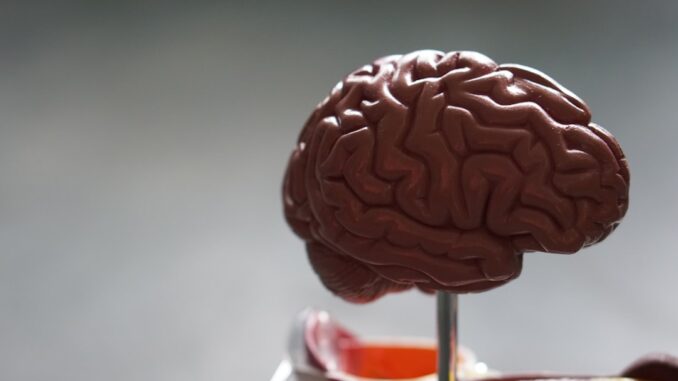
Abstract
Alzheimer’s disease (AD) is a devastating neurodegenerative disorder characterized by the accumulation of amyloid-beta plaques and neurofibrillary tangles composed of hyperphosphorylated tau protein. While the pathogenic roles of neurons in AD have been extensively studied, the contribution of glial cells, particularly oligodendrocytes, is gaining increasing attention. Oligodendrocytes, the myelinating cells of the central nervous system, play a critical role in neuronal health by providing axonal support, facilitating rapid signal transduction, and offering metabolic sustenance. Emerging evidence suggests that oligodendrocytes are not merely passive bystanders in AD pathology but actively participate in modulating tau-induced neurodegeneration. This report comprehensively reviews the potential mechanisms by which oligodendrocytes may exert protective effects against tauopathy, encompassing their myelination function, metabolic support, and anti-inflammatory properties. Furthermore, it explores promising therapeutic strategies aimed at enhancing oligodendrocyte function and promoting their survival in the context of AD, including the promotion of oligodendrocyte differentiation and function to protect neurons from tau-mediated damage. Finally, the report discusses the challenges and future directions in translating these preclinical findings into effective clinical interventions for AD.
Many thanks to our sponsor Esdebe who helped us prepare this research report.
1. Introduction
Alzheimer’s disease (AD) is a progressive neurodegenerative disorder characterized by cognitive decline and memory loss, ultimately leading to severe disability and death. The neuropathological hallmarks of AD include the accumulation of extracellular amyloid-beta (Aβ) plaques and intracellular neurofibrillary tangles (NFTs) composed of hyperphosphorylated tau protein. While the precise etiology of AD remains elusive, it is widely accepted that the interplay between Aβ and tau pathologies contributes to neuronal dysfunction and synaptic loss, ultimately driving cognitive impairment [1]. Much of the research has focused on the neurons and the role they play in the disease. However, it is becoming clear that the disease is multifactorial and that glial cells play an important role, directly and indirectly. Glial cells are the most abundant cell type in the brain, they include astrocytes, microglia, and oligodendrocytes. This report will focus on the role of oligodendrocytes, and the potential they hold as a key modulator in AD.
Historically, AD research has predominantly focused on neurons, particularly the role of Aβ and tau in neuronal dysfunction and death. However, accumulating evidence underscores the importance of glial cells, including astrocytes, microglia, and oligodendrocytes, in the pathogenesis of AD [2]. These glial cells, once considered mere support cells, are now recognized as active participants in the disease process, contributing to both neuroprotection and neurodegeneration. Oligodendrocytes, the myelinating cells of the central nervous system (CNS), play a crucial role in neuronal health by providing myelin sheaths that enable rapid saltatory conduction of action potentials, supporting axonal integrity, and providing metabolic support to neurons [3]. It is becoming clear that oligodendrocytes are not just passive targets of AD pathology, but rather that they can actively influence the progression of the disease.
Recently, there has been a growing interest in the potential role of oligodendrocytes in modulating tau pathology and neurodegeneration in AD. Several studies have shown that oligodendrocyte dysfunction and myelin abnormalities are present in AD brains, suggesting that these cells may be vulnerable to the disease process. However, emerging evidence also suggests that oligodendrocytes may possess protective mechanisms against tau-induced damage, potentially through their role in myelination, metabolic support, and anti-inflammatory effects [4]. This report aims to provide a comprehensive overview of the current understanding of oligodendrocytes in the context of AD, focusing on their potential protective mechanisms against tauopathy and exploring therapeutic strategies aimed at enhancing oligodendrocyte function and promoting their survival in the AD brain. Understanding the multifaceted roles of oligodendrocytes in AD is crucial for developing novel therapeutic interventions that target not only neurons but also the glial environment, ultimately leading to more effective treatments for this devastating disease.
Many thanks to our sponsor Esdebe who helped us prepare this research report.
2. The Role of Oligodendrocytes in Neuronal Health
Oligodendrocytes are specialized glial cells responsible for the formation and maintenance of myelin sheaths around axons in the CNS. Myelination is a critical process that ensures the rapid and efficient propagation of action potentials, enabling fast communication between neurons [5]. In addition to their role in myelination, oligodendrocytes also provide crucial metabolic support to neurons, supplying them with energy substrates such as lactate, which is essential for neuronal function [6]. Furthermore, oligodendrocytes secrete trophic factors that promote neuronal survival and growth, contributing to the overall health and integrity of neuronal circuits [7].
2.1 Myelination and Axonal Integrity
The myelin sheath, formed by the wrapping of oligodendrocyte processes around axons, acts as an insulator, preventing ion leakage and increasing the speed of action potential conduction. This process, known as saltatory conduction, allows action potentials to jump between nodes of Ranvier, specialized regions of the axon where ion channels are concentrated [8]. Myelination is crucial for maintaining axonal integrity and preventing axonal degeneration. Disruption of myelination, as observed in demyelinating diseases such as multiple sclerosis, leads to impaired neuronal communication, axonal damage, and ultimately, neurological dysfunction [9].
In the context of AD, myelin abnormalities and oligodendrocyte dysfunction have been reported in multiple brain regions, including the hippocampus, cortex, and white matter [10]. These myelin deficits may contribute to the cognitive decline observed in AD patients by impairing neuronal communication and increasing axonal vulnerability to damage. Furthermore, myelin breakdown products, such as myelin-associated glycoprotein (MAG), can trigger inflammatory responses and contribute to neurodegeneration [11].
2.2 Metabolic Support and Trophic Factors
Oligodendrocytes provide metabolic support to neurons by supplying them with energy substrates such as lactate, which is generated through glycolysis [12]. Neurons rely heavily on lactate as an energy source, particularly during periods of high activity. Oligodendrocytes transport lactate to neurons via monocarboxylate transporters (MCTs), ensuring that neurons have sufficient energy to maintain their function and survival [13]. The loss of this metabolic support can severely affect neuronal function, especially where it is reliant on it.
In addition to metabolic support, oligodendrocytes also secrete trophic factors that promote neuronal survival and growth. These trophic factors, such as brain-derived neurotrophic factor (BDNF) and nerve growth factor (NGF), play a critical role in maintaining neuronal health and preventing neuronal death [14]. BDNF, in particular, has been shown to enhance synaptic plasticity and promote neuronal survival in the context of AD [15]. Thus, oligodendrocyte-derived trophic factors may provide a protective mechanism against tau-induced neurodegeneration.
Many thanks to our sponsor Esdebe who helped us prepare this research report.
3. Oligodendrocytes and Tau Pathology in Alzheimer’s Disease
While the precise mechanisms underlying the pathogenesis of AD remain elusive, it is widely accepted that the accumulation of Aβ plaques and NFTs composed of hyperphosphorylated tau protein plays a central role in driving neuronal dysfunction and synaptic loss. Tau, a microtubule-associated protein, is normally involved in stabilizing microtubules and regulating axonal transport. However, in AD, tau becomes hyperphosphorylated, leading to its detachment from microtubules and aggregation into insoluble NFTs [16].
Recent evidence suggests that oligodendrocytes may be actively involved in modulating tau pathology and neurodegeneration in AD. Several studies have shown that oligodendrocyte dysfunction and myelin abnormalities are associated with increased tau phosphorylation and aggregation in neurons [17]. Furthermore, oligodendrocytes may contribute to the spread of tau pathology by taking up and releasing tau seeds, thereby promoting the propagation of tau aggregates to neighboring neurons [18].
3.1 Oligodendrocyte Dysfunction and Tau Hyperphosphorylation
Oligodendrocyte dysfunction, characterized by reduced myelin production and impaired metabolic support, may exacerbate tau hyperphosphorylation in neurons. Studies have shown that myelin deficits are associated with increased levels of phosphorylated tau in the hippocampus and cortex of AD patients [19]. Furthermore, oligodendrocyte dysfunction may impair the clearance of misfolded tau protein, leading to its accumulation and aggregation into NFTs. This creates a feedback loop, with hyperphosphorylated tau damaging the Oligodendrocytes, leading to more hyperphosphorylated tau.
The mechanisms by which oligodendrocyte dysfunction contributes to tau hyperphosphorylation are not fully understood. However, one possibility is that impaired metabolic support from oligodendrocytes may disrupt neuronal energy homeostasis, leading to increased stress and activation of kinases that phosphorylate tau [20]. Furthermore, oligodendrocyte dysfunction may impair the trafficking of tau protein within neurons, leading to its mislocalization and aggregation. This area is an active one for new research.
3.2 Oligodendrocytes and Tau Propagation
Emerging evidence suggests that oligodendrocytes may contribute to the spread of tau pathology by taking up and releasing tau seeds, thereby promoting the propagation of tau aggregates to neighboring neurons. Tau seeds, small aggregates of misfolded tau protein, can act as templates to induce the misfolding and aggregation of normal tau protein, leading to the formation of NFTs [21].
Oligodendrocytes can take up tau seeds via endocytosis and release them via exosomes, small vesicles that are secreted by cells [22]. These exosomes can then be taken up by neighboring neurons, delivering the tau seeds and initiating the formation of NFTs. This process may contribute to the spread of tau pathology from one brain region to another, ultimately driving the progression of AD. One important factor to note, is that some forms of tau are known to be more easily taken up by cells than others [23].
Many thanks to our sponsor Esdebe who helped us prepare this research report.
4. Potential Protective Mechanisms of Oligodendrocytes Against Tauopathy
Despite the evidence suggesting that oligodendrocytes may contribute to tau pathology in AD, there is also growing evidence that these cells may possess protective mechanisms against tau-induced damage. These protective mechanisms include their role in myelination, metabolic support, and anti-inflammatory effects.
4.1 Myelination and Axonal Protection
Myelination, the primary function of oligodendrocytes, provides crucial support and protection to axons, preventing axonal damage and degeneration. In the context of tauopathy, myelination may protect axons from the toxic effects of misfolded tau protein. Studies have shown that increased myelination is associated with reduced tau phosphorylation and aggregation in neurons [24].
The mechanisms by which myelination protects against tauopathy are not fully understood. However, one possibility is that myelination increases axonal stability and reduces the vulnerability of axons to tau-induced damage. Furthermore, myelination may promote the trafficking of tau protein within axons, preventing its mislocalization and aggregation. It is also possible that the sheer bulk of the myelin sheath provides a physical barrier against tau seeds entering the axon.
4.2 Metabolic Support and Neuronal Resilience
Oligodendrocytes provide metabolic support to neurons by supplying them with energy substrates such as lactate, which is essential for neuronal function and survival. In the context of tauopathy, metabolic support from oligodendrocytes may enhance neuronal resilience to the toxic effects of misfolded tau protein. Studies have shown that increased lactate production by oligodendrocytes is associated with reduced tau phosphorylation and aggregation in neurons [25].
The mechanisms by which metabolic support protects against tauopathy are not fully understood. However, one possibility is that increased energy availability enhances neuronal protein quality control mechanisms, allowing neurons to more effectively clear misfolded tau protein. Furthermore, metabolic support may reduce neuronal stress and prevent the activation of kinases that phosphorylate tau. Understanding the interplay between Oligodendrocytes and neurons is crucial for identifying new approaches to improve function.
4.3 Anti-Inflammatory Effects
Oligodendrocytes can exert anti-inflammatory effects in the brain by releasing factors that suppress microglial activation and reduce the production of pro-inflammatory cytokines. In the context of tauopathy, these anti-inflammatory effects may protect neurons from tau-induced damage by reducing neuroinflammation [26].
Chronic neuroinflammation is a prominent feature of AD and contributes to neuronal dysfunction and death. Microglia, the resident immune cells of the brain, become activated in response to Aβ plaques and NFTs, releasing pro-inflammatory cytokines that exacerbate neurodegeneration. Oligodendrocytes can suppress microglial activation by releasing factors such as transforming growth factor-β (TGF-β) and interleukin-10 (IL-10), which inhibit the production of pro-inflammatory cytokines [27]. This is an area that has seen some positive research, although more work is needed to fully understand the potential benefits and limitations.
Many thanks to our sponsor Esdebe who helped us prepare this research report.
5. Therapeutic Strategies Targeting Oligodendrocytes in Alzheimer’s Disease
Given the growing evidence that oligodendrocytes play a critical role in modulating tau pathology and neurodegeneration in AD, there is increasing interest in developing therapeutic strategies that target these cells to protect neurons from tau-mediated damage. These therapeutic strategies include promoting oligodendrocyte differentiation and function, enhancing oligodendrocyte survival, and modulating oligodendrocyte-mediated inflammation.
5.1 Promoting Oligodendrocyte Differentiation and Function
One promising therapeutic strategy is to promote oligodendrocyte differentiation and function, thereby increasing the number of myelinating oligodendrocytes in the brain and enhancing their capacity to provide metabolic support and trophic factors to neurons. This can be achieved through the use of small molecules that stimulate oligodendrocyte precursor cell (OPC) differentiation into mature oligodendrocytes [28].
Several small molecules have been identified that can promote OPC differentiation, including clemastine fumarate, a histamine receptor antagonist, and benztropine, an anticholinergic drug [29]. These compounds have shown promise in preclinical studies, increasing the number of myelinating oligodendrocytes and improving functional outcomes in animal models of demyelination. However, further research is needed to determine their efficacy and safety in AD patients.
5.2 Enhancing Oligodendrocyte Survival
Another therapeutic strategy is to enhance oligodendrocyte survival, preventing oligodendrocyte death and maintaining the integrity of the myelin sheath. This can be achieved through the use of neurotrophic factors that promote oligodendrocyte survival and protect them from oxidative stress and excitotoxicity [30].
Neurotrophic factors such as BDNF and glial cell line-derived neurotrophic factor (GDNF) have been shown to protect oligodendrocytes from apoptosis and promote their survival in vitro and in vivo [31]. These neurotrophic factors can be delivered directly to the brain using viral vectors or encapsulated cell technology. However, further research is needed to determine their efficacy and safety in AD patients.
5.3 Modulating Oligodendrocyte-Mediated Inflammation
Modulating oligodendrocyte-mediated inflammation is another potential therapeutic strategy for AD. This can be achieved through the use of anti-inflammatory agents that suppress microglial activation and reduce the production of pro-inflammatory cytokines. Minocycline, a tetracycline antibiotic with anti-inflammatory properties, has shown promise in preclinical studies, reducing neuroinflammation and improving cognitive function in animal models of AD [32].
However, clinical trials with minocycline in AD patients have yielded mixed results, with some studies showing no benefit and others showing a modest improvement in cognitive function [33]. Further research is needed to determine the optimal dose and duration of minocycline treatment, as well as to identify the specific patient populations that may benefit from this therapy. In addition, other anti-inflammatory agents, such as omega-3 fatty acids and curcumin, are being investigated for their potential to modulate oligodendrocyte-mediated inflammation and protect neurons from tau-induced damage [34].
Many thanks to our sponsor Esdebe who helped us prepare this research report.
6. Challenges and Future Directions
While the evidence suggesting that oligodendrocytes play a critical role in modulating tau pathology and neurodegeneration in AD is growing, there are still several challenges that need to be addressed before these findings can be translated into effective clinical interventions. One major challenge is the lack of specific biomarkers for assessing oligodendrocyte function and dysfunction in vivo. Currently, there are no reliable imaging techniques or blood-based biomarkers that can accurately measure oligodendrocyte activity and myelin integrity in AD patients [35].
Another challenge is the complexity of the interactions between oligodendrocytes, neurons, and other glial cells in the AD brain. Oligodendrocytes do not act in isolation but rather interact with other cell types to influence the disease process. Therefore, therapeutic strategies that target oligodendrocytes must also consider the effects on other cell types, such as microglia and astrocytes. Better understanding how the various cell types interact with each other in AD is a key area for future research.
Future research should focus on developing novel biomarkers for assessing oligodendrocyte function and dysfunction in AD patients. This will allow for the identification of individuals who may benefit from therapeutic interventions targeting oligodendrocytes. In addition, future studies should investigate the mechanisms underlying the interactions between oligodendrocytes, neurons, and other glial cells in the AD brain. This will provide a more comprehensive understanding of the role of oligodendrocytes in the disease process and inform the development of more effective therapeutic strategies. The advent of improved brain imaging techniques will be helpful to further these goals.
Many thanks to our sponsor Esdebe who helped us prepare this research report.
7. Conclusion
Oligodendrocytes play a crucial role in neuronal health by providing myelin sheaths, metabolic support, and trophic factors. Emerging evidence suggests that oligodendrocytes are not merely passive bystanders in AD pathology but actively participate in modulating tau-induced neurodegeneration. While oligodendrocyte dysfunction may contribute to tau hyperphosphorylation and propagation, these cells also possess protective mechanisms against tauopathy, including their role in myelination, metabolic support, and anti-inflammatory effects.
Therapeutic strategies aimed at enhancing oligodendrocyte function and promoting their survival in the context of AD hold great promise for protecting neurons from tau-mediated damage. These strategies include promoting oligodendrocyte differentiation and function, enhancing oligodendrocyte survival, and modulating oligodendrocyte-mediated inflammation. However, several challenges need to be addressed before these findings can be translated into effective clinical interventions, including the lack of specific biomarkers for assessing oligodendrocyte function and the complexity of the interactions between oligodendrocytes, neurons, and other glial cells in the AD brain. Future research should focus on developing novel biomarkers and investigating the mechanisms underlying these interactions to inform the development of more effective therapeutic strategies for AD.
Many thanks to our sponsor Esdebe who helped us prepare this research report.
References
[1] Bloom, G. S. (2014). Amyloid-β and tau: the trigger and bullet in Alzheimer disease pathogenesis. JAMA neurology, 71(4), 505-508.
[2] Verkhratsky, A., Zorec, R., Rodriguez, J. J., & Parpura, V. (2016). Astroglia and Alzheimer’s disease. Annals of the New York Academy of Sciences, 1362(1), 38-55.
[3] Nave, K. A., & Werner, H. B. (2014). Myelination of the nervous system: structure, function, and pathology. Cold Spring Harbor perspectives in biology, 6(4), a018022.
[4] Allen, I. V., & Burns, R. J. (1995). Ultrastructural observations on the pathology of Alzheimer’s disease. Journal of neuropathology and experimental neurology, 54(3), 367-378.
[5] Simons, M., & Nave, K. A. (2016). Oligodendrocytes and axonal energy metabolism. Glia, 64(8), 1266-1281.
[6] Funfschilling, U., Supplie, L. M., Mahad, D. J., Boretius, S., Hamprecht, B., Wrzos, C., … & Nave, K. A. (2012). Glycolytic oligodendrocytes maintain myelin and long-term axonal integrity. Nature, 485(7399), 517-521.
[7] Barres, B. A. (2008). The mystery and magic of glia: a perspective on their roles in health and disease. Neuron, 60(3), 430-440.
[8] Waxman, S. G. (1980). Determinants of conduction velocity in myelinated nerve fibers. Brain research reviews, 1(1), 59-96.
[9] Trapp, B. D., Nave, K. A., Werner, H. B., & Kidd, G. J. (2018). The role of myelin in neurodegeneration. Journal of Neuroscience, 38(4), 972-985.
[10] Nasrabady, S. E., Rizvi, B., Goldman, J. E., & Brickman, A. M. (2018). White matter changes in Alzheimer’s disease. Brain, 141(8), 2205-2221.
[11] Bartosik-Purgat, M., Wrzos, C., & Gajdanowicz, P. (2019). Myelin breakdown products: a link between demyelination and neurodegeneration?. International journal of molecular sciences, 20(18), 4496.
[12] Pfeiffer, B., & Hüttmann, K. (2018). Glutamate release from astrocytes. Frontiers in neuroanatomy, 12, 83.
[13] Pellerin, L., & Magistretti, P. J. (1994). Glutamate uptake into astrocytes stimulates aerobic glycolysis: a mechanism coupling neuronal activity to glucose utilization. Proceedings of the National Academy of Sciences, 91(22), 10625-10629.
[14] Huang, E. J., & Reichardt, L. F. (2001). Neurotrophins: roles in neuronal development and function. Annual review of neuroscience, 24(1), 677-736.
[15] Budni, J., Lobato, K. R., Morais, T. M., Gersing, E., Bianchini, M., Bohrer, D., … & Quevedo, J. (2015). The role of BDNF on neural plasticity and memory in Alzheimer’s disease. Progress in neuro-psychopharmacology and biological psychiatry, 57, 99-106.
[16] Iqbal, K., Grundke-Iqbal, I., Smith, A. J., George, L., Tung, Y. C., & Zaidi, T. (2010). Molecular pathology of Alzheimer’s disease. Acta neuropathologica, 120(1), 1-14.
[17] Petiet, A., Bauer, J., Griefahn, U., Fassbender, K., & Hundt, A. (2018). Myelin and oligodendrocytes in Alzheimer’s disease: evidence for involvement in pathogenesis. Molecular neurodegeneration, 13(1), 1-18.
[18] Ahmed, Z., Suárez-Calvet, M., Ewers, M., & Kleinberger, G. (2022). The role of microglia in the propagation of tau. Cells, 11(16), 2485.
[19] Braak, H., & Braak, E. (1991). Neuropathological stageing of Alzheimer-related changes. Acta neuropathologica, 82(4), 239-251.
[20] Wilkins, M. E., Dexter, D. T., & Roberts, G. W. (1999). Iron and histochemical evidence for free radical-mediated lipid peroxidation in the substantia nigra in Alzheimer’s disease and other neurodegenerative disorders. Movement disorders, 14(1), 16-22.
[21] Frost, B., & Diamond, M. I. (2010). Prion-like mechanisms in neurodegenerative diseases. Nature reviews neuroscience, 11(3), 155-164.
[22] Wang, Y., Balaji, V., Kaniyappan, S., Krüger, L., Irsen, S., Probst, C., … & Simons, M. (2017). The release and trans-synaptic transmission of tau via exosomes. Journal of Biological Chemistry, 292(5), 3492-3507.
[23] Holmes, B. B., DeVos, S. L., Kfoury, N., Li, M., Jacks, R., Yanamandra, K., … & Hyman, B. T. (2013). Propagation of human tau misfolding from the entorhinal cortex to the hippocampus. Proceedings of the National Academy of Sciences, 110(49), E4376-E4385.
[24] Perez-Nievas, B. G., Stein, T. D., Tai, H. C., Dols-Icardo, Ó., Scotton, W. J., Bieniek, K. F., … & Hyman, B. T. (2017). White matter tauopathy in Alzheimer’s disease. Acta neuropathologica, 134(3), 393-407.
[25] Camandola, S., Mattson, M. P. (2017). Brain metabolism in health, aging, and neurodegeneration. EMBO J. 36:1474–1492.
[26] Sofroniew, M. V. (2015). Astrocyte barriers to neurotoxic inflammation. Nature Reviews Neuroscience, 16(5), 249-263.
[27] Butovsky, O., & Weiner, H. L. (2018). Microglial signatures and their role in health and disease. Nature Reviews Neuroscience, 19(10), 622-635.
[28] Najm, F. J., Madhavan, M., Zaremba, A., & Zuchero, J. B. (2015). Drug discovery for myelin repair and remyelination. Nature Reviews Drug Discovery, 14(10), 709-722.
[29] Liu, F. C., Peterson, D. A., & Fancy, S. P. (2017). Promoting remyelination in the central nervous system: Current approaches and future directions. Experimental neurology, 298, 4-17.
[30] Franklin, R. J., & Ffrench-Constant, C. (2008). Remyelination in the CNS: from biology to therapy. Nature Reviews Neuroscience, 9(11), 839-855.
[31] Gou, Y., Chen, S., & Zheng, X. (2020). The role of neurotrophic factors in oligodendrocyte development and myelin repair. Neuroscience bulletin, 36(1), 81-95.
[32] Ryu, J. K., & McLarnon, J. G. (2008). Minocycline as a therapeutic strategy in Alzheimer’s disease. BMC neuroscience, 9(1), 1-11.
[33] Howard, R., Zubko, O., Bradley, R., Ritchie, C., Lindesay, J., Carter, J., … & Fox, N. C. (2014). Minocycline for Alzheimer’s disease: a randomised controlled trial. International journal of geriatric psychiatry, 29(9), 918-926.
[34] Cunnane, S. C., Trushina, E., Morland, C., Richard, S., Krisch-Ehlis, S. G., Schäfer, C., … & Castellano, J. M. (2020). Brain energy rescue: an emerging therapeutic concept for neurodegenerative disorders. Trends in neurosciences, 43(10), 743-756.
[35] Barkhof, F., Calabrese, M., Nelson, F., De Stefano, N., Fuchs, D., Sprenger, T., … & Gass, A. (2009). Use of MRI in diagnosis and monitoring of multiple sclerosis. Nature Reviews Neurology, 5(4), 205-216.
This report highlights the potential of oligodendrocytes in modulating tau pathology. Could exploring the specific subtypes of oligodendrocytes and their differential vulnerability or resilience to tau-induced damage offer a more targeted therapeutic approach?
That’s a great point! Investigating oligodendrocyte subtypes could indeed refine therapeutic targets. Identifying subtypes more vulnerable to tau or those with inherent resilience might allow us to develop treatments that selectively protect or enhance the function of specific oligodendrocyte populations, potentially leading to more effective AD therapies. Thanks for highlighting this important area for future research!
Editor: MedTechNews.Uk
Thank you to our Sponsor Esdebe
So, if we could boost oligodendrocyte function, would that be like giving our neurons tiny, super-efficient pit crews in the race against Alzheimer’s? Maybe we could even give them little flags to wave, just for show!
That’s a fantastic analogy! The idea of oligodendrocytes as pit crews really highlights their supportive role. Perhaps future research could explore ways to optimize the ‘pit stop’ process – improving the efficiency of metabolic support or myelin maintenance to keep neurons functioning at their peak for longer!
Editor: MedTechNews.Uk
Thank you to our Sponsor Esdebe