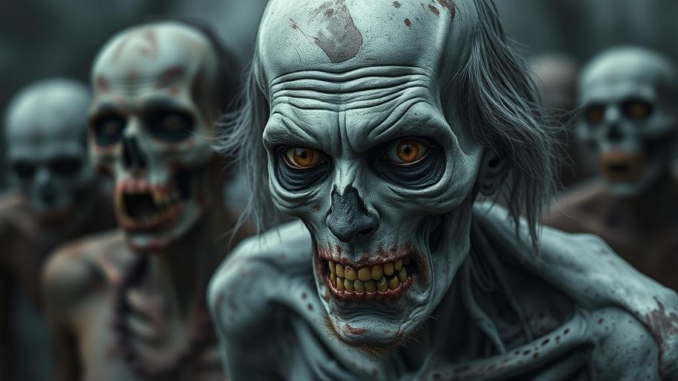
Senescent Cells: A Comprehensive Review of Their Biology, Function, and Therapeutic Implications
Abstract
Cellular senescence, initially recognized as a mechanism to prevent uncontrolled proliferation of damaged cells, is now understood to play a complex and multifaceted role in aging, tissue remodeling, and disease. Senescent cells, often dubbed “zombie cells,” are characterized by a stable cell cycle arrest, altered metabolism, resistance to apoptosis, and the secretion of a complex array of factors known as the senescence-associated secretory phenotype (SASP). This review provides an in-depth biological characterization of senescent cells, encompassing the diverse pathways triggering senescence, the heterogeneity of senescent cell types, factors governing their accumulation, and the intricate composition and effects of the SASP. We further explore the dual nature of senescent cells, highlighting their beneficial roles in processes such as wound healing and tissue repair, alongside their detrimental contributions to age-related pathologies. Finally, we discuss the current landscape of therapeutic interventions targeting senescent cells, including senolytics and senomorphics, and their potential for modulating aging and disease.
1. Introduction
The concept of cellular senescence emerged from the Hayflick limit, describing the finite replicative capacity of normal somatic cells in culture [1]. Originally conceived as a mechanism to prevent the proliferation of cells with DNA damage, senescence has evolved into a broader concept encompassing various forms of cellular stress, including oncogene activation, oxidative stress, telomere shortening, and mitochondrial dysfunction [2]. Senescent cells are no longer viewed solely as inert bystanders but as active participants in tissue microenvironments, profoundly impacting tissue function and organismal health through the secretion of the SASP.
The SASP comprises a diverse cocktail of pro-inflammatory cytokines, chemokines, growth factors, and proteases, creating a paracrine signaling milieu that can influence neighboring cells and the surrounding extracellular matrix. This influence can have both beneficial and detrimental consequences, contributing to tissue repair and wound healing in the short term but also driving chronic inflammation, tissue remodeling, and age-related pathologies in the long term [3]. The accumulation of senescent cells with age is increasingly recognized as a major driver of aging and age-related diseases, making them a compelling therapeutic target. This review aims to provide a comprehensive overview of the biology of senescent cells, highlighting their diverse roles and therapeutic potential.
2. Pathways Leading to Cellular Senescence
Cellular senescence is not a monolithic process but can be triggered by a variety of stimuli, activating distinct but often interconnected signaling pathways. These pathways ultimately converge on the activation of key cell cycle inhibitors and the induction of the SASP.
2.1 DNA Damage Response (DDR)
The DDR is a central pathway in the induction of senescence, activated by various forms of DNA damage, including double-strand breaks (DSBs), single-strand breaks, and oxidative DNA damage. The DDR involves the activation of sensors such as ATM (ataxia-telangiectasia mutated) and ATR (ataxia-telangiectasia and Rad3-related), which phosphorylate downstream targets like p53 and CHK2 (checkpoint kinase 2), leading to cell cycle arrest [4]. Sustained DDR signaling can trigger the stable cell cycle arrest characteristic of senescence, typically mediated by the p53-p21 pathway or the p16INK4a-Rb pathway. The DDR also contributes to the activation of the SASP, often through the activation of NF-κB.
2.2 Telomere Shortening
Telomeres, protective caps at the ends of chromosomes, shorten with each cell division in the absence of telomerase. Critically short telomeres are recognized as DNA damage, activating the DDR and inducing senescence [5]. This pathway is particularly relevant in cells with limited replicative capacity, such as somatic cells. Telomere dysfunction can also occur independently of shortening, for example, due to telomere uncapping or DNA damage at telomeres. Such dysfunction activates the DDR, leading to senescence. The enzyme telomerase can extend telomeres, but its expression is usually suppressed in adult somatic cells. Reactivation of telomerase can prevent telomere shortening and delay or prevent senescence.
2.3 Oncogene Activation
Paradoxically, the activation of oncogenes, such as RAS and BRAF, can induce cellular senescence, termed oncogene-induced senescence (OIS). OIS serves as a tumor suppressor mechanism, preventing the uncontrolled proliferation of cells with oncogenic mutations [6]. The mechanisms underlying OIS involve the activation of the DDR, increased production of reactive oxygen species (ROS), and changes in chromatin structure. OIS is often associated with the activation of the p53 and p16INK4a pathways. However, if OIS is bypassed through inactivation of p53 or p16INK4a, cells can continue to proliferate despite oncogene activation, leading to tumorigenesis. A key point is that the bypass of OIS also leads to cells that are still senescent, just without cell cycle arrest.
2.4 Mitochondrial Dysfunction
Mitochondrial dysfunction, characterized by decreased ATP production, increased ROS generation, and altered mitochondrial dynamics, can trigger cellular senescence [7]. Damaged mitochondria can activate the DDR and induce the SASP. Furthermore, mitochondrial dysfunction can lead to the release of mitochondrial DNA (mtDNA) into the cytoplasm, which can act as a damage-associated molecular pattern (DAMP), activating innate immune responses and promoting inflammation. Mitophagy, the selective removal of damaged mitochondria, is a critical process for maintaining mitochondrial health and preventing senescence. Defects in mitophagy can contribute to the accumulation of damaged mitochondria and the induction of senescence.
2.5 Other Stressors
Various other stressors can induce cellular senescence, including oxidative stress, endoplasmic reticulum (ER) stress, and mechanical stress. Oxidative stress, caused by an imbalance between ROS production and antioxidant defenses, can damage DNA, proteins, and lipids, leading to senescence [8]. ER stress, triggered by the accumulation of misfolded proteins in the ER, can activate the unfolded protein response (UPR) and induce senescence. Mechanical stress, such as excessive stretching or compression, can also induce senescence, particularly in cells in tissues subjected to mechanical forces.
3. Heterogeneity of Senescent Cells
Senescent cells are not a homogenous population but exhibit considerable heterogeneity in their characteristics, depending on the initiating stress, cell type, tissue context, and age of the organism. This heterogeneity is reflected in differences in gene expression, SASP composition, morphology, and functional properties. Understanding this heterogeneity is crucial for developing targeted therapies that selectively eliminate or modulate specific types of senescent cells.
3.1 Stress-Specific Senescence
The initiating stress significantly influences the phenotype of senescent cells. For example, senescent cells induced by DNA damage may exhibit a more pronounced DDR and a distinct SASP profile compared to senescent cells induced by oncogene activation [9]. Telomere-induced senescence may be characterized by telomere dysfunction-induced foci (TIFs) and a specific pattern of gene expression. Stress-specific senescence can also be influenced by the duration and intensity of the stress. Mild or transient stress may induce a reversible form of senescence, whereas severe or chronic stress may induce a more stable and irreversible form.
3.2 Cell Type-Specific Senescence
Different cell types exhibit distinct responses to senescence-inducing stimuli. For example, fibroblasts and epithelial cells may exhibit different SASP profiles and different sensitivities to senolytic drugs [10]. The cell type-specific response to senescence may be influenced by differences in gene expression, signaling pathways, and epigenetic modifications. Furthermore, the functional consequences of senescence may vary depending on the cell type. For example, senescence in immune cells may have different effects on immune function compared to senescence in structural cells.
3.3 Tissue-Specific Senescence
The tissue microenvironment can also influence the phenotype and function of senescent cells. For example, senescent cells in the skin may exhibit a different SASP profile compared to senescent cells in the liver [11]. The tissue-specific response to senescence may be influenced by the presence of different cell types, extracellular matrix components, and signaling molecules. Furthermore, the functional consequences of senescence may vary depending on the tissue. For example, senescence in the lung may contribute to pulmonary fibrosis, whereas senescence in the kidney may contribute to renal dysfunction.
3.4 Age-Related Senescence
The accumulation of senescent cells with age contributes to age-related pathologies. However, the characteristics of senescent cells may change with age. For example, the SASP profile of senescent cells may become more pro-inflammatory with age [12]. The age-related changes in senescent cells may be influenced by changes in gene expression, epigenetic modifications, and the accumulation of cellular damage. Furthermore, the functional consequences of senescence may become more detrimental with age. For example, the contribution of senescent cells to tissue repair may decline with age, whereas their contribution to chronic inflammation may increase.
4. Factors Influencing Senescent Cell Accumulation
The accumulation of senescent cells with age is a critical factor in the pathogenesis of aging and age-related diseases. Several factors influence the rate of senescent cell accumulation, including the rate of senescence induction, the rate of senescent cell clearance, and the replicative history of cells.
4.1 Rate of Senescence Induction
The rate of senescence induction is determined by the exposure of cells to senescence-inducing stimuli, such as DNA damage, oxidative stress, and oncogene activation. The rate of senescence induction may be influenced by genetic factors, environmental factors, and lifestyle factors. For example, individuals with mutations in DNA repair genes may be more susceptible to DNA damage and have a higher rate of senescence induction. Exposure to environmental toxins, such as cigarette smoke and air pollution, can increase oxidative stress and promote senescence. Lifestyle factors, such as diet and exercise, can also influence the rate of senescence induction.
4.2 Rate of Senescent Cell Clearance
The rate of senescent cell clearance is determined by the efficiency of the immune system in recognizing and eliminating senescent cells. The immune system plays a crucial role in clearing senescent cells through mechanisms such as phagocytosis and natural killer (NK) cell-mediated cytotoxicity [13]. The efficiency of the immune system declines with age, leading to a reduced rate of senescent cell clearance and an accumulation of senescent cells. Immunosenescence, the age-related decline in immune function, is a major contributor to the accumulation of senescent cells. Strategies to boost immune function may enhance senescent cell clearance.
4.3 Replicative History of Cells
The replicative history of cells also influences the rate of senescent cell accumulation. Cells with a limited replicative capacity, such as somatic cells, are more prone to senescence due to telomere shortening. The rate of cell division influences the rate of telomere shortening and the likelihood of senescence induction. Cells that divide frequently are more likely to reach the Hayflick limit and undergo senescence. Strategies to slow down cell division may delay senescence.
4.4 Microenvironment Influence
The microenvironment that senescent cells reside in also affects accumulation. As the SASP of senescent cells can induce senescence in surrounding cells, the existence of a senescent cell in tissue may accelerate the creation of more senescent cells. The process is non-linear as the concentration of senescent cells increases.
5. Senescence-Associated Secretory Phenotype (SASP)
The SASP is a complex and dynamic array of secreted factors that mediate the paracrine and systemic effects of senescent cells. The composition of the SASP varies depending on the initiating stress, cell type, tissue context, and age of the organism. The SASP includes pro-inflammatory cytokines, chemokines, growth factors, proteases, and extracellular matrix remodeling enzymes.
5.1 Cytokines and Chemokines
The SASP contains a variety of pro-inflammatory cytokines, such as IL-1α, IL-1β, IL-6, IL-8, and TNF-α, which activate inflammatory signaling pathways in neighboring cells and contribute to chronic inflammation [14]. The SASP also contains chemokines, such as CCL2, CCL3, and CXCL1, which recruit immune cells to the site of senescent cells. The pro-inflammatory cytokines and chemokines in the SASP contribute to the development of age-related diseases, such as atherosclerosis, arthritis, and Alzheimer’s disease.
5.2 Growth Factors
The SASP contains growth factors, such as VEGF, PDGF, and TGF-β, which stimulate cell proliferation, angiogenesis, and extracellular matrix synthesis. These growth factors can promote tissue repair and wound healing in the short term but can also contribute to fibrosis and tumor progression in the long term [15]. The growth factors in the SASP can also stimulate the proliferation of pre-cancerous cells, increasing the risk of cancer development.
5.3 Proteases
The SASP contains proteases, such as MMPs and cathepsins, which degrade the extracellular matrix and promote tissue remodeling. These proteases can contribute to tissue repair and wound healing but can also contribute to matrix degradation and tissue dysfunction in age-related diseases [16]. The proteases in the SASP can also release growth factors and cytokines from the extracellular matrix, further amplifying the inflammatory response.
5.4 Extracellular Matrix Remodeling Enzymes
The SASP contains enzymes that remodel the extracellular matrix, such as lysyl oxidase (LOX) and transglutaminases. These enzymes can cross-link collagen and other extracellular matrix proteins, leading to tissue stiffening and fibrosis [17]. The extracellular matrix remodeling enzymes in the SASP can contribute to the development of age-related diseases, such as pulmonary fibrosis and liver cirrhosis.
5.5 Regulation of the SASP
The SASP is regulated by a variety of signaling pathways, including the NF-κB pathway, the MAPK pathway, and the mTOR pathway. The NF-κB pathway is a major regulator of pro-inflammatory cytokine production in senescent cells [18]. The MAPK pathway regulates the production of growth factors and proteases. The mTOR pathway regulates cell growth and metabolism and can also influence the SASP. Epigenetic modifications, such as DNA methylation and histone acetylation, can also regulate the expression of SASP genes. Post-transcriptional regulation, such as mRNA stability and microRNA-mediated gene silencing, also plays a role in modulating the SASP.
6. Dual Role of Senescent Cells: Harmful and Beneficial Effects
Senescent cells have a dual role, exhibiting both harmful and beneficial effects depending on the context. In the short term, senescent cells can promote tissue repair and wound healing. However, in the long term, the accumulation of senescent cells can contribute to chronic inflammation, tissue remodeling, and age-related diseases.
6.1 Beneficial Effects
Senescent cells play a beneficial role in tissue repair and wound healing by secreting growth factors that stimulate cell proliferation and angiogenesis. Senescent fibroblasts, for example, can promote wound closure by secreting collagen and other extracellular matrix components [19]. Senescent cells also play a role in embryonic development, contributing to tissue remodeling and organogenesis. Senescent cells are also crucial for limiting the expansion of cancerous cells via OIS.
6.2 Harmful Effects
The accumulation of senescent cells contributes to chronic inflammation, tissue remodeling, and age-related diseases. The SASP secreted by senescent cells can induce inflammation in neighboring cells, leading to tissue damage and dysfunction [20]. The SASP can also promote fibrosis and tumor progression. Senescent cells can disrupt tissue homeostasis and contribute to the development of age-related diseases, such as atherosclerosis, arthritis, and Alzheimer’s disease.
7. Therapeutic Interventions Targeting Senescent Cells
The detrimental effects of senescent cells in aging and age-related diseases have led to the development of therapeutic interventions targeting senescent cells. These interventions include senolytics, which selectively eliminate senescent cells, and senomorphics, which modulate the SASP and other functions of senescent cells.
7.1 Senolytics
Senolytics are drugs that selectively kill senescent cells. Several senolytic drugs have been identified, including dasatinib, quercetin, navitoclax, and fisetin [21]. These drugs target different anti-apoptotic pathways that are essential for the survival of senescent cells. Dasatinib, a tyrosine kinase inhibitor, targets tyrosine kinases that are upregulated in senescent cells. Quercetin, a flavonoid, inhibits PI3K and other signaling pathways that promote senescent cell survival. Navitoclax, a BCL-2 inhibitor, targets the BCL-2 family of anti-apoptotic proteins that are overexpressed in senescent cells. Fisetin is a naturally occurring flavonol with senolytic and senomorphic properties.
Preclinical studies have shown that senolytic drugs can extend lifespan, improve healthspan, and alleviate age-related diseases in animal models. Clinical trials are currently underway to evaluate the safety and efficacy of senolytic drugs in humans. The development of new and more selective senolytic drugs is an active area of research.
7.2 Senomorphics
Senomorphics are drugs that modulate the SASP and other functions of senescent cells without killing them. Several senomorphic drugs have been identified, including rapamycin, metformin, and nicotinamide riboside [22]. These drugs target different signaling pathways that regulate the SASP. Rapamycin, an mTOR inhibitor, can reduce the production of pro-inflammatory cytokines by senescent cells. Metformin, an AMPK activator, can improve mitochondrial function and reduce oxidative stress in senescent cells. Nicotinamide riboside, a precursor to NAD+, can improve mitochondrial function and reduce inflammation in senescent cells.
Preclinical studies have shown that senomorphic drugs can improve healthspan and alleviate age-related diseases in animal models. Clinical trials are currently underway to evaluate the safety and efficacy of senomorphic drugs in humans. The development of new and more effective senomorphic drugs is an active area of research.
7.3 Combination Therapies
Combining senolytic and senomorphic drugs may provide a more effective approach to targeting senescent cells. Senolytic drugs can eliminate senescent cells, while senomorphic drugs can modulate the remaining senescent cells and prevent the development of new senescent cells. Combination therapies may also reduce the side effects associated with high doses of individual drugs.
7.4 Challenges and Future Directions
Despite the promising results of preclinical studies, several challenges remain in the development of therapeutic interventions targeting senescent cells. These challenges include the lack of specific biomarkers for senescent cells, the heterogeneity of senescent cells, and the potential for off-target effects of senolytic and senomorphic drugs. Future research should focus on developing more specific biomarkers for senescent cells, identifying the mechanisms that regulate the SASP, and developing more selective and effective senolytic and senomorphic drugs. Furthermore, understanding the long-term effects of senolytic and senomorphic interventions is crucial for ensuring their safety and efficacy. Targeted delivery of senolytic drugs to specific tissues may also improve their safety and efficacy. New technologies, such as CRISPR-Cas9 gene editing, may offer novel approaches to targeting senescent cells.
8. Conclusion
Senescent cells play a complex and multifaceted role in aging, tissue remodeling, and disease. The accumulation of senescent cells with age contributes to chronic inflammation, tissue remodeling, and age-related pathologies. Therapeutic interventions targeting senescent cells, including senolytics and senomorphics, hold great promise for modulating aging and disease. However, further research is needed to address the challenges and optimize the use of these interventions. A deeper understanding of the biology of senescent cells is essential for developing effective strategies to promote healthy aging and prevent age-related diseases. The field is rapidly evolving, and future research will undoubtedly reveal new insights into the role of senescent cells in health and disease.
References
[1] Hayflick, L., & Moorhead, P. S. (1961). The serial cultivation of human diploid cell strains. Experimental Cell Research, 25(3), 585-621.
[2] López-Otín, C., Blasco, M. A., Partridge, L., Serrano, M., & Kroemer, G. (2013). The hallmarks of aging. Cell, 153(6), 1194-1217.
[3] van Deursen, J. M. (2014). The role of senescent cells in ageing. Nature, 509(7501), 439-446.
[4] d’Adda di Fagagna, F. (2008). Living on the edge: telomeres and DNA damage. Nature Reviews Cancer, 8(9), 728-739.
[5] Campisi, J., & d’Adda di Fagagna, F. (2007). Cellular senescence: when bad things happen to good cells. Nature Reviews Molecular Cell Biology, 8(9), 729-740.
[6] Collado, M., Gil, J., Sesena, L., García-Cao, M., Van Hengel, J., Marine, J. C., … & Serrano, M. (2005). Tumour biology: senescence in premalignant tumours. Nature, 436(7051), 642-646.
[7] Wiley, C. D., & Campisi, J. (2016). Mitochondria, telomeres, and cellular senescence. Experimental Gerontology, 86, 78-81.
[8] Toussaint, O., Medrano, E. E., Von Zglinicki, T. (2000). Cellular and molecular mechanisms of stress-induced premature senescence (SIPS) of human diploid fibroblasts. Experimental Gerontology, 35(8), 927-945.
[9] Hernandez-Segura, A., Nehme, J., & Demaria, M. (2018). Understanding and targeting cellular senescence in aging and age-related diseases. Nature Reviews Drug Discovery, 17(5), 361-381.
[10] Childs, B. G., Baker, D. J., Wijshake, T., Conover, C. A., Campisi, J., & van Deursen, J. M. (2016). Senescent intimal foam cells are pro-inflammatory and pro-atherogenic. Aging Cell, 15(5), 952-961.
[11] Krizhanovsky, V., Yon, M., Dickins, R. A., Hearn, S., Simon, J., Miething, C., … & Lowe, S. W. (2008). Senescence of activated stellate cells limits liver fibrosis. Cell, 134(4), 657-667.
[12] Freund, A., Orjalo, A. V., Desprez, P. Y., & Campisi, J. (2010). Inflammatory networks during cellular senescence: causes and consequences. Trends in Molecular Medicine, 16(5), 238-246.
[13] Xue, J., Schmidt, C. S., Sander, J., Moore, C., Brown, K. L., Trussoni, C. E., … & Demaria, M. (2022). Senescence-induced senescence drives osteoarthritis progression. JCI Insight, 7(17), e159263.
[14] Coppe, J. P., Desprez, P. Y., Krtolica, A., & Campisi, J. (2010). The senescence-associated secretory phenotype: the dark side of tumor suppression. Annual Review of Pathology: Mechanisms of Disease, 5, 99-118.
[15] van der Pluijm, I., Vlug, E. J., de Jong, M., Dijke, P. D., & Quax, P. H. (2005). TGF-β signaling in cancer progression and metastasis. J Cell Mol Med, 9(2), 343-356.
[16] López-Otin, C., Bond, J. S., & Denhardt, D. T. (2005). Metalloproteinase, a brief multigenic overview. Annals of the New York Academy of Sciences, 732, 475-485.
[17] Lucero, H. A., & Kagan, H. M. (2006). Lysyl oxidase: properties, regulation and biological roles. Enzyme Research, 2006, 87180.
[18] Salminen, A., Kauppinen, A., & Kaarniranta, K. (2012). Emerging role of NF-κB signaling in the induction of senescence-associated secretory phenotype (SASP). Cellular Signalling, 24(10), 1483-1495.
[19] Jun, J. I., & Lau, L. F. (2010). The matricellular protein CCN1 induces fibroblast senescence and restricts fibrosis in wound healing. Nature Cell Biology, 12(11), 1045-1057.
[20] Childs, B. G., Durik, M., Baker, D. J., & van Deursen, J. M. (2015). Cellular senescence in aging and age-related disease: from mechanisms to therapy. Nature Medicine, 21(12), 1424-1435.
[21] Zhu, Y., Tchkonia, T., Pirtskhalava, T., Gower, A. C., Casaclangana, T., Tejera, D., … & Kirkland, J. L. (2015). The Achilles’ heel of senescent cells: from transcriptome to senolytic drugs. Aging Cell, 14(4), 644-658.
[22] Kirkland, J. L., & Tchkonia, T. (2017). Senolytic drugs: from discovery to translation. Journal of Internal Medicine, 282(3), 216-227.
So, zombie cells, huh? Does this mean we need to start stocking up on senolytic weaponry next to the canned goods and bottled water? Asking for a friend… who may or may not be immortal.
That’s a great question! While we aren’t quite at the zombie apocalypse stage, exploring senolytics is definitely gaining traction in research. The exciting thing is the potential to target the SASP, not just eliminate cells. This could have benefits beyond just extending lifespan, impacting age-related diseases. Always good to be prepared though!
Editor: MedTechNews.Uk
Thank you to our Sponsor Esdebe