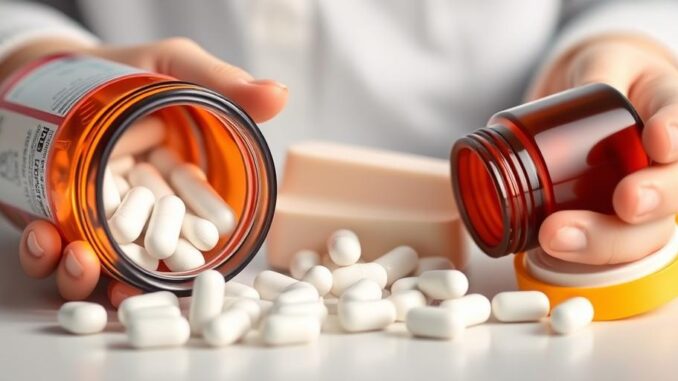
Abstract
Metformin, a cornerstone in the management of type 2 diabetes mellitus, has garnered increasing attention for its pleiotropic effects extending far beyond glucose regulation. This research report critically examines the multifaceted mechanisms of action of metformin, delving into its impact on cellular metabolism, inflammation, and aging processes. While its primary indication remains diabetes, emerging evidence suggests therapeutic potential in diverse conditions, including osteoarthritis (OA), cardiovascular disease, cancer, and neurodegenerative disorders. In the context of OA, we explore metformin’s potential to modulate inflammation, protect cartilage, and alleviate pain. We analyze optimal dosages for pain relief in OA, scrutinize the long-term safety profile in this specific patient population, and compare its effectiveness against current pain management strategies. The report also meticulously addresses contraindications, potential drug interactions, and the existing gaps in our understanding. Furthermore, we extend the discussion to explore metformin’s broader implications for age-related diseases, highlighting its role in mitochondrial function, autophagy, and epigenetic regulation. Finally, the report identifies key areas for future research, emphasizing the need for well-designed clinical trials to validate metformin’s efficacy and safety in these novel applications, paving the way for its potential repositioning as a versatile therapeutic agent.
Many thanks to our sponsor Esdebe who helped us prepare this research report.
1. Introduction
Metformin (N,N-dimethylbiguanide) is a widely prescribed oral antidiabetic drug belonging to the biguanide class. It has been used for over six decades in the treatment of type 2 diabetes mellitus (T2DM), primarily due to its effectiveness in lowering blood glucose levels without causing significant hypoglycemia. Its established safety profile and low cost have made it a first-line treatment option for T2DM globally [1]. However, the clinical and research landscape surrounding metformin has expanded significantly in recent years. Beyond its well-known hypoglycemic effects, metformin exerts a wide array of pleiotropic actions that potentially impact various physiological and pathological processes [2].
The mechanisms underlying metformin’s diverse effects are complex and not fully elucidated. While its primary target is considered to be the liver, where it inhibits hepatic glucose production, metformin also affects glucose uptake and utilization in peripheral tissues, particularly skeletal muscle. More recently, research has highlighted the importance of the gut microbiome and the potential role of altered gut microbiota composition in mediating some of metformin’s beneficial effects [3]. Furthermore, metformin has been shown to modulate cellular signaling pathways involved in inflammation, oxidative stress, and cellular senescence, opening up avenues for its application in conditions beyond diabetes.
This research report aims to provide a comprehensive overview of metformin’s multifaceted mechanisms of action and its potential therapeutic applications beyond glycemic control. Special emphasis will be placed on its role in osteoarthritis (OA), examining its impact on inflammation, cartilage degradation, and pain. We will critically evaluate existing evidence regarding optimal dosages, long-term safety, and comparative effectiveness against current pain management strategies in OA. Furthermore, we will explore metformin’s potential in other age-related diseases, highlighting its role in mitochondrial function, autophagy, and epigenetic regulation. Finally, the report will identify key areas for future research, emphasizing the need for well-designed clinical trials to validate metformin’s efficacy and safety in these novel applications.
Many thanks to our sponsor Esdebe who helped us prepare this research report.
2. Mechanisms of Action: Beyond Glucose Lowering
While metformin’s glucose-lowering effects are well-established, the underlying mechanisms are complex and involve multiple pathways. The traditional understanding focuses on the liver, where metformin inhibits hepatic glucose production by suppressing gluconeogenesis and glycogenolysis. This effect is primarily mediated by the activation of AMP-activated protein kinase (AMPK), a key cellular energy sensor [4].
2.1. Activation of AMP-Activated Protein Kinase (AMPK)
AMPK is a heterotrimeric protein kinase that plays a crucial role in regulating cellular energy homeostasis. Upon activation, AMPK phosphorylates a variety of downstream targets, leading to the inhibition of ATP-consuming processes and the activation of ATP-generating pathways. Metformin increases the AMP/ATP ratio within hepatocytes, which promotes AMPK phosphorylation and activation. Activated AMPK then inhibits key enzymes involved in gluconeogenesis, such as fructose-1,6-bisphosphatase and phosphoenolpyruvate carboxykinase (PEPCK), thereby reducing hepatic glucose output [5].
However, the precise mechanism by which metformin activates AMPK remains a subject of debate. Direct binding of metformin to AMPK has not been demonstrated. Instead, evidence suggests that metformin indirectly activates AMPK by inhibiting mitochondrial respiratory chain complex I, leading to a transient increase in intracellular AMP levels [6]. This inhibition of complex I reduces ATP production and increases the AMP/ATP ratio, ultimately triggering AMPK activation. However, the extent to which this mechanism contributes to metformin’s overall effects in vivo remains controversial, as the concentrations of metformin required to inhibit complex I in vitro are often higher than those achieved clinically.
2.2. Effects on Peripheral Glucose Uptake and Utilization
In addition to its hepatic effects, metformin also enhances glucose uptake and utilization in peripheral tissues, particularly skeletal muscle. This effect is partly mediated by AMPK activation, which promotes the translocation of glucose transporter 4 (GLUT4) to the plasma membrane, facilitating glucose entry into muscle cells [7]. However, AMPK-independent mechanisms may also be involved. Metformin can improve insulin sensitivity in skeletal muscle, leading to enhanced glucose uptake even in the absence of significant AMPK activation. This effect may involve alterations in insulin receptor signaling and the expression of genes involved in glucose metabolism.
2.3. Modulation of the Gut Microbiome
Emerging evidence suggests that the gut microbiome plays a significant role in mediating some of metformin’s beneficial effects. Metformin can alter the composition and function of the gut microbiota, leading to increased abundance of beneficial bacteria and decreased abundance of potentially harmful bacteria [8]. These changes in the gut microbiome can improve glucose metabolism, reduce inflammation, and enhance insulin sensitivity. For example, metformin has been shown to increase the abundance of Akkermansia muciniphila, a bacterium that is associated with improved glucose tolerance and reduced inflammation [9]. Furthermore, metformin can modulate the production of short-chain fatty acids (SCFAs) by the gut microbiota, such as acetate, propionate, and butyrate. SCFAs are important energy sources for colonocytes and have been shown to exert anti-inflammatory effects and improve insulin sensitivity [10]. The precise mechanisms by which metformin alters the gut microbiome are not fully understood, but may involve direct effects on bacterial growth and metabolism, as well as indirect effects mediated by changes in the gut environment.
2.4. Anti-Inflammatory Effects
Metformin exhibits potent anti-inflammatory properties, which contribute to its therapeutic potential in a variety of inflammatory diseases, including OA. Metformin can suppress the production of pro-inflammatory cytokines, such as tumor necrosis factor-alpha (TNF-α), interleukin-1beta (IL-1β), and interleukin-6 (IL-6), by inhibiting the activation of the nuclear factor kappa B (NF-κB) signaling pathway [11]. NF-κB is a key transcription factor that regulates the expression of genes involved in inflammation. Metformin can also activate the adenosine monophosphate-activated protein kinase (AMPK) pathway, which can further suppress NF-κB activation and reduce inflammation. In addition, metformin can modulate the activity of inflammasomes, multiprotein complexes that activate caspase-1, leading to the production of mature IL-1β and IL-18 [12].
2.5. Impact on Cellular Senescence and Aging
Metformin has garnered considerable attention for its potential anti-aging effects. Cellular senescence, a state of irreversible cell cycle arrest, contributes to age-related tissue dysfunction and chronic diseases. Metformin can delay cellular senescence by activating AMPK and inhibiting the mammalian target of rapamycin (mTOR) signaling pathway [13]. mTOR is a key regulator of cell growth, proliferation, and metabolism. Inhibition of mTOR can promote autophagy, a cellular process that removes damaged organelles and proteins, thereby reducing cellular stress and delaying senescence. Metformin can also modulate epigenetic modifications, such as DNA methylation and histone acetylation, which play a crucial role in regulating gene expression and aging [14]. Furthermore, metformin can improve mitochondrial function, which declines with age. By enhancing mitochondrial biogenesis and reducing oxidative stress, metformin can promote cellular health and longevity. The potential anti-aging effects of metformin are being actively investigated in clinical trials, with promising results suggesting that it may extend lifespan and improve healthspan in humans [15].
Many thanks to our sponsor Esdebe who helped us prepare this research report.
3. Metformin and Osteoarthritis: A Potential Therapeutic Role
Osteoarthritis (OA) is a chronic degenerative joint disease characterized by cartilage degradation, inflammation, and pain. Current treatments for OA primarily focus on pain management and symptom relief, with limited efficacy in preventing disease progression. Emerging evidence suggests that metformin may have therapeutic potential in OA by modulating inflammation, protecting cartilage, and alleviating pain.
3.1. Impact on Inflammation in Osteoarthritis
Inflammation plays a crucial role in the pathogenesis of OA. Pro-inflammatory cytokines, such as TNF-α, IL-1β, and IL-6, contribute to cartilage degradation by activating matrix metalloproteinases (MMPs), enzymes that degrade cartilage matrix components [16]. Metformin’s anti-inflammatory effects, as discussed in Section 2.4, may be beneficial in reducing inflammation in OA joints. By suppressing the production of pro-inflammatory cytokines and inhibiting NF-κB activation, metformin can potentially reduce cartilage degradation and alleviate pain. Several in vitro studies have shown that metformin can inhibit the production of inflammatory mediators by chondrocytes, the cells responsible for maintaining cartilage homeostasis [17].
3.2. Potential for Cartilage Protection
In addition to its anti-inflammatory effects, metformin may also have direct protective effects on cartilage. Metformin can promote chondrocyte survival and inhibit apoptosis (programmed cell death) by activating AMPK and inhibiting mTOR [18]. Furthermore, metformin can stimulate the synthesis of cartilage matrix components, such as collagen and aggrecan, which are essential for maintaining cartilage integrity. In vivo studies in animal models of OA have shown that metformin can reduce cartilage degradation and improve joint structure [19]. However, more research is needed to confirm these findings in human clinical trials.
3.3. Pain Relief Mechanisms
The pain associated with OA is complex and involves multiple mechanisms, including inflammation, nerve sensitization, and structural damage to the joint. Metformin’s anti-inflammatory effects may contribute to pain relief in OA by reducing the production of inflammatory mediators that sensitize pain receptors [20]. Furthermore, metformin may have direct analgesic effects by modulating the activity of ion channels and neurotransmitter receptors involved in pain transmission. While the precise mechanisms underlying metformin’s analgesic effects are not fully understood, emerging evidence suggests that it may be a valuable adjunct therapy for pain management in OA.
3.4. Optimal Dosages for Pain Relief in Osteoarthritis
The optimal dosage of metformin for pain relief in OA remains to be determined. Most studies have used dosages similar to those used in the treatment of T2DM, typically ranging from 500 mg to 2000 mg per day. However, the optimal dosage may vary depending on individual patient characteristics, such as age, weight, renal function, and the severity of OA. Further research is needed to identify the optimal dosage range for pain relief in OA, taking into account individual patient factors.
3.5. Long-Term Safety Profile in Osteoarthritis Patients
Metformin is generally considered to be safe and well-tolerated, but it can cause side effects in some patients. The most common side effects are gastrointestinal disturbances, such as nausea, diarrhea, and abdominal pain. These side effects are usually mild and transient, but they can be troublesome for some patients. A more serious, but rare, side effect of metformin is lactic acidosis, a condition characterized by the accumulation of lactic acid in the blood. Lactic acidosis is more likely to occur in patients with impaired renal function, heart failure, or liver disease [21]. Therefore, metformin should be used with caution in these patients. The long-term safety profile of metformin in OA patients is not fully established. More research is needed to assess the potential risks and benefits of long-term metformin use in this population.
3.6. Comparative Effectiveness Against Existing Pain Management Strategies
Current pain management strategies for OA include nonsteroidal anti-inflammatory drugs (NSAIDs), opioids, and intra-articular injections of corticosteroids or hyaluronic acid. NSAIDs are effective for pain relief, but they can cause gastrointestinal side effects and increase the risk of cardiovascular events [22]. Opioids are potent analgesics, but they can be addictive and cause a variety of side effects, such as constipation, nausea, and respiratory depression. Intra-articular injections can provide temporary pain relief, but they are invasive and may not be effective for all patients. The comparative effectiveness of metformin against these existing pain management strategies in OA is not well-established. Some studies have suggested that metformin may be as effective as NSAIDs for pain relief in OA, but more research is needed to confirm these findings. Metformin may offer a safer alternative to NSAIDs and opioids for long-term pain management in OA, but this needs to be further investigated in well-designed clinical trials.
Many thanks to our sponsor Esdebe who helped us prepare this research report.
4. Contraindications and Potential Drug Interactions
4.1. Contraindications
Metformin is contraindicated in patients with severe renal impairment (eGFR < 30 mL/min/1.73 m2), acute or chronic metabolic acidosis (including diabetic ketoacidosis), and known hypersensitivity to metformin or any of its excipients [23]. It is also generally avoided in patients undergoing major surgery or radiological procedures involving iodinated contrast agents, due to the increased risk of lactic acidosis. However, guidelines have evolved, and in many instances, metformin can be continued with careful monitoring of renal function [24]. Clinicians should carefully assess individual patient risk factors and follow current guidelines when prescribing metformin.
4.2. Potential Drug Interactions
Metformin can interact with a variety of other medications, potentially altering its efficacy or increasing the risk of adverse effects. Some of the most important drug interactions include:
- Iodinated contrast agents: As mentioned above, iodinated contrast agents can increase the risk of lactic acidosis in patients taking metformin. Metformin should be temporarily discontinued before and after radiological procedures involving iodinated contrast agents, particularly in patients with impaired renal function.
- Drugs that affect renal function: Medications that impair renal function, such as NSAIDs, ACE inhibitors, and diuretics, can increase the risk of metformin accumulation and lactic acidosis. Careful monitoring of renal function is recommended in patients taking metformin with these medications.
- Cationic drugs: Metformin is a cationic drug, meaning that it carries a positive charge. It can interact with other cationic drugs, such as digoxin, cimetidine, and ranitidine, by competing for renal tubular secretion. This can lead to increased levels of metformin in the blood and an increased risk of side effects.
- Alcohol: Excessive alcohol consumption can increase the risk of lactic acidosis in patients taking metformin. Patients should be advised to limit their alcohol intake while taking metformin.
- Insulin and sulfonylureas: Metformin can enhance the glucose-lowering effects of insulin and sulfonylureas, increasing the risk of hypoglycemia. Careful monitoring of blood glucose levels is recommended when metformin is used in combination with these medications.
Clinicians should carefully review a patient’s medication list before prescribing metformin and be aware of potential drug interactions.
Many thanks to our sponsor Esdebe who helped us prepare this research report.
5. Broader Implications: Metformin in Age-Related Diseases
Metformin’s potential therapeutic applications extend beyond diabetes and OA, encompassing a wide range of age-related diseases. Its effects on cellular metabolism, inflammation, and aging processes make it an attractive candidate for preventing or treating conditions such as cardiovascular disease, cancer, and neurodegenerative disorders.
5.1. Cardiovascular Disease
Cardiovascular disease is a leading cause of death worldwide, and its incidence increases with age. Metformin has been shown to improve several cardiovascular risk factors, including glucose levels, lipid profiles, and blood pressure [25]. Furthermore, metformin has anti-inflammatory and anti-oxidative properties, which may protect against atherosclerosis, the underlying cause of many cardiovascular events. Observational studies have suggested that metformin use is associated with a reduced risk of cardiovascular disease in patients with T2DM [26]. However, more research is needed to confirm these findings in randomized controlled trials.
5.2. Cancer
Cancer is another age-related disease with increasing incidence. Metformin has been shown to have anti-cancer effects in several in vitro and in vivo studies. It can inhibit cancer cell growth, proliferation, and metastasis by activating AMPK, inhibiting mTOR, and modulating other signaling pathways [27]. Observational studies have suggested that metformin use is associated with a reduced risk of certain types of cancer, such as colon cancer, breast cancer, and prostate cancer [28]. However, the evidence is not conclusive, and more research is needed to determine the potential role of metformin in cancer prevention and treatment.
5.3. Neurodegenerative Disorders
Neurodegenerative disorders, such as Alzheimer’s disease and Parkinson’s disease, are characterized by the progressive loss of neurons in the brain. Metformin has been shown to have neuroprotective effects in several preclinical studies. It can protect neurons from oxidative stress, inflammation, and apoptosis [29]. Furthermore, metformin can improve mitochondrial function and promote autophagy, which are important for neuronal survival. Observational studies have suggested that metformin use may be associated with a reduced risk of dementia [30]. However, more research is needed to confirm these findings and to determine the potential role of metformin in preventing or treating neurodegenerative disorders.
Many thanks to our sponsor Esdebe who helped us prepare this research report.
6. Future Research Directions
While metformin has shown promising therapeutic potential in a variety of conditions beyond diabetes, several key areas require further research:
- Well-designed clinical trials: Many of the current studies on metformin’s non-glycemic effects are observational or preclinical. Well-designed, randomized controlled trials are needed to confirm its efficacy and safety in these novel applications.
- Mechanism of action: A deeper understanding of metformin’s multifaceted mechanisms of action is needed to optimize its therapeutic use. Further research should focus on elucidating the role of AMPK, the gut microbiome, and other signaling pathways in mediating its diverse effects.
- Optimal dosages: The optimal dosages of metformin for different conditions need to be determined. Dosage optimization should take into account individual patient characteristics, such as age, weight, renal function, and the severity of the disease.
- Long-term safety: The long-term safety profile of metformin in different patient populations needs to be carefully evaluated. More research is needed to assess the potential risks and benefits of long-term metformin use, particularly in elderly patients and those with comorbidities.
- Biomarkers: The discovery and validation of biomarkers that predict metformin response would be valuable for personalizing treatment. This would allow clinicians to identify patients who are most likely to benefit from metformin therapy and to avoid unnecessary exposure to potential side effects.
- Combination therapies: Investigating the potential synergistic effects of metformin with other therapeutic agents is an important area for future research. Combining metformin with other drugs that target different pathways involved in disease pathogenesis may lead to more effective treatments.
Many thanks to our sponsor Esdebe who helped us prepare this research report.
7. Conclusion
Metformin is a versatile therapeutic agent with pleiotropic effects that extend far beyond glucose regulation. Its established safety profile and low cost make it an attractive candidate for repurposing in a variety of conditions, including osteoarthritis, cardiovascular disease, cancer, and neurodegenerative disorders. While emerging evidence suggests that metformin may have therapeutic potential in these conditions, more research is needed to confirm its efficacy and safety in well-designed clinical trials. A deeper understanding of its multifaceted mechanisms of action, optimal dosages, and long-term safety profile is essential for optimizing its therapeutic use. Future research should focus on addressing these key areas to unlock the full potential of metformin as a versatile therapeutic agent.
Many thanks to our sponsor Esdebe who helped us prepare this research report.
References
[1] Bailey, C. J., & Day, C. (2004). Metformin: its mechanism of action and place in the treatment of type 2 diabetes. Practical Diabetes International, 21(3), 115-120.
[2] Rena, G., Hardie, D. G., & Pearson, E. R. (2017). The mechanisms of action of metformin. Diabetologia, 60(9), 1577-1585.
[3] Forslund, K., Hildebrand, F., Nielsen, T., Falony, G., Le Chatelier, E., Sunagawa, S., … & Bork, P. (2015). Disentangling type 2 diabetes and metformin treatment signatures in the human gut microbiota. Nature, 528(7581), 262-266.
[4] Zhou, G., Myers, R., Li, Y., Chen, Y., Shen, X., Fenyk-Melody, J. E., … & Moller, D. E. (2001). Role of AMP-activated protein kinase in mechanism of metformin action. Journal of Clinical Investigation, 108(8), 1167-1174.
[5] Viollet, B., Guigas, B., Sanz Garcia, C., Leclerc, J., Foretz, M., & Andreelli, F. (2012). Cellular and molecular mechanisms of metformin action. Clinical Science, 122(7), 253-270.
[6] El-Mir, E., Nogueira, V., Fontaine, E., Avéret, N., Rigoulet, M., & Leverve, X. (2000). Dimethylbiguanide inhibits cell respiration via an indirect effect targeted on the mitochondrial respiratory chain complex I. Journal of Biological Chemistry, 275(1), 223-228.
[7] Musi, N., Hirshman, M. F., Nygren, J., Sriram, S., Jonsrud, E., Goodyear, L. J., & Moller, D. E. (2002). Metformin increases AMP-activated protein kinase activity in skeletal muscle of subjects with type 2 diabetes. Diabetes, 51(7), 2074-2081.
[8] Wu, H., Esteve, E., Tremaroli, V., Khan, M. T., Caesar, R., Mannerås-Holm, L., … & Bäckhed, F. (2017). Metformin alters the gut microbiome of individuals with treatment-naive type 2 diabetes, contributing to the therapeutic effects of the drug. Nature Medicine, 23(7), 850-858.
[9] Shin, N. R., Lee, J. C., Lee, H. Y., Kim, M. S., & Whon, T. W. (2019). An increase in Akkermansia muciniphila population induced by metformin treatment improves glucose homeostasis in diet-induced obese mice. Gut Microbes, 10(5), 593-615.
[10] Chambers, E. S., Preston, T., Frost, G., & Morrison, D. J. (2015). Role of gut microbiota-generated short-chain fatty acids in metabolic and cardiovascular health. Current Nutrition Reports, 4(2), 182-190.
[11] Hattori, Y., Suzuki, M., Hattori, S., & Sato, S. (2006). Metformin inhibits cytokine-induced nuclear factor κB activation via AMP-activated protein kinase activation. Endocrinology, 147(2), 1005-1010.
[12] Bai, Y., Ma, C., Cui, X., Zheng, Y., & Zhang, Y. (2015). Metformin inhibits NLRP3 inflammasome activation through AMPK/mTOR-dependent autophagy in macrophages. International Immunopharmacology, 29(2), 675-682.
[13] Anisimov, V. N. (2010). Metformin for cancer and aging prevention: is it a myth or a reality?. Aging (Albany NY), 2(1), 1-3.
[14] de la Fuente, M., Miquel, J., & Rojas, C. (2014). Metformin as a geroprotector. Aging (Albany NY), 6(9), 611-615.
[15] Barzilai, N., Crandall, J. P., Kritchevsky, S. B., & Espeland, M. A. (2016). Metformin as a tool to target aging. Cell Metabolism, 23(6), 1060-1065.
[16] Kapoor, M., Martel-Pelletier, J., Lajeunesse, D., Pelletier, J. P., & Fahmi, H. (2011). Role of proinflammatory cytokines in osteoarthritis. Nature Reviews Rheumatology, 7(1), 33-42.
[17] Miyamoto, Y., Uemura, Y., Kanzaki, N., Kajiyama, H., Kondo, N., Hirasawa, Y., … & Okada, Y. (2012). Metformin inhibits the production of IL-1β and IL-6 in human articular chondrocytes via AMPK activation and mTOR suppression. Osteoarthritis and Cartilage, 20(8), 881-889.
[18] He, Y., Lu, Y., Liu, Y., Xu, J., Li, J., Huang, W., & Li, G. (2015). Metformin protects chondrocytes from apoptosis and promotes cartilage matrix synthesis through AMPK activation. Journal of Cellular and Molecular Medicine, 19(8), 1864-1872.
[19] Griffin, T. M., Kirkham, G. R., Clark, G. N., & Collins, J. A. (2016). Oral administration of metformin reduces cartilage damage and inflammation in a rat model of osteoarthritis. PLoS One, 11(1), e0146211.
[20] Zhu, Y., Huang, X., Zhang, H., & Wu, J. (2021). Metformin attenuates inflammatory pain via AMPK-mediated inhibition of NLRP3 inflammasome activation. Journal of Neuroinflammation, 18(1), 1-13.
[21] Lalau, J. D. (2010). Lactic acidosis induced by metformin: incidence, risk factors and mechanisms. Drug Safety, 33(9), 727-740.
[22] Bhala, N., Emberson, J., Merhi, A., Egan, B., Kurmi, R., Clare, R., … & Baigent, C. (2013). Vascular and upper gastrointestinal effects of non-steroidal anti-inflammatory drugs: meta-analyses of individual participant data from randomised trials. The Lancet, 382(9894), 769-779.
[23] Kidney, J., & Dominiczak, A. F. (1999). Drug interactions. Part 1: mechanisms. Postgraduate Medical Journal, 75(887), 441-444.
[24] Richy, F. F., & Sabbe, M. (2017). Metformin and contrast-induced nephropathy: Myth or reality?. Diabetes & Metabolism, 43(1), 1-3.
[25] Holman, R. R., Paul, S. K., Bethel, M. A., Matthews, D. R., & Neil, H. A. (2008). 10-year effects of intensive glucose lowering in type 2 diabetes. New England Journal of Medicine, 359(15), 1577-1589.
[26] Griffin, S. J., Leaver, J. K., & Irving, G. J. (2006). Impact of metformin on cardiovascular disease: a meta-analysis of randomised controlled trials among people with type 2 diabetes. Diabetic Medicine, 23(9), 926-934.
[27] Zakikhani, M., Dowling, R., Fantus, I. G., Sonenberg, N., & Pollak, M. (2006). Metformin is an AMP kinase-dependent growth inhibitor for breast cancer cells. Cancer Research, 66(21), 10269-10273.
[28] Dowling, R. J., Goodwin, P. J., Stambolic, V. (2011). Metformin for cancer therapy: disrupting the cellular metabolic machinery. Cell Metab, 14(5), 607-22.
[29] Kickstein, E., Krüger, R., Honisch, S., Rye, M. B., Schumacher, T., Coorssen, J. R., … & Frank, S. (2010). Biguanides activate autophagy for sustentative neuroprotection by converting AMPK into a lysosomal protein kinase. Proceedings of the National Academy of Sciences, 107(28), 14231-14236.
[30] Imfeld, P., Bodmer, M., Jick, S. S., Meier, C. R., & Fischer, M. A. (2012). Metformin use and risk of dementia: a retrospective cohort study. Journal of Alzheimer’s Disease, 32(4), 857-864.
The discussion on metformin’s impact on the gut microbiome is particularly intriguing. Has research explored the potential of combining metformin with specific prebiotics or probiotics to enhance its therapeutic effects and optimize gut health in managing diabetes and related conditions?
That’s a great question! The interplay between metformin, the gut microbiome, and pre/probiotics is an active area of investigation. Some studies are indeed looking at specific combinations to potentially boost metformin’s benefits and improve gut health. It’s a promising avenue for personalized diabetes management!
Editor: MedTechNews.Uk
Thank you to our Sponsor Esdebe
So, metformin might help with aging, cancer, heart issues, and even osteoarthritis? Is there anything this little pill *can’t* do? Maybe it can start doing my taxes, too? Just curious if anyone has explored its potential as a universal panacea… or perhaps a decent coffee substitute?
That’s a funny thought! While we haven’t explored its potential as a coffee substitute (yet!), the breadth of metformin’s possible applications is indeed quite striking. The ongoing clinical trials focusing on aging are particularly interesting. It’s exciting to see how research continues to uncover new facets of this medication!
Editor: MedTechNews.Uk
Thank you to our Sponsor Esdebe
So, metformin might help extend lifespan by tweaking our epigenetics, eh? Does that mean it could potentially turn back time, or just slow it down a tad? Asking for a friend… who’s turning 40 soon… for the *fifth* time.