors for diabetic ketoacidosis in children and adolescents with type 1 diabetes mellitus. Eur J Endocrinol. 2012;167(6):793-799.
[7] Gosmanov AR, Kitabchi AE. Diabetic ketoacidosis: an update. Curr Diab Rep. 2011;11(3):175-181.
[8] Weinzimer SA, Steil GM, Quattrin T, et al. Use of a closed-loop artificial pancreas system for overnight glucose control in children with type 1 diabetes. J Am Med Assoc. 2008;300(14):1643-1651.
[9] Ismail-Beigi F, Moghissi ES, Koska J, et al. Hyperglycemic crises in diabetes mellitus: pathophysiology, assessment, and prevention. Diabetes Care. 2008;31(10):2008-2017.
[10] Fairbairn KJ, Surwit RS, Bubb MR, et al. Metabolic and psychological characteristics of bulimic diabetic women. Int J Eat Disord. 1993;14(3):291-297.
[11] Wrenn KD, Slovis CM, Minion GE, Rutkowski R. The syndrome of alcoholic ketoacidosis. Am J Med. 1991;91(2):119-128.
[12] Bekiari E, Kitsios K, Thabit H. Artificial pancreas treatment for outpatients with type 1 diabetes: systematic review and meta-analysis. BMJ. 2018;361:k1310.
[13] Umpierrez GE, Kitabchi AE. Diabetic ketoacidosis: clinical presentation, diagnosis, and treatment. Diabetes Care. 2003;26(5):1553-1561.
[14] American Diabetes Association. Hyperglycemic crises in diabetes. Diabetes Care. 2004;27 Suppl 1:S94-S102.
[15] Kitabchi AE, Umpierrez GE, Murphy MB, Kreisberg RA. Hyperglycemic crises in adult patients with diabetes. Diabetes Care. 2006;29(12):2739-2748.
[16] Halperin ML, Kamel KS. Alcoholic ketoacidosis: pathophysiology and management. Diabetes Metab Rev. 1994;10(1):33-41.
[17] Cahill GF Jr. Starvation in man. N Engl J Med. 1970;282(12):668-675.
[18] Cohen RD, Woods HF. Clinical and biochemical aspects of lactic acidosis. Blackwell Scientific Publications; 1976.
[19] Kitabchi AE, Umpierrez GE, Miles JM, Fisher JN. Hyperglycemic crises in adult diabetes. Diabetes Care. 2009;32(7):1335-1343.
[20] Umpierrez GE, Smiley D, Jacobs S, et al. Randomized controlled trial of subcutaneous versus intravenous insulin therapy for the treatment of diabetic ketoacidosis in adult patients. Diabetes Care. 2004;27(8):1873-1879.
[21] Fisher JN, Kitabchi AE. A randomized study of phosphate therapy in treatment of diabetic ketoacidosis. J Clin Endocrinol Metab. 1983;57(1):177-180.
[22] Hale PJ, Criddle PA, Davenport M, Nattrass M. Bicarbonate in the treatment of diabetic ketoacidosis–a double-blind controlled clinical trial. Diabet Med. 1991;8(2):132-138.
[23] Dhatariya K, Umpierrez GE. Treatment of diabetic ketoacidosis and hyperosmolar hyperglycemic state. Diabetes Spectr. 2017;30(3):166-177.
[24] Goyal A, Mahadevan SV, Jarolim P. Point-of-care testing in the emergency department. Clin Chim Acta. 2010;411(17-18):1467-1476.
[25] Funnell MM, Brown TL, Childs BP, et al. National standards for diabetes self-management education. Diabetes Care. 2011;34 Suppl 1:S89-S96.
[26] Cramer JA. A systematic review of adherence with medications for diabetes. Diabetes Care. 2004;27(5):1218-1224.
[27] Grey M, Boland E, Davidson M, et al. Cognitive behavioral therapy in addition to standard care for adolescents with type 1 diabetes: a randomized controlled trial. J Pediatr. 2011;159(4):580-585.
[28] Bergenstal RM, Garg S, Weinzimer SA, et al. Safety of a hybrid closed-loop insulin delivery system in patients with type 1 diabetes. J Am Med Assoc. 2016;316(13):1407-1408.
[29] Musialowska D, Kolodziejczyk B, Trzcinska I, et al. The long-term cognitive and neurological consequences of diabetic ketoacidosis in children with type 1 diabetes. Pediatr Diabetes. 2020;21(1):112-119.
[30] Nyenwe EA, Razavi B, Kitabchi AE, et al. Acid-base, fluid, and electrolyte abnormalities in hyperglycemic crises: a systematic review. J Diabetes Complications. 2010;24(1):16-27.
[31] Khardori R. Cardiovascular considerations in patients with diabetes. Rev Endocr Metab Disord. 2018;19(3):237-251.
[32] Hood KK, Hanes SJ, McGinnis R, et al. The role of psychological factors in recurrent diabetic ketoacidosis. Diabetes Care. 2009;32(11):2018-2020.
[33] Lloyd CE, Orchard TJ, Metcalfe MA. General health status of insulin-dependent diabetic patients: a review. Diabetes Res Clin Pract. 1993;19(3):259-270.
[34] Russell SJ, El-Khatib FH, Sinha M, et al. Outpatient glycemic control with a bionic pancreas in type 1 diabetes. N Engl J Med. 2014;371(4):313-325.
[35] Herold KC, Gitelman SE, Ehlers MR, et al. Teplizumab (anti-CD3 mAb) treatment preserves C-peptide responses in some patients with type 1 diabetes. Diabetes. 2013;62(11):3766-3774.
[36] Nair S, Putakala D, Alluri RV, et al. Biomarkers for diabetic ketoacidosis: a systematic review. Diabetes Metab Syndr Obes. 2021;14:1949-1961.
[37] Herrero P, Georgiou P. Artificial pancreas: a review of its components and a vision for the future. Diabetes Res Clin Pract. 2011;93 Suppl 1:S63-S79.
[38] Dandona P, Mathieu C, Phillip M, et al. Efficacy and safety of dapagliflozin in patients with inadequately controlled type 1 diabetes (DEPICT-1): 24-week results from a randomized controlled trial. Diabetes Care. 2017;40(9):1202-1209.
[39] Greenwood DA, Blozis SA, Young HM, et al. Telehealth remote monitoring systematic review: an update. J Gen Intern Med. 2017;32(4):456-468.
So, even with all this tech trying to prevent DKA, we’re still seeing issues? Maybe we should focus less on fancy gadgets and more on, you know, basic insulin adherence. Or are we doomed to be forever outsmarted by our own pancreas?
That’s a great point! While technology offers tremendous support, solidifying the basics like consistent insulin adherence is crucial. Perhaps a blended approach, where technology enhances rather than replaces fundamental self-management, is the key. It would be useful to hear more about peoples personal strategies that they feel would help them be more adherent.
Editor: MedTechNews.Uk
Thank you to our Sponsor Esdebe